Introduction
The Mixteca region of Oaxaca is located in the Sierra Madre del Sur (Instituto Nacional de Estadística y Geografía [INEGI], 2001). This region experiences natural resource degradation, mainly soil erosion, triggered by the interaction of geological, topographical and anthropogenic variables (Toledo et al., 2018). Soil erosion observed is severe (Palacio et al., 2016); according to INEGI (2014), 54 % is hydric.
Environmental deterioration in the region has been noted by several authors (Pérez & Anderson, 2013; Pérez et al., 2019; Reyes et al., 2019) as a consequence of population growth, land use change, overexploitation of natural resources and introduction of grazing. This has caused changes in the ecosystems that are attributed to the initial settlements and agricultural techniques (Leigh et al., 2013), together with current migration (Palacio et al., 2016), which reduces the labor force and causes carelessness in activities that are essential to prevent soil erosion.
In a scenario of dry soils, subject to water erosion, low moisture retention and irregular rainfall, the Mixtecs developed an agricultural system of terraces along intermittent streams, called lama-bordo (LBS), which has become important in terms of production to the present day (Bocco et al., 2019; Leigh et al., 2013; Pérez Sánchez, 2019). According to Rivas et al. (2008), the LBS is a traditional system built with a series of stone curbs or compacted earth, perpendicular to the riverbeds, to capture sediment coming from the upper parts to form new croplands. Stone walls can measure 1 to 4 m in height and 10 to 200 m in length (Spores, 1969). These fluvial terrace systems, in addition to managing water flow, slow down the speed, favor runoff infiltration and drain excess moisture (Perez, 2016); for this reason, it is considered an adaptive practice for food production and important for soil use and management (Perez & Anderson, 2013) and moisture retention.
According to the research by Leigh et al. (2013), LBS may date back at least 3 400 to 3 500 years. Currently, Mixtec communities continue to cultivate in the LBS, maintaining their basic function of reducing soil erosion, retaining sediments, and creating farmland (Pérez & Anderson, 2013). The retained sediment consists of eroded material from adjacent slopes, transported in suspension, and detached from the bed and banks of the channel during torrential events, which need to be analyzed at the watershed scale.
The watershed is a hydrological unit that generates runoff as a result of precipitation and is prone to soil erosion degradation induced by anthropogenic activities and extreme natural events; its size and boundary depend on the length of the stream (Chandniha & Kansal, 2017; Sridhar & Ganapuram, 2021). As noted by Sujatha et al. (2015) and Prabhakar et al. (2019), morphometric factors, dependent on the watershed's topography, shape, and drainage network, are descriptive elements of a hydrographic watershed.
Morphometric analysis provides insights into the evolution, characteristics, dynamics, and potential of a watershed (Sujatha et al., 2015), including its spatial distribution (Rajasekhar et al., 2020). It also quantitatively assesses the hydrological unit (Chandniha & Kansal, 2017) and identifies the relationships among its parameters (Sukristiyanti et al., 2017). By employing equations, this analysis scrutinizes the shape, size, and hydrological relationships within the watershed (Parupalli et al., 2019). Additional complementary parameters for watershed studies are the Topographic Wetness Index (TWI), Flow Power Index (FPI), and Sediment Transport Index (STI), contributing to the hydrological analysis of the landscape. According to Bannari et al. (2017) and Ahmad et al. (2019), these parameters are associated with soil erosion, flow accumulation, sediment deposition, and particle detachment onto the channel. The combination of hydrological indices and morphometric parameters allows for the assessment of potential erosion and flash floods (Abu El-Magd et al., 2021).
The objective of this study is to obtain a model to predict the volume of soil captured by the LBS, based on the morphometric and hydrological characteristics of the micro-watersheds, prevailing in the Mixteca Alta of Oaxaca, Mexico.
Materials and Methods
Study area
A total of twenty-seven sites with LBS were studied (Figure 1), distributed in 13 municipalities of the Mixteca Alta region of Oaxaca, between the coordinates 17° 06’ 00” and 17° 48’ 00” N and 97° 06’ 00” and 97° 36’ 00” W (Figure 2). According to Köppen's climate classification, modified by García (2004), the study area has a semi-arid temperate BS1kw and temperate C(w0) and C(w1) climates, all with mean annual temperatures between 12 and 18 °C with rainfall in summer. The soils are Lithosol, Feozem, Regosol, Luvisol and Cambisol (INEGI, 2005).
