INTRODUCTION
The western North American Cordillera hosts important goldbearing quartz veins, silver-bearing quartz veins, volcanogenic massive sulfide (VMS) deposits and Zn-Cu-Pb-Ag skarns systems including the silver belt of central Mexico, the Mother Lode of southern California, and counterparts in British Columbia and southeastern Alaska, to the Klondike district in central Yukon. All these veins, VMS and skarns systems are structurally controlled by major fault zones or weakness zones, which are often reactivated terrane-bounding sutures that formed in orogens built during accretion and subduction of terranes along the continental margin of North America, and in México along the faults (Figure 1) related to the opening of the Gulf of México, (de Cserna, 1976a, Pindell, 1985, Vélez, 1990, Vassallo, 2003, Vassallo et al., 2004, Piercey, 2011, Pindell et al., 2012). Mineralization ages span mid-Jurassic to early Tertiary (Vassallo et al., 2000, 2004). The VMS are related with high-temperature magmatism within well-defined thermal corridors, as those described by Piercey (2011), associated with extensional geodynamic settings in volcanogenic environments, Sillitoe, (1982). VMS deposits are determined by tectonic regime change, evolution of the continental lithosphere composition, and irreversible transformations occurring in the Earth ́s atmosphere, hydrosphere, and biosphere, Dergachev and Eremin, (2008).

Figure 1 Ore deposits of Central Mexico. With Jurassic-Cretaceous faults modified after De Cserna (1976a), Vélez (1990), and others.
Hydrothermal alteration assemblages of VMS have been investigated from mineralogical, textural, petrophysical, and chemical perspectives (e.g., Starostin, 1979, 1984; Franklin et al., 1981; Starostin and Dergachev, 1982; Lesher et al., 1986; Eastoe et al., 1987; Morton and Franklin, 1987; Gemmell and Large, 1992; Large, 1992; Huston, 1993; Khin et al., 1996; Paulick et al., 2001; Schardt et al., 2001; Solomon and Gaspar, 2001; Starostin et al., 2002). Much of this research has contributed to the knowledge of composition, mineralogical and chemical zonation of footwall alteration systems below massive sulfide systems, which are the sites of intense fluid-rock interaction in the upflow or vent zone below the sea floor. Due to metamorphism, tectonic deformation, and lack of access to alteration pipes, few studies have focused on stringer zones of VMS and their alteration assemblages (Lydon, 1988).
San Nicolás is a Late Jurassic-Early Cretaceous, (Danielson, 2000, Iriondo et al 2003, Mortensen et al 2008), stratiform, polymetallic massive sulfide deposit hosted within a felsic-basic lava-dominated sequence in southern Zacatecas, Mexico. The deposit has a total mineral resource of 99 Mt with 1.36 % Cu, 1.64 % Zn, 0.41 g/t Au and 24 g/t Ag, (Johnson et al., 2000). The San Nicolás ore consists of variable proportions of pyrite, sphalerite, galena and chalcopyrite, with gangue chlorite, tremolite, calcite, dolomite, barite, magnetite and quartz. Massive and semi-massive sulfides occur in a single horizon at the stratigraphic contact between rhyolite domes in the hangingwall and a volcano-sedimentary sequence dominated by mafic lavas in the footwall. This rhyolite horizon is referred to the "San Nicolás favorable unit" in this paper. Below the San Nicolás deposit there is a texturally, mineralogically and geochemically diverse strata-bound alteration zone that extends across the controlling mineralization fault zone into the footwall at least 200 m down, with gradational contacts to the surrounding unaltered or weakly altered volcanic rocks.
It has been recognized (Smirnov, 1944, 1963, 1968, 1976; Yakovlev, 1959) that footwall alteration zones associated with volcanic massive sulfide (VMS) deposits may have a stratabound geometry or a discordant, pipelike shape. A comparative study of Australian VMS (Large, 1992) and VMS in Russia and Kazakhstan (Yakovlev, 1959; Smirnov, 1976; Smirnov and Gorzhevskii, 1978; Starostin, 1984), showed that most deposits have stratabound and semiconformable footwall alteration zones. Well-defined, pipelike alteration zones, such as those associated with Precambrian deposits of Noranda-Canada, (Sangster, 1972; Barrett and MacLean, 1994; Shriver and MacLean, 1993), Paleozoic deposits of Rudnii Altai (Yakovlev, 1959; Smirnov, 1976; Smirnov and Gorzhevskii, 1978) and Tertiary Kuroko deposits, (Ohmoto and Skinner, 1983; Shikazono et al., 2008), are common worldwide.
The study of stratabound alteration zones is relevant for base metal exploration and for research in rock-water interaction processes. Because of their large extent below the deposit, stratabound alteration zones provide better exploration criteria than the spatially confined alteration pipes. However, in contrast to pipelike alteration zones, stratabound footwall alteration zones typically lack clear boundaries of mineralogical zoning that increases the difficulty for massive sulfide exploration.
Careful examination of geological, petrographical, and geochemical data is required to discriminate the large-scale alteration zones generated by general mineralizing hydrothermal activity from alteration related to syn-volcanic seafloor alteration processes.
This paper provides a regional geological appraisal and a detailed description of alteration observed at San Nicolás ore deposit, including textural, mineralogical and geochemical characteristics. Several geochemical features like CCPI, AI, Na2O, show systematic variations with increased proximity to the massive stratabound sulfide deposits.