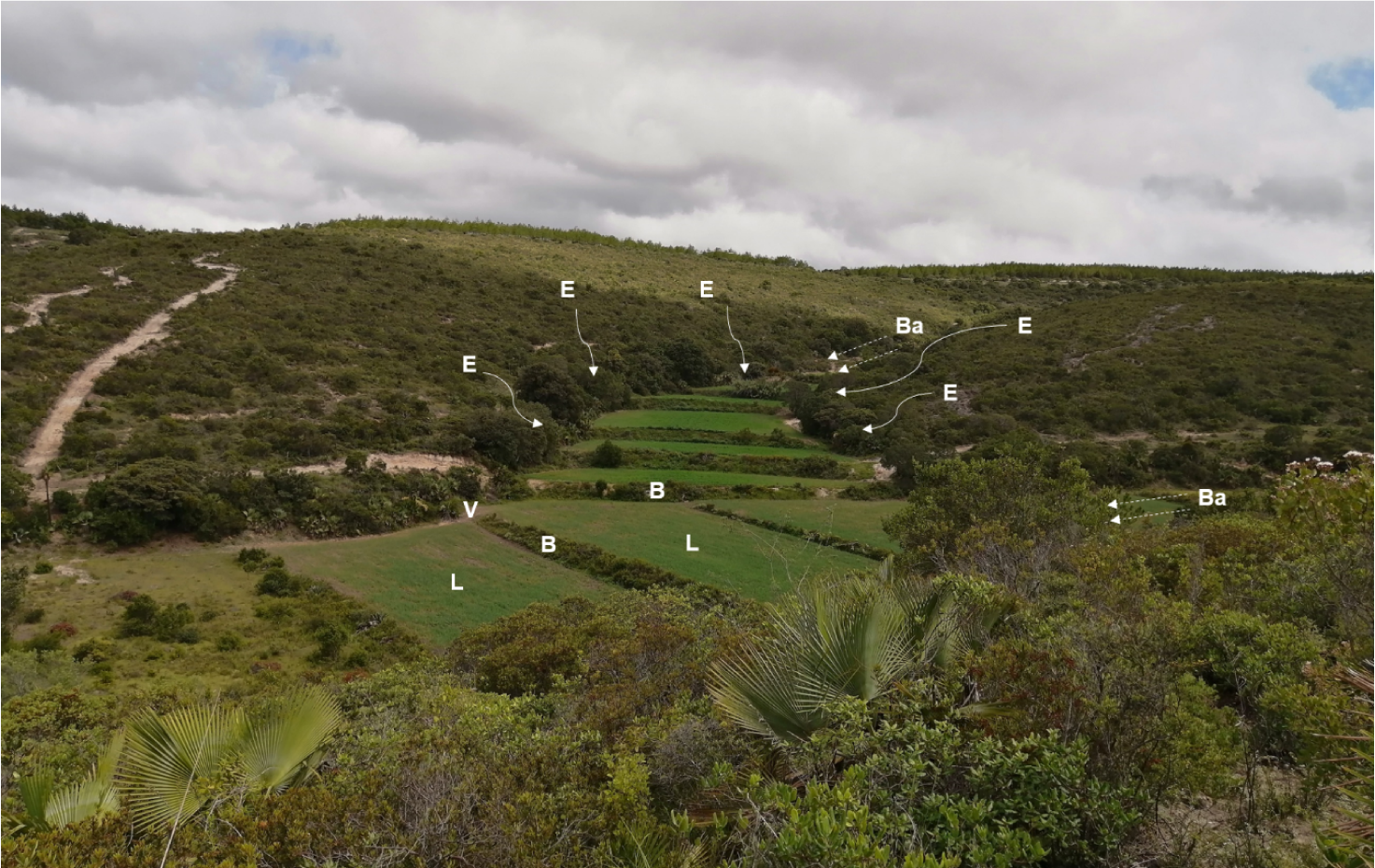
Figure 1 Lama- bordo system in the Mixteca Alta of Oaxaca, México. L = lama, B = bordo, E = lateral runoff, Ba = gullies or flood, V = spillway.
Micro-watershed delimitation and morphometric parameter estimation
The micro-watersheds were delimited with the Continuo de Elevaciones Mexicano 3.0 (INEGI, 2013), the Red Hidrográfica 2.0 (INEGI, 2010) and the automated watershed delimitation module of SWAT (Soil & Water Assessment Tool) model. With this information, the following morphometric parameters were estimated for each micro-watershed: drainage area (A), micro-watershed perimeter (P), maximum micro-watershed elevation (EMa), minimum micro-watershed elevation (EMi), micro-watershed length (Wl), stream order (Oc), main stream length (Le), mean micro-watershed slope (Pc) and mean main stream slope (Pe). These parameters facilitated the calculation of the circularity ratio (Cr), elongation ratio (Er), shape index (Si), time of concentration (Tc) and soil volume (Sv) which are described below.
Circularity ratio
It is the ratio between the area of the watershed (A, km2) and the area of the circle that has the same circumference as the perimeter (P, km) of the watershed (Miller, 1953); its expression is Cr = 4πA / P 2 . This parameter is influenced by the length and frequency of streams, geological structure, land use and cover, climate and slope of the watershed (Balasubramani et al., 2019).
Elongation ratio
The elongation ratio (Er) represents the relationship between the diameter of a circle with the same area as the watershed and the maximum length of the watershed. When the value approaches 1, the shape of the watershed resembles a circle (Schumm, 1956). This parameter serves as an indicator of topography; values close to 1.0 are found in regions with very low relief, while values ranging from 0.6 to 0.8 are generally associated with high relief and steep slopes (Strahler, 1964). The expression is as follows: Er = 2/Le * (A/π) 0.5 ; where A is the area of the watershed (km2), and Le is the length of the main stream (km).
Shape Index
It is the ratio of the width to the length of the watershed squared (Horton, 1932), indicating the flow intensity of a watershed for a defined area. The index should be less than 0.7854, a value corresponding to a perfectly circular watershed (Kandekar et al., 2021). The lower the value, the more elongated the watershed (Singh et al., 2014). The expression of the index is as follows: SI = A / L 2 ; where A is the area of the watershed (km2), and L is the length of the watershed (km).
Time of Concentration
Time of concentration is the duration required for a volume of water to travel from the hydrologically farthest point of a watershed to a downstream reference point (Kaufmann et al., 2017). Several methods are employed for its estimation, and the Kirpich method has been proven to be the most suitable for small watersheds (Rawat & Mishra, 2016). The formula for concentration time is expressed as:
Hydrological Parameters
The specific catchment area and hydrological indexes were estimated using the ‘WhiteboxTools’ included the QGIS program version 3.20.2 (QGIS, 2022), requiring the slope in degrees (β) for the entire watershed. This is distinct from Pe, which refers to the average slope of the main stream. The values reported for each site are weighted averages.
Topographic Wetness Index
This index was proposed by Beven and Kirkby (1979) to quantify the tendency of water distribution in the soil affected by topography (Raduła et al., 2018). The index is determined by the following expression: TWI = Ln (A s / tan β); where A s is the specific catchment area, and β is the slope in degrees.
Stream Power Index
This index is directly proportional to stream power, measures the erosive power of surface flow (Moore et al., 1993) and can be used to identify locations where soil conservation measures need to be implemented (Moore et al., 1991). The parameter is estimated with the following expression:
Sediment Transport Index
This index predicts erosion at a specific point on the landscape (Moore et al., 1991); moreover, it is a fundamental component in the Universal Soil Loss Equation (Bannari et al., 2017). The expression of the index is as follows:
Soil Volume
This parameter allows us to know the approximate amount of soil accumulated in the LBS, with the following expression: Sv = A L * H m ; where A L is the area of the LBS and H m is the average height of the LBS. It should be clarified that in the present study an estimated value of Sv was used as observed data, due to the complexity of obtaining real data in channels filled with sediment that hide the variations in hydraulic sections and changes in slope.