REGIONAL GEOLOGY
The San Nicolás deposit is located in a NW-SE-trending belt of deformed Jurassic-Cretaceous marine sedimentary and volcanic rocks defined in this paper as the Chilitos Group in the central part of Mexico (Figure 2). The lower unit of this group was defined first by de Cserna (1976a) as Chilitos Formation. Discontinuous outcrops over a distance of more than 300 km (Zacatecas to Guanajuato) expose a grossly conformable stratigraphic succession, up to 1.5 km thick, with gentle folding and NW 45° axis orientation. The group was intruded by Jurassic to early Tertiary plutons (El Saucito, Peñon Blanco and Comanja type plutons). Mesozoic rocks are for the most part covered by the Tertiary rhyolitic La Bufa Formation and others minor volcanics by semiconsolidated alluvium.
The Chilitos Group was divided into three conformable formations (Vassallo, 2012). At the base is the Arroyo Chilitos Formation, (de Cserna, 1976b), which consists of dominantly basaltic to andesitic sills and lavas, similar to those described at Guanajuato, (Vassallo, 1988a, 1988b; Vassallo et al., 1989, Freydier et al., 1997, 2000, Vassallo and Reyes-Salas, 2007, Aranda-Gómez et al., 2012).
The overlying San Nicolás Formation mainly consists of rhyolitic lavas, synvolcanic intrusions and volcaniclastic rocks. The crystallization age of a rhyolite sample is given by the total range of 206Pb/238U ages for three concordant analyses (146.5±2.2 Ma) dated using U-Pb zircon methods (Mortensen et al., 2008). The upper La Virgen Formation comprises lithic sandstone and graywacke with some basaltic lava (Table 1) with a hornblende age of 117.94 Ma (Iriondo et al., 2003), synvolcanic intrusions, and various volcaniclastic and sedimentary rocks. Geochemical data suggests that the volcanic rocks of the Chilitos Group were emplaced in an active continental margin (Schandl and Gorton, 2002). The Chilitos Group was wide folded and took a tilted position during regional normal faulting in the Aptian. The area probably represents the northeastern part of a large NW-SE trending overthrust structure, and in some places the Chilitos Group overthrusts the Lower Cretaceous limestone of the Proaño Group (Trejo, 2001), as seen in the Town of Fresnillo (de Cserna, 1976b). The regional metamorphic grade increases from sub-greenschist in the eastern part to greenschist in the western part of the Zacatecas state (Vassallo and Solorio-Munguia, 2005).
In early Tertiary, the Saucito and Peñón Blanco plutons were emplaced (Aranda et al., 2007), which postdates the end of Laramide deformation (Aranda et al., 2007; Vassallo et al., 2008). Its porphyritic texture, with beta quartz pseudomorphs and euhedral sanidine phenocrysts suggests a shallow subvolcanic intrusion. The absence of penetrative fabrics associated with folding and/or shearing indicates a post-tectonic emplacement. A40Ar/39Ar age of 49.5 Ma constrains the end of Laramide deformation in this area (Aranda et al., 2007).
Tertiary rhyolitic rocks from 32 to 27 Ma cover the mining district. Samples MC-14, 15, 20, 33 and P5-95 (Table 1) provide several ages for the known volcanic episode at Pinos, Zacatecas, 20 km east of San Nicólas, (Aranda et al., 2007).
A later phase of Miocene extensional faulting is associated with the NE-SW faults structures that formed large grabens and cut the VMS deposits and Eocene clastic units (Figure 3).
GEOLOGICAL SETTING OF THE SAN NICOLÁS ORE DEPOSIT
San Nicolás ore deposit is the largest known VMS deposit in central Mexico. The San Nicolás mining district contains several stratiform, mound or lense-shape orebodies, which extend over an area of more than 500 km2, at the middle of the Chilitos Group. The San Nicolás deposit and its host-rock succession are examined here in 5 drill corebased cross sections through the deposit (Figures 3 and 4).
A model for the paleofacies architecture of the host-rock succession is reconstructed based on structural-volcanic analysis. The San Nicolás deposit formed on a paleochannel or graben produced by Jurassic-Cretaceous growing longevity faults, that act as feeders of marine bimodal-felsic volcanic rocks as Kuroko type deposits of Piercey, (2011), one of them La Panza fault is the principal control of the mineralization (Figures 4, 5). This geologic setting is analogous to the environment of the modern PACMANUS hydrothermal field, Manus Basin, Papua New Guinea, (Binns and Scott, 1993), and others described by Schulz, (2012). Lydon, (2010) also recognized the important role that have the synsedimentary faulting and the growing longevity faults that control the mineralization also in Sedex (VMS variant) deposits at B.C. Canada.
The footwall alteration zone at San Nicolás is laterally continuous along the strike extent of the deposit and extends for at least 200 m into the hosting volcanic rocks. Nevertheless, Larson (1984) found in the footwall andesitic rocks at Bruce VMS deposit, Arizona, that the alteration zone extends for at least 400 m away from the ore lenses.
Contacts with the surrounding unaltered basalts are gradational. Moderately altered rhyolite represents the bulk of the footwall alteration zone and shows diverse textural, mineralogical and geochemical features, which are described below. Minor zones of intense alteration cut across the footwall alteration zone and extend up to the massive sulfide bodies (Figures 4, 5).
The San Nicolás deposit favorable host strata is up to 300 m thick lithologically diverse unit. It includes coarse, "quartz-eye" bearing volcaniclastic rocks, coarse porphyritic "quartz-eye" intrusions and a few rhyolite breccias. This volcanic succession includes massive to semimassive sulfide and massive to semi-massive barite orebodies.