Statistical Analysis
The data were analyzed using RStudio version 1.4.1717 (R Core Team, 2021) to estimate basic statistics (minimum, maximum, mean, standard deviation, and coefficient of variation). Multiple linear regression models were employed to derive the final model explaining the parameter Sv. The model's variables were selected using the backward stepwise method, where initially all calculated parameters were considered and gradually eliminated one by one. The elimination criterion was based on the parameter contributing the smallest amount to R2. The procedure stopped when the reduction in R2 was statistically significant with the remaining predictors (Ruengvirayudh & Brooks, 2016).
To validate the results, the assumptions of the final multiple linear regression model were assessed using Shapiro-Wilks test for normality, Breusch-Pagan test for heteroscedasticity, and variance inflation factor test for multicollinearity.
Results and Discussion
Morphometric Characterization of the Lama-Bordo System
The morphometric characterization data of the LBS are presented in Tables 1 and 2. The behavior of the parameters in the system is described below.
Table 1 Basic morphometric parameters of the micro-watersheds of the lama-bordo systems in the Mixteca Alta region of Oaxaca.
Sites | Area (km2) |
Perimeter (km) |
Mae (m) |
Mie (m) |
Wl (km) | Le (km) |
Pc (%) |
Pe (%) |
---|---|---|---|---|---|---|---|---|
1 | 4.04 | 12.52 | 2 568.00 | 2 153.00 | 4.03 | 4.11 | 17.52 | 7.56 |
2 | 1.65 | 8.27 | 2 313.00 | 2 103.00 | 2.66 | 2.58 | 18.89 | 9.46 |
3 | 0.72 | 4.65 | 2 326.00 | 2 152.00 | 1.38 | 1.29 | 23.81 | 14.39 |
4 | 2.33 | 10.87 | 2 444.00 | 2 131.00 | 3.36 | 4.31 | 27.55 | 18.56 |
5 | 0.36 | 3.95 | 2 341.00 | 2 208.00 | 1.04 | 1.04 | 18.55 | 14.20 |
6 | 0.84 | 5.00 | 2 255.00 | 2 143.00 | 1.52 | 1.08 | 10.00 | 5.79 |
7 | 1.69 | 7.54 | 2 221.00 | 2 075.00 | 2.13 | 2.01 | 13.93 | 7.92 |
8 | 2.19 | 9.93 | 2 307.00 | 2 085.00 | 2.94 | 2.93 | 25.06 | 11.82 |
9 | 2.06 | 7.99 | 2 731.00 | 2 391.00 | 2.34 | 2.18 | 21.47 | 15.85 |
10 | 0.13 | 1.88 | 2 223.00 | 2 155.00 | 0.56 | 0.48 | 18.33 | 18.55 |
11 | 2.52 | 8.93 | 2 710.00 | 2 270.00 | 2.75 | 3.16 | 48.52 | 21.33 |
12 | 1.38 | 6.98 | 2 103.00 | 2 404.00 | 1.88 | 1.67 | 20.58 | 15.62 |
13 | 0.38 | 3.52 | 2 198.00 | 2 333.00 | 0.88 | 0.79 | 16.49 | 9.26 |
14 | 0.88 | 5.65 | 2 167.00 | 2 396.00 | 1.75 | 1.76 | 29.97 | 18.94 |
15 | 0.71 | 5.04 | 2 166.00 | 2 383.00 | 1.50 | 1.36 | 29.29 | 23.88 |
16 | 1.06 | 6.28 | 2 394.00 | 2 627.00 | 1.87 | 1.80 | 18.08 | 16.83 |
17 | 0.68 | 4.74 | 2 435.00 | 2 534.00 | 1.31 | 1.27 | 18.13 | 10.54 |