The hanging wall of the normal fault consists of altered to weakly altered rocks, including volcaniclastic rocks and graywackes with some basaltic lavas overlying the San Nicolás Formation of porphyritic rhyolite. Volcaniclastic sedimentary rocks consist mostly of dacitic lithic clasts and some lithoclasts of basaltic lava.
Previous studies of the San Nicolás deposit have proposed that the orebody is syngenetic and massive sulfides were deposited onto the sea floor from dense saline solutions (Johnson et al., 2000), and may have partially formed by sub-seafloor replacement of intensely altered rhyolite rocks. The arrangement of massive pyrite lenses in the San Nicolás unit, the distribution of barite, and variations in metal ratios of the massive sulfides along strike of the deposit indicate that mineralization occurred in 10 hydrothermal district fields (Figure 3). Parts of ore lenses with high Cu ratios (Cu/[Cu + Zn]), low Zn ratios (Zn/[Zn+Pb]) and basal zones of massive pyrite-chalcopyrite are interpreted as discharge sites of hot, metal-rich fluids, whereas massive barite with disseminated sulfides represent peripheral, low-temperature zones of the hydrothermal system (Huston and Large, 1987).
The structural geology of the San Nicolás area was examined in detail by Johnson et al. (2000) and Vassallo (2003). Two generations of faults have been recognized. A NW-trending pattern of faults of Jurassic-Cretaceous age cut the footwall rhyolite rocks into an apparent hanging wall position (Figure 3). NW trending faults structures have been recognized at San Nicolás, for example the so called La Panza Jurassic-Cretaceous growing fault, (Figures 5, and 6) after Johnson et al., (2000). The San Nicolás mining district is cut by a second NE- striking group of normal faults (Miocene Basin and Range structures of Vassallo, 2003; Figure 3).
ALTERATION FACIES AND GEOMETRY OF THE ALTERATION ZONE AT SAN NICOLÁS
Alteration at the San Nicolás deposit is mineralogically and texturally diverse and several alteration facies have been defined here on the basis of dominant mineral assemblages and alteration intensity with variable proportions of quartz, muscovite, chlorite, epidote, tremolite, calcite, K-feldspar, and pyrite, (Figures 6, 7). Alteration facies is a strictly descriptive term as proposed by Riverin and Hodgson (1980), and Vassallo (2003). In this paper alteration facies refers to rocks with modification of pristine characteristic mineralogical, petrographical, chemical and textural features.
There are 6 alteration facies from weak to intense degree (Figure 8):
Weak alteration is patchy or vein controlled and includes two different facies: Weak chlorite-sericite (weak chl-ser) alteration (Figure 7), and chlorite-pyrite-sericite (chl-py-ser) alteration (Figure 7).
Both facies are generally not texturally destructive. These alteration facies are particularly common in hanging-wall volcanic rocks. No clear systematic variations in the abundance or relative proportions of weak alteration facies have been observed with regard to the proximity to the massive sulfides (Vassallo, 2003). Phyllosilicate alteration is particularly prominent in the groundmass of volcaniclastic rocks in the hanging wall block, which likely were rich in fine-grained, glassy volcanic fragments and/or fine grain sedimentary clay minerals. Hence, it is inferred that these alteration facies reflect background alteration processes related to mineralizing hydrothermal circulation.
Moderate to intense alteration is confined to the footwall below the massive sulfides. Representative cross sections through San Nicolás deposit (Figures 5, 7) illustrate the arrangement of alteration facies. The footwall alteration zone consists predominately of:
Feldspar-destructive, muscovite-chlorite-biotite-rich mottled (strong chl-py-ser-K-feldspar) alteration facies (Figure 7) with minor disseminated pyrite (3 vol %). This alteration facies is characterized by substantial variations in the relative proportions of muscovite, chlorite, biotite and quartz, in hand specimen scale, producing textures which superficially resembling those of volcaniclastic rocks. Such fabrics, which are related to one domain and/or multiphase alteration processes, are common in footwall alteration zones below VMS deposits and have frequently led to the misinterpretation of altered felsic lavas as primary pyroclastic rocks (Allen, 1988). Criteria for the discrimination of genuine clastic and apparent clastic textures at San Nicolás are presented in Vassallo and Solorio-Munguía (2005).
The quartz-K-feldspar (intense qtz-py-ser) alteration facies (Figure 7) occurs locally on the fringes of the footwall alteration zone. It has a white, flinty appearance in hand specimen and is composed of microcrystalline quartz and K feldspar with minor sphalerite.Mottled alteration and quartz-K-feldspar alteration facies typically show complex, gradational contacts.
Disseminated tremolite and patchy carbonate-tremolite-chlorite (Ab-calc-ep) alteration facies (Figure 7) exist sporadically in the footwall alteration zone, generally in the top of the rhyolite lava and in association with the tectonic breccias of the San Nicolás sulfide SW lenses (Figures 6, 7). Herrmann and Hill (2001) interpreted this type of alteration facies as representing metamorphosed hydrothermal carbonate-chlorite-quartz alteration facies.
Intense, quartz-pyrite (silica alteration and cpy-py stringers) alteration facies, (Figure 7) develop stratiform bands around the massive sulfides and discordant zones within the footwall, that are interpreted as the main fluid pathways during mineralizing hydrothermal activity. Apart from relict, partly recrystallized quartz phenocrysts, the quartz-pyrite alteration facies retains no primary mineralogy or fabrics. This facies is dominantly siliceous with 5 to 20 % (modal) pyrite in disseminated grains and irregular veins. The total phyllosilicate content is <20 % and proportions of muscovite, chlorite and biotite are variable (Figure 8).

Figure 8a Textures of volcanics at the San Nicolás deposit. Altered mafic volcanic rocks. a) BSN-05depth 526 m (MVT), py, sericite, calcite, chlorite; b) BSN-6depth 501 m (MVT), py, sericite, calcite, chlorite; c) BSN-75 adepth 414 m (MVT), py, sericite, chlorite; d) BSN75 bdepth 414 m (MVT), py, sericite, chlorite. BSN-10depth 412 m (MVT), py, chlorite, sericite; f) BSN-11depth 217 m (MVS), py, sericite, qtz, chlorite. Textures of volcanics at the San Nicolás deposit; g) BSN-12depth 188 m (MVS), plagioclase, sericite, chlorite; h) BSN-13depth 164 m (MVS), py, sericite, calcite, chlorite; i) BSN-14 depth 145 m Mafic flows (XLT), py, calcite, sericite, chlorite; j) BSN-15depth 125 m Mafic flows, volcaniclastic sediments and tuffs (TUF), Basalt with low alteration, qtz, calcite, k) B01superficial sample, greywackes (TUF) with: py, chlorite, sericite; l) B02 superficial sample, sandstone (TUF) with: py, sericite, qtz, chlorite.
GEOCHEMISTRY OF ALTERATION FACIES
This section examines the major and trace element compositions of the variably altered volcanics for San Nicolás deposit. Representative analyses of least altered footwall rhyolite, quartz-eye porphyry, hanging wall dacite and the principal alteration facies are presented in Table A1 (in the electronic supplement).
Methods
Whole-rock chemical analyses were obtained from 100 samples of altered footwall volcanics and hanging wall rhyolites (Table A1). Crushed samples of drill core (quarter core splits, 10 cm long) were pulverized in a tungsten carbide mill. Major elements were measured by X-ray fluorescence spectrometry (XRF) on fused discs and pressed powder pellets at the ACME Laboratory in Vancouver, Canada. Concentrations of the trace elements (Ag, Bi, Cd, Sb, Cs, Tl, and U) were determined by ICP-MS, (0.5 gm sample leached with 3 ml 2-2-2 HCl-HNO3-H2O at 95 °C for one hour, diluted to 10 ml), REE by LiBO2 fusion, ICP/MS finished, at the same laboratory.
Additional data were compiled from previous studies. These included analyses of carbonate-tremolite-chlorite alteration facies from Johnson et al. (2000) and quartz-eye porphyry (Mortensen et al., 2008). These analyses were performed at the ACME Laboratory using a combination of ICP-OES for major elements, XRF on pressed powder pellets for Zr and Ba, and atomic absorption spectroscopy (AAS) for Cu, Pb and Zn. Furthermore, supplementary analyses of altered volcanic rocks in diamond drill holes (DDH) SN-25, SN-35-37 and SN-40-41, (Table A1) were analyzed by ICP-OES for major elements, ICP-MS and INAA for trace elements, XRF on pressed powder pellets for Zr, AAS for Cu, Pb, and Zn, and Leco (infrared spectrophotometry) for S.
Major element geochemistry
The altered mafic rocks show large variations in the concentrations of Na, Ca, Mg and Fe (Figure 7, Table A1, DDH SN-25, samples BSN-28-31). Least altered mafic rocks are within Mg and Fe average and contain between 3 and 8 wt % Na2O+CaO, which is within the range typical for fresh modern mafic volcanics. Increasing alteration is coupled with a gradual decrease in Na2O and CaO, culminating in intensely altered basalts having Na2O+CaO values < 1.04 wt % (sample BSN-45). This trend is indicative of plagioclase destruction and formation of muscovite (sericitization). Intense pyritic alteration facies is characterized by high MgO+FeO values, commonly between 3 and 20 wt % (up to 36 wt %, sample BSN-24), due to the abundance of pyrite and chlorite. The calcareous alteration facies show extreme CaO and MgO enrichment (samples BSN-11-12, BSN-76-81, above the ore body), which is related to a combination of carbonate (dolomite) and chlorite (Figures 9, 10). Samples of quartz-eye porphry have MgO+Fe2O3 values mainly between 6 and 10 wt % and their Na2O+CaO content ranges between <1 and 4 wt %, (BSN-05-43) overlapping with least altered, weakly altered, and moderately altered footwall rhyolite.

Figure 9 The alteration and variations in the concentrations of Na, Ca, Mg, and Fe. The composition of moderately to intensely altered footwall rhyolite indicates that hydrothermal alteration was dominated by processes such as destruction of primary feldspar, sericitization, chloritization, and pyritization leading to Na2O loss and elevated values for MgO+FeO.

Figure 10 The alteration facies at San Nicolás, DDH (diamond drill hole) SAL-25 (samples 45-41 under ore; 31-26 above ore, arrows are from bottom to surface), with alteration facies box-plot (Large et al., 2001). Stars represent other drill samples. (see Table A1 in the electronic supplement).