18 | 2.19 | 9.06 | 2 319.00 | 2 127.00 | 2.24 | 2.35 | 19.41 | 10.56 |
19 | 0.88 | 5.59 | 2 280.00 | 2 128.00 | 1.78 | 1.69 | 17.32 | 9.64 |
20 | 1.88 | 8.20 | 2 345.00 | 2 152.00 | 2.26 | 2.29 | 27.43 | 13.45 |
21 | 2.77 | 10.75 | 2 307.00 | 2 053.00 | 3.74 | 3.23 | 17.40 | 9.96 |
22 | 7.86 | 20.57 | 2 447.00 | 2 119.00 | 6.42 | 7.02 | 24.07 | 8.88 |
23 | 2.40 | 10.08 | 2 871.00 | 2 501.00 | 3.35 | 3.33 | 18.83 | 19.91 |
24 | 0.56 | 4.86 | 2 404.00 | 2 064.00 | 1.44 | 1.43 | 31.92 | 18.76 |
25 | 0.89 | 5.34 | 2 342.00 | 2 150.00 | 1.52 | 1.46 | 26.39 | 16.23 |
26 | 0.27 | 3.85 | 2 307.00 | 2 156.00 | 1.23 | 1.20 | 17.39 | 13.23 |
27 | 0.65 | 4.43 | 2 195.00 | 2 386.00 | 1.28 | 1.10 | 27.39 | 17.25 |
Minimum | 0.13 | 1.88 | 2 103.00 | 2 053.00 | 0.56 | 0.48 | 10.00 | 5.79 |
Maximum | 7.86 | 20.57 | 2 871.00 | 2 627.00 | 6.42 | 7.02 | 48.52 | 23.88 |
Average | 1.63 | 7.28 | 2 359.96 | 2 236.26 | 2.19 | 2.18 | 22.36 | 14.01 |
±SD | 1.56 | 3.75 | 181.04 | 160.88 | 1.22 | 1.38 | 7.46 | 4.74 |
CV (%) | 95.82 | 51.58 | 7.67 | 7.19 | 55.86 | 63.26 | 33.38 | 33.84 |
Mae: maximum elevation, Mie: minimum elevation, Wl: micro-watershed length, Le: main stream length, Pc: average slope of watershed, Pe: slope of the main stream. ±SD: standard deviation of the mean, CV: coefficient of variation.
Table 2 Morphometric and Hydrological Parameters of Micro-watersheds in Lama-Bordo Systems in the Mixteca Alta Region of Oaxaca.
Sites | Si (adim) |
Cr (adim) |
Er (adim) |
Tc (min) |
TWI (adim) |
FPI (adim) |
STI (adim) |
Sv (m3) |
---|---|---|---|---|---|---|---|---|
1 | 0.25 | 0.32 | 0.55 | 28.79 | 9.06 | 673.85 | 8.08 | 975 871.95 |
2 | 0.23 | 0.30 | 0.56 | 24.27 | 8.66 | 101.98 | 5.20 | 525 326.59 |
3 | 0.38 | 0.42 | 0.74 | 11.68 | 9.07 | 484.90 | 9.35 | 191 671.88 |
4 | 0.21 | 0.25 | 0.40 | 34.43 | 8.17 | 66.69 | 3.96 | 434 514.41 |
5 | 0.33 | 0.29 | 0.65 | 10.26 | 9.28 | 328.45 | 13.03 | 97 483.20 |
6 | 0.36 | 0.42 | 0.96 | 13.32 | 8.79 | 31.43 | 1.70 | 318 530.33 |
7 | 0.37 | 0.37 | 0.73 | 20.87 | 8.46 | 69.68 | 3.94 | 728 631.59 |
8 | 0.25 | 0.28 | 0.57 | 27.05 | 8.60 | 182.38 | 8.07 | 890 980.00 |
9 | 0.38 | 0.41 | 0.74 | 15.86 | 9.82 | 474.49 | 11.44 | 153 574.83 |
10 | 0.41 | 0.45 | 0.84 | 4.83 | 7.89 | 102.21 | 10.29 | 180 702.67 |
11 | 0.33 | 0.40 | 0.57 | 22.43 | 7.87 | 34.19 | 4.15 | 172 703.00 |
12 | 0.39 | 0.35 | 0.79 | 13.22 | 8.60 | 365.57 | 14.26 | 459 882.22 |
13 | 0.49 | 0.39 | 0.89 | 8.62 | 9.98 | 329.52 | 10.13 | 63 407.65 |
14 | 0.29 | 0.34 | 0.60 | 13.98 | 8.87 | 702.88 | 15.87 | 131 128.83 |
15 | 0.32 | 0.35 | 0.70 | 11.18 | 8.63 | 738.00 | 14.92 | 85 271.13 |