The alteration facies of the San Nicolás deposit can be geochemically distinguished in one diagram, which utilizes two multielement ratios to monitor relative changes in the concentrations of MgO, FeO, K2O, Na2O and CaO (the alteration boxplot; Large et al., 2001; Figures 9, 10). The alteration index (AI = 100*[MgO+K2O]/[MgO+K2O+Na2O+CaO]; Ishikawa et al., 1976) quantifies Ca and Na depletion and enrichment relative to Mg and K. The AI increases as a result of plagioclase destruction or chlorite, muscovite, and K feldspar formation. In contrast, relative K depletion due to K-feldspar alteration or Ca enrichment associated with carbonate alteration results in low AI values. On the other hand, the carbonate-chlorite-pyrite index CCPI =100*[MgO+FeO]/[MgO+FeO+Na2O+K2O]; measures total alkali depletion relative to Mg and Fe enrichment associated with chlorite and pyrite formation and dolomiteand/or ankerite-rich carbonate precipitation. A combination of both indices in the boxplot (Figure 10) enables discrimination of the main alteration assemblages and separates unaltered or weakly altered samples, representing background compositions, from samples with hydrothermal alteration (Large et al., 2001). Least altered footwall volcanic rocks have AI values between 28 and 36 and CCPI values between 44 and 64 (Figure 10), reflecting relatively high, primary concentrations of alkalis and low Fe and Mg concentrations (samples BSN26-27-28).
In contrast, intensely altered, pyrite-rich rhyolites plot in the upper right-hand corner of the boxplot (AI: >90, CCPI: 50-95). BSN-29 (CCPI=80, from 35 m to ore body), BSN-15 (CCPI=81.8 from 100 mto the ore), BSN-77 (CCPI=75.6 from 80 m to the ore), and BSN-48 (CCPI=78.8 from 83 m to ore body). The large range of CCPI is related to variations in the proportions of chlorite, biotite and muscovite.
The mottled alteration facies has intermediate AI and CCPI values, partly overlapping the fields for the least altered rhyolite and pyrite-rich alteration facies. Samples of the carbonate alteration facies plot along the upper boundary of the diagram (CCPI >90) and the large spread in AI values reflects variations in the proportions of carbonate, chlorite, and tremolite. Samples of the quartz-K feldspar alteration facies overlap with samples of the mottled alteration facies and define a trend toward the composition of K feldspar. Most samples of the hanging-wall dacite and a few samples of weakly altered footwall rhyolite have low AI values (<20), which is consistent with albite alteration. Samples of quartz-eye porphyry show a wide distribution in the alteration box-plot, with data points overlapping with the least and weakly altered footwall rhyolites and mottled alteration facies (Figure 10).
Immobile trace element ratios- constraints on primary geochemistry
Geochemical discriminant diagrams are relatively common for mafic volcanic rocks (Pearce and Cann, 1973; Winchester and Floyd, 1977, and Wood, 1980). Discriminant diagrams are commonly (although not exclusively) based on elements that are considered to be immobile under most geologic conditions. These include selected major, trace, and rare earth elements. Some well-known discriminant diagrams use absolute concentrations of element pairs such as Zr-Y, NbY, Zr-Nb, Zr-Ti, Th-Ta, Th-Yb, Th-Hf, and La-Yb, whereas others use element ratios. Because the behavior of some elements is comparable (i.e., Zr and Hf, Nb and Ta, Y and Yb), these elements are commonly used on diagrams, depending on the preferred analytical technique. In order to avoid common geochemical problems associated with mass loss and mass gain caused by extensive hydrothermal alteration of the host volcanic rocks around VMS deposits, the diagrams presented are based on element ratios, rather than on element concentrations (Gorton and Schandl, 2000, Schandl and Gorton, 2002).
A Zr/TiO2-Nb/Y discrimination diagram (Figure 11) shows that immobile element data are consistent with the classification of volcanic rocks of San Nicolás (Winchester and Floyd, 1977). Hanging wall orerelated rhyolite flow-dome (RFD) plots in the field for rhyolite despite the extensive modifications of bulk-rock composition due to alteration. This suggests that rhyolite was a typical acid rock essentially homogeneous in primary composition prior to hydrothermal alteration. The immobile element signature of hanging-wall dacite is similar to ratios of fresh volcanics of dacitic and rhyodacitic composition. Samples of quartz-eye porphyry also plot in the field for dacite and rhyodacite because of their lower Zr/TiO2 ratios compared to footwall rhyolite. Samples of footwall strong chl-py-ser K-feldspar plot in the field for basaltic and andesite-basaltic rocks because of their lower Zr/TiO2 ratios compared to hanging-wall weak chl-ser mafic rocks.
Base metals and mobile trace elements
Concentrations of Cu, Pb, Zn, Ba, Rb, Sr, Tl, As, Bi, and Mo were plotted against Na2O, because Na depletion is an indicator of increased alteration intensity in the footwall alteration zone, whereas albitealtered hanging-wall dacite has high Na2O values (Figures 12, 13). The base metal contents of samples from the footwall alteration zone vary substantially and intensely altered rhyolite often has one order of magnitude higher Cu, Zn, and Pb concentrations than least altered rhyolite, hanging-wall dacite, and quartz-eye porphyry (Figures 12, 13). However, there is overlap and no clear correlation between alteration intensity and base metal concentrations.

Figure 12 Concentrations of Cu, Pb, Zn, and Ce for SAL-25 DDH, in hangingwall rhyolite, and quartz-eye porphyry. The decreasing concentration of Na2O is an indicator of increased intensity of hydrothermal alteration.
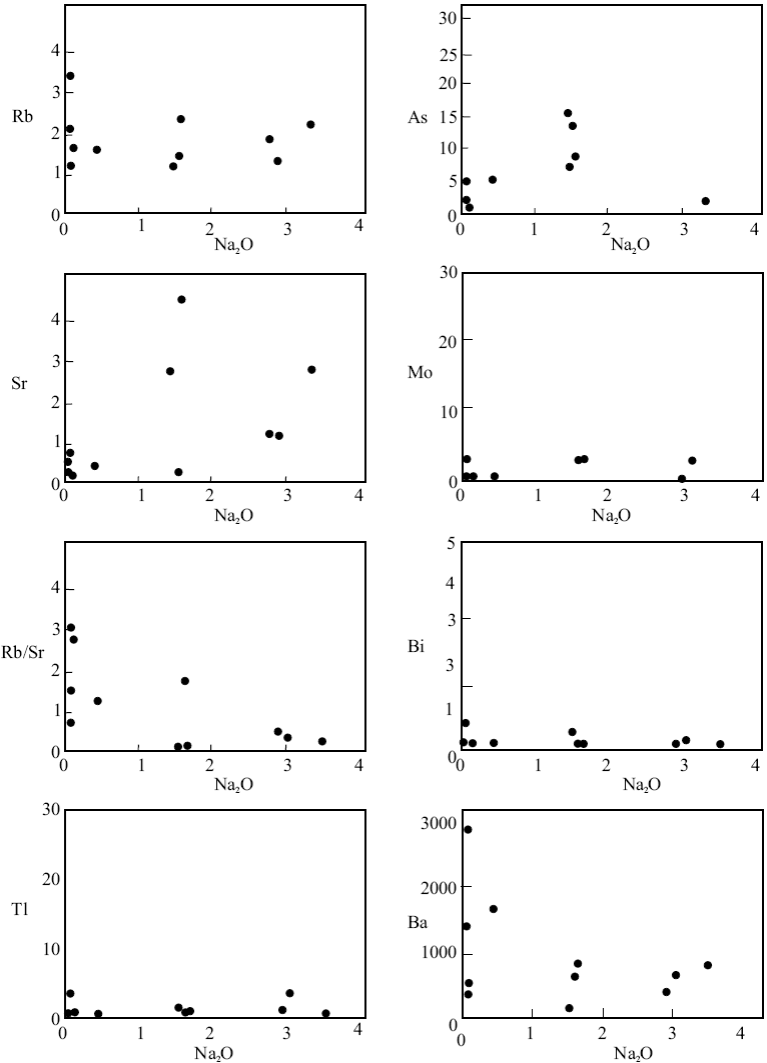
Figure 13 Variation diagrams for SAL-25 DDH showing the variability of trace element concentrations in hanging-wall rhyolite and quartz-eye porphyry (Rb, Sr, Rb/Sr, Tl, As, Mo, Bi and Ba ppm vs. Na2O %).
Variations in Ba, Rb and Sr contents and in the Rb/Sr ratio are probably controlled by differences in mineralogical composition (Figures 12, 13). The increase in Ba and Rb from the least altered hanging wall mafic volcanics (samples BSN-26-31) to some samples of moderately to intensely altered footwall mafic volcanics (samples BSN41-45) may be related to the formation of muscovite and K feldspar replacing primary plagioclase with Ba and Rb for K. Thus, muscovitepoor samples of the intense quartz-pyrite-ser alteration facies (Figure 7, Table A1) have low Ba and Rb concentrations. Extremely low values for Ba and Rb occur in several Na-rich samples of the hanging wall rhyolite where albitization of feldspar led to concurrent K, Ba and Rb depletion. Samples of cabonate alteration facies are barium-rich (commonly about 1 wt %), which is related to the occurrence of barite. The somewhat erratic variations in Sr concentrations are probably related to occurrences of epidote and calcite where Sr replaces Ca.
The concentrations of Tl, As, Bi and Mo (Figure 13, Table A2 in the electronic supplement) are close to or below the detection limit for most samples with Na2O values higher than approximately 1 wt %. In contrast, samples of altered rhyolite, with Na2O <2 wt % show a large range of variation of As with maximum values typically 10 to 15 times higher than in least altered rhyolite.
GEOCHEMICAL INDICATORS OF PROXIMITY TO THE SAN NICOLÁS DEPOSIT
Representative drill holes from San Nicolás deposit were selected to illustrate the relationship between geochemical changes and proximity to the massive sulfide lenses. The orientation of these drill holes with regard to the San Nicolás Formation (rhyolite flow-dome, RFD in Figure 4) is variable. (Figure 7). Drill hole SAL-25 was collared in no altered hanging wall rhyolite ~220 m over the San Nicolás Formation. Drill hole SAL-35 (100 m to the south-west of the section in figures 3, 5) cuts 245 m of several zones of quartz-pyrite alteration facies. This drill hole starts in no altered basalts and ends with quartz-K feldspar alteration facies ~40 m stratigraphically below the San Nicolás Formation. Drill hole SAL-25 was selected to examine the geochemistry of a thick lava unit directly overlying the massive sulfides in San Nicolás deposit.
Footwall halo
Major elements and alteration indexes
The data from drill hole SAL-25 illustrate the geochemical variability of the San Nicolás alteration zone in the hanging wall (samples BSN-41-45), which is characterized by elevated values for MgO, S, AI, and CCPI in San Nicolás due to the abundance of chlorite-rich quartz-pyrite alteration facies.
In drill hole SAL-25, the vertical concentration of Na2O decreases toward the San Nicolás Formation and values <1 wt % are common in the upper part of the mottled alteration facies between 384 to 420 m (Table A1, Figure 14). The concentration of MgO increases from regional background levels (2-3 wt %) to values around 9.82 wt % in the central part of the alteration zone. Alteration index and CCPI values are close to values typical (35-64) for the least altered rhyolite in the lower part of the mottled alteration facies but increase toward the San Nicolás Formation sulfides (203 m depth) (45-80). Sulfur concentrations are high (2.61 %) at the quartz-pyrite alteration facies just below the massive ore body (384 m), and low (0.03 wt %) over the sulfides (203 m).