16 | 0.30 | 0.34 | 0.65 | 15.80 | 7.64 | 82.65 | 6.02 | 119 605.14 |
17 | 0.40 | 0.38 | 0.73 | 13.18 | 8.22 | 45.35 | 4.07 | 135 907.69 |
18 | 0.44 | 0.34 | 0.71 | 21.83 | 9.04 | 164.96 | 5.98 | 516 080.63 |
19 | 0.28 | 0.36 | 0.63 | 15.88 | 8.57 | 85.80 | 6.07 | 290 605.02 |
20 | 0.37 | 0.35 | 0.67 | 21.66 | 7.95 | 22.37 | 1.71 | 154 622.00 |
21 | 0.20 | 0.30 | 0.58 | 29.07 | 8.36 | 57.22 | 3.03 | 458 929.57 |
22 | 0.19 | 0.23 | 0.45 | 61.05 | 8.04 | 28.28 | 2.37 | 1 835 990.94 |
23 | 0.21 | 0.30 | 0.52 | 23.94 | 9.87 | 1057.65 | 21.14 | 831 329.88 |
24 | 0.27 | 0.30 | 0.59 | 11.76 | 9.74 | 926.29 | 21.45 | 108 758.06 |
25 | 0.39 | 0.39 | 0.73 | 12.89 | 8.13 | 92.05 | 6.62 | 156 126.97 |
26 | 0.18 | 0.23 | 0.49 | 10.95 | 7.94 | 71.21 | 6.92 | 494 686.30 |
27 | 0.40 | 0.42 | 0.83 | 9.29 | 8.72 | 315.45 | 10.63 | 86 249.33 |
Minimum | 0.18 | 0.23 | 0.40 | 4.83 | 7.64 | 22.37 | 1.70 | 63 407.65 |
Maximum | 0.49 | 0.45 | 0.96 | 61.05 | 9.98 | 1057.65 | 21.45 | 1 835 990.94 |
Average | 0.32 | 0.34 | 0.66 | 18.82 | 8.67 | 282.80 | 8.53 | 392 539.70 |
±SD | 0.08 | 0.06 | 0.13 | 11.19 | 0.66 | 300.46 | 5.45 | 393 390.22 |
CV (%) | 26.52 | 17.38 | 20.25 | 59.45 | 7.56 | 106.24 | 63.86 | 100.22 |
Si: Shape Index, Cr: Circularity Ratio, Er: Elongation Ratio, Tc: Time of Concentration, TWI: Topographic Wetness Index, FPI: Flow Power Index, STI: Sediment Transport Index, Sv: Soil Volume. ±SD: standard deviation of the mean, CV: coefficient of variation.
Area
The area of the watersheds where the LBS is located ranges from less than 7.86 km2 to more than 0.13 km2, classifying them as micro-watersheds, as indicated by Campos (1992). The perimeter exceeds 1.88 km. Shri et al. (2015) state that the size of watersheds determines the speed of rainfall reaching the main stream, with smaller watersheds experiencing faster runoff compared to larger ones.
Altitude and Average Slope
The analyzed micro-watersheds range in altitude from 2 053 to 2 871 m. The average slopes vary between 10.00 and 48.52 %, and their main streams exhibit slopes between 5.79 and 23.88 %. These parameters favor sudden flooding events, leading to high erosion in mountainous areas and significant sediment accumulation in low-lying areas (Abu El-Magd et al., 2021). These factors have contributed to the formation of the Lama-Bordo Systems (LBS) in the region.
Stream Order
According to Strahler's classification (1964), the streams in 14 micro-watersheds are of the first order, while in 13 micro-watersheds, they are of the second order. This suggests a higher susceptibility to water erosion (Méndez-Gutiérrez et al., 2021).