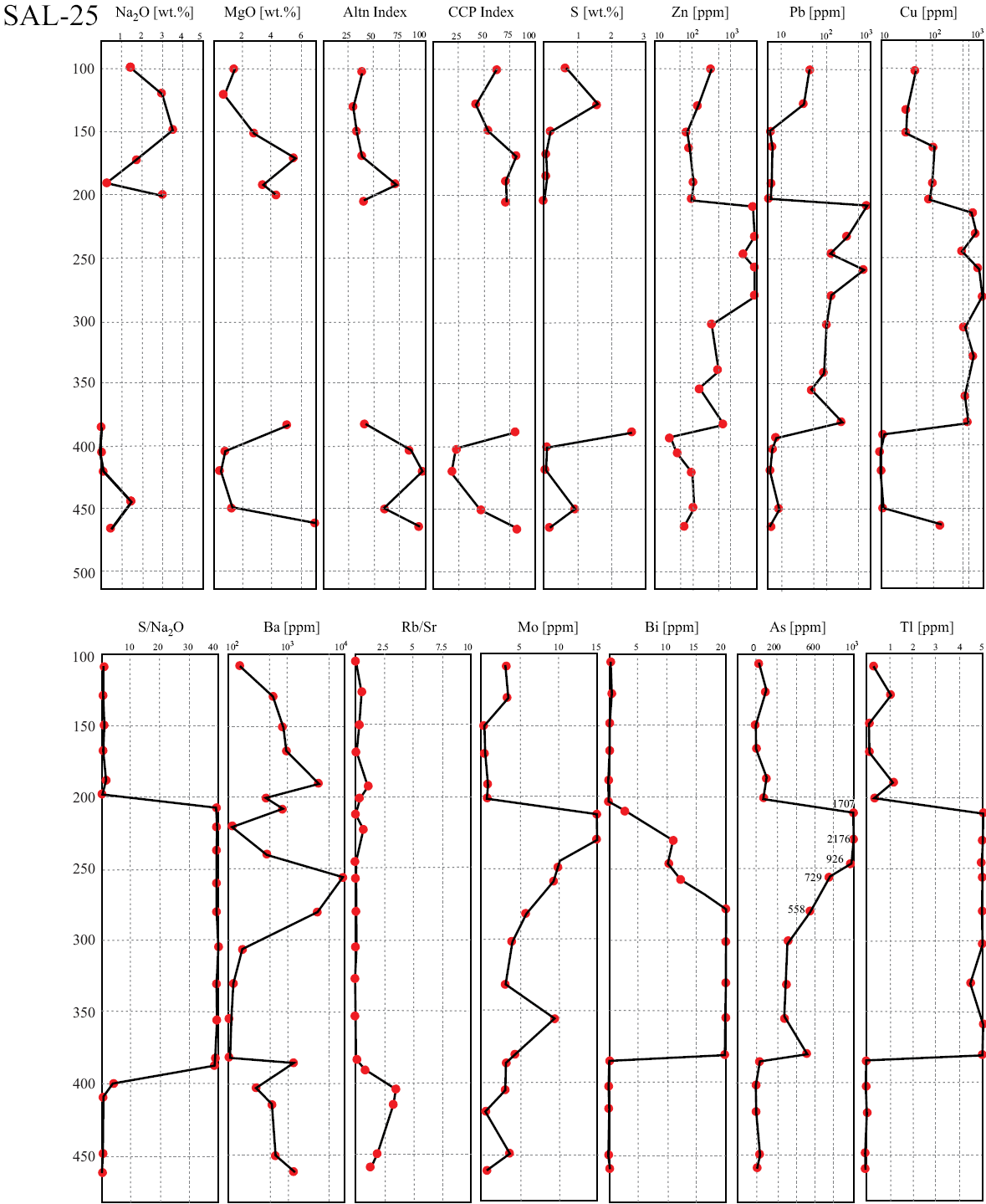
Figure 14 Geologic logs and geochemical data for SAL-25 DDH through the hanging wall and footwall alteration zone in San Nicolás. The orebody is between 204 and 383 m deep, a vertical distance of 179 m.
In drill hole SAL-25, Na2O values are below 1.62 wt % for most samples and AI values >61.4 predominate (Figures 6, 14). A drop in AI values just below the San Nicolás Formation (203 m depth) is correlated with a tremolite and barite-rich sample of strong chl-py-ser-K-feldspar alteration facies. The pattern for S drops from 2.61 wt % to almost zero, although most samples have concentrations <1 wt % and one zone of chl-py-ser-K-feldspar alteration facies has exceptionally high S values (≥ 2 wt %). This zone is also marked by peaks in MgO concentrations (3.0 and 9.82 wt %). In contrast, the CCPI values increase smoothly from 43 at the start of drill core SAL-25 to values >79 just below the ore.
Increase of MgO, AI and CCPI and depletion of Na2O and S are characteristic geochemical features for the San Nicolás footwall alteration zone. The potential of these parameters to be a guide in base metal exploration is variable and depends on the specific alteration facies present. In San Nicolás, the smooth increase in CCPI (BSN-29-31) is an excellent guide to ore, due to intense Na2O depletion and abundance of chlorite-rich quartz-pyrite alteration facies. Also, a comparatively smooth trend in Na2O depletion and a relatively subtle increase in CCPI (from 25-50) are observed when approaching massive sulfides orebodies in any direction, up or down (Table A1).