Main Stream Length
The main stream length varies from 0.48 to 7.02 km. Shri et al. (2015) suggest that relatively shorter lengths are characteristic of areas with steeper slopes and fine soil textures, aligning with the rugged terrain and soil conditions where the Lama-Bordo Systems (LBS) are located.
Shape Index
The shape index in the LBS ranged from 0.17 to 0.44, with an average of 0.29, suggesting an elongated shape of the micro-watersheds experiencing low maximum flows with longer duration (Singh et al., 2014). This indicates low intensity (Kumar & Chaudhary, 2016; Kusre, 2016) and the ability to manage floods (López & Fernández, 2021).
Circularity Ratio
The values ranged from 0.23 to 0.45, with an average of 0.33, indicating an elongated shape (<0.5), low runoff discharge, and permeable subsurface conditions, according to Singh et al. (2014) and Malik et al. (2019).
Elongation Ratio
Based on the classification proposed by Schumm (1956), 4 % of the sites have a circular shape (>0.9), 11 % oval shape (0.8-0.9), 30 % less elongated shape (0.7-0.8), 44 % elongated shape (0.5-0.7) and 11 % very elongated shape (<0.5). In general, most of the micro-watersheds where LBS are found have elongated shape. Soni (2017) points out that areas with high Er values have high infiltration capacity and low runoff, which favors moisture storage in LBS.
Hydrological Characterization of Lama-Bordo Systems
The hydrological characterization data of the LBS are presented in Table 2. The following describes how the parameters behave within the system.
Topographic Wetness Index
Values ranged between 7.87 and 10.06, indicating areas more susceptible to flooding based on the assessment of local relief (Pourali et al., 2014). Higher values are associated to drainage lines (Różycka et al., 2017), where the LBS are situated due to a gentler slope, facilitating moisture accumulation. On the other hand, it identifies areas prone to landslides (Różycka et al., 2017) contributing sediments to the system.
Flow Power Index
The values ranged from 22.37 to 1 057.65, indicating particle detachment due to accumulation and flow velocity effects (Bannari et al., 2017), where the energy of runoff water moves the soil's surface layer. Low values suggest potential sediment deposition and are characteristic of the lower parts of watersheds and the main channel, while high values indicate a higher risk of erosion (Abu El-Magd et al., 2021). For the LBS, higher values were found on their sides (runoff areas) with steeper slopes, contributing to increased acceleration in the currents that transport sediments and water to the terraces.
Sediment Transport Index
The values ranged from 1.70 to 21.45, indicating the capacity for sediment transport, accumulation, and their spatial distribution (Ahmad et al., 2019). The highest values observed in the LBS were situated on their sides (lateral runoff), while the lower values were found in the lamas or terraces. This agrees with the findings of Bannari et al. (2017), who suggested that lower areas are prone to flooding and serve as deposition points for transported particles; low values reflect slow mobility and sediment accumulation. The system as a whole is not significantly affected by this index, but the lateral areas, due to their steep slopes, are highly susceptible. Similar to the Flow Power Index (FPI), slope gradient is a critical factor influencing these areas.
Although morphometric analysis allows the prioritization and location of the places where it is necessary to implement soil and water conservation structures, in the micro-watersheds under study there are already LBS that have contributed to soil and moisture retention for agricultural purposes and where local materials are used for their construction. On the other hand, the hydrological indexes allow the detection of areas of moisture accumulation and those most prone to erosion that will contribute to the system over time.
Calibration and Validation of the Model for Soil Volume Estimation
The model, constructed through backward stepwise regression, initially involved 11 variables from the first 17 sites (Tables 1 and 2). It was then reduced to parameters A, Pe, TWI, and STI, which, according to the analysis, are directly related to the captured sediment volume. The importance of this model lies in demonstrating the potential of LBS in storing soil particles and explores its impact on the soil erosion problem.
Table 3 shows the regression analysis presenting coefficients for each parameter, all of which are statistically significant (P < 0.01). These coefficients facilitated the development of the predictive model to estimate Sv in the LBS of the region of Mixteca Alta, Oaxaca:
Table 3 Multiple linear regression analysis of morphometric parameters and hydrological indexes of lama-bordo systems in the Mixteca Alta region of Oaxaca.
Parameters | Coefficients | Standard error | t | p>|t| |
---|---|---|---|---|
Intercept | 2 716 855 | 698 801 | 3.888 | 0.00216** |
Area | 257 920 | 35 727 | 7.219 | 1.06E-05*** |
Pe | -98 429 | 19 532 | -5.039 | 0.00029*** |
TWI | -266 849 | 77 505 | -3.443 | 0.00487** |
STI | 43 621 | 13 054 | 3.342 | 0.00587** |
Pe = main stream slope, TWI = topographic wetness index, STI = sediment transport index. R2 = 0.8502, P = 6.894E-05.
Using the parameter values of the micro-watershed area (A), main stream slope (Pe), topographic Wetness Index (TWI), and sediment transport index (STI), 85 % of the observed variability in accumulated sediment volume is explained (R2 = 0.8502), with a P-value of 6.894E-05. Therefore, the estimation of sediment volume in the LBS is considered reliable.
To test the above results, the model was validated with data from 10 independent sites (18-27 from Tables 1 and 2). The simple linear regression analysis of the observed and predicted cumulative sediment volume can be seen in Table 4, with a P value = 5.32E-05 proving to be statistically significant.
Table 4 Simple linear regression analysis for validation of sediment volume estimation model (R2 = 0.8833) in the lama-bordo systems.
Parameters | Coefficient | Standard error | t | p>|t| |
---|---|---|---|---|
Intercept | 4 973.946 | 99 762.395 | 0.050 | 0.961 |
Measured volume | 1.105 | 0.142 | 7.782 | 5.32E-05*** |
Figure 3 shows the linear regression with R2 = 0.883, indicating a good fit of the model and demonstrating certainty in its subsequent use for sediment volume estimation in the LBS.
The parameters included in the predictive model (A, Pe, TWI, and STI) turned out to be a combination of variables that allowed the physical and hydrological characterization of LBS, because they are formed in micro-watersheds with high slopes and are established in the lower parts, where sediment deposition is favored. The slope has a greater impact on sediment accumulation in LBS as it influences Pe, TWI, and STI. This is because it is one of the factors determining geomorphic activity (Guerra et al., 2017), closely related to soil erosion processes (Manyevere et al., 2016).
The involvement of STI and TWI in the model is interesting. The former has a positive effect, demonstrating spatial sediment transport capacity (Bannari et al., 2017), with higher values on the sides of the LBS, indicating soil particle contribution to the systems. In the case of TWI, its relationship is negative with sediment accumulation; higher TWI values are within the LBS, where there is less slope, preventing sediment loss and promoting moisture accumulation.
This is where morphometric analysis provides an understanding of the drainage watershed hydrology, and hydrological indexes help identify areas prone to flash floods and soil erosion. This combined approach provides a tool for comprehending and determining sediment accumulation, erosion, and the zoning of flash floods, as mentioned by Abu El-Magd et al. (2021).
A combination of parameters supports the formation of the sites where LBS are located. Additionally, local farmers construct and maintain the containing walls using family labor and locally sourced materials, keeping costs low and making it accessible. These systems are implemented on a small scale; at larger scales, it would be necessary to consider different materials, labor, and machinery.
The LBS have played a crucial role in maintaining the system in balance for many years by retaining sediments, they prevent their loss and facilitate deposition in the lower parts of micro-watersheds. This alteration in the landscape proves beneficial for land-use changes, particularly in integrating agricultural components and ensuring self-sufficiency in food production.
Conclusions
Morphometric parameters and hydrological indexes were employed to characterize the conditions that facilitate the development of lama-bordo systems. These systems hold significant importance in the Mixteca region of Oaxaca, Mexico, where they are established within natural watercourses for erosion control, sediment retention, and moisture storage, conditions crucial for the local agriculture's development. The obtained model provides a reliable estimate of sediment volume storage using geographic information systems and equations incorporating morphometric parameters (micro-watershed area and main channel slope) and hydrological indexes (topographic wetness index and sediment transport index). The model accounted for 85 % of the observed variability, indicating that the estimation of sediment volume accumulated in lama-bordo systems is reliable and potentially applicable to sites with similar characteristics to those in this study.