Base metals
Concentrations of base metals in altered rhyolite show erratic variations with comparable ranges in San Nicolás. Although values higher than background are predominant, numerous samples show base metal concentrations within the range of the least altered rhyolite. There are no consistent trends in the base metal data that could be used as a guide to the massive sulfides in San Nicolás. Consequently, analyses of Cu, Pb, and Zn have a low potential as vectors to ore (Figure 14).
Trace elements
In general, concentrations of Ba show consistent changes with distance from the San Nicolás favorable unit, and increases with CCPI values. Ba-rich samples in the vicinity of massive sulfides are potentially useful as mine-scale exploration vectors and correlate with local occurrences of barite. In San Nicolás the chlorite-rich quartz-pyrite alteration facies are marked by strong depletion in Ba. A general increase in the Rb/Sr ratio can be observed in all the ore body of San Nicolás; however, there is considerable scattering and overlapping with the range for the least altered rhyolite. Just below the San Nicolás favorable unit, the Rb/Sr ratio decreases dramatically to the presence of disseminated tremolite alteration facies. The concentrations of Mo, Bi, and As are typically less than 1 ppm in the least altered footwall rhyolite. In contrast, altered footwall rhyolites often contain several to >10 ppm of these elements. In San Nicolás, the concentrations of these elements vary erratically and a correlation with distance from ore is not apparent. In the west part of San Nicolás, elevated values of Mo and As (>10 ppm) are restricted to the quartz-pyrite alteration facies immediately below massive sulfides. In general, Tl concentrations are close to, or below, the detection limit (<0.5 ppm) for all samples regardless of alteration facies. However, elevated Tl values (>3 ppm) occur in samples just at the begining of the San Nicolás favorable unit in drill hole SAL-25 (from 210 to 380 m, Figure 14).
Barium concentrations are strongly controlled by mineralogy of alteration (i.e., abundance of muscovite, K feldspar, or barite) and may be enriched or depleted in the hanging wall alteration zone compared to the least altered footwall volcanic rocks. It is typical that Ba is concentrated in the upper parts of ore bodies, but its concentration is depleted at the lower parts where Cu is abundant. The Sr is a good indicator of feldspar-destructive alteration and generally increases toward the San Nicolás favorable unit. However, the occurrence of Ca-bearing minerals (epidote, calcite, tremolite) have a strong effect, causing substantial distortion of the trend in drill holes SAL-25 (samples BSN-41-51), and SAL-35 (samples BSN-3-24-25). Elevated concentrations of Mo, Bi and As distinguish the footwall alteration zone from the surrounding least altered rhyolite and may be useful in district-scale base metal exploration (Figures 12, 13).
Hanging wall halo
The hanging wall andesite lavas in drill hole SAL-37 are relatively homogeneous in geochemical composition (Table A1). However, high Na2O values (2.6-3.3 wt %) and alteration index AI=36 indicate albite alteration in its upper part, which is also accompanied by low concentrations of Ba. Increasing albite alteration in samples near to the San Nicolás favorable unit indicates that this type of alteration is related to the hydrothermal activity associated with mineralization at San Nicolás. Nevertheless, andesite sampled at approximately 50 m stratigraphically above the San Nicolás favorable unit (SAL-25, 240 m; Figure 14) shows elevated concentrations of Zn, Pb, and Bi compared to mafic flows higher in the sequence. This sample also contains sulfur (0.07 wt %), which is unusual because, in general, rhyolite is extremely sulfur-poor with values generally below the detection limit (<0.01 wt %). This enrichment in S, Zn, Pb and Bi may be related to localized late activity of the San Nicolás hydrothermal system.
DISCUSSION
Hydrothermal alteration of the volcanic rocks
The San Nicolás alteration zone consists of six alteration facies with a distinctive mineralogical and geochemical characteristics. Based on geological, mineralogical and geochemical data, a conceptual model for the evolution of the San Nicolás hydrothermal system is presented (Figure 7). Although a temporal evolution of the hydrothermal system is suggested, other alteration processes may have occurred concurrently in different parts of the system.
Carbonate alteration
The origin of the carbonate alteration has been examined in detail by Herrmann and Hill (2001) at the Thalanga deposit, Australia, who concluded that carbonate-tremolite-chlorite alteration facies represents the metamorphic equivalent of intense chlorite-calcite ± dolomite alteration, which occurred in footwall rocks close to the sea floor by a process involving mixing of hydrothermal fluids and seawater.
The abundance of the carbonate-tremolite-chlorite alteration facies in central San Nicolás suggests that the volcaniclastic units and the fault pathway (Figures 5, 10) provided favorable conditions for mixing of upwelling hydrothermal fluids with ambient seawater.
Hydrothermal carbonate alteration is common in VMS deposits and has been described from several deposits in Tasmania, such as Rosebery deposit, (Lees et al., 1990); Hercules deposit, (Zaw and Large, 1992); Hellyer deposit, (Gemmell and Large, 1992), in VMS russian districts (Smirnov, 1968, 1976; Smirnov and Gorzhevskii, 1978; Starostin, 1984), in Chisel Lake, Canada, (Galley et al., 1993), and in Kuroko, Japan (Shikazono et al., 1998). It has also been observed in modern, submarine hydrothermal systems Middle Valley, northern Juan de Fuca Ridge, (Goodfellow et al., 1993). In general, oxidizing conditions and low temperatures (<200 °C) are inferred for carbonate alteration. By analogy, carbonate alteration at San Nicolás is interpreted to represent a relatively low temperature process, which occurred at an early stage during the development of the San Nicolás hydrothermal system (Figures 7, and 10).
Phyllosilicate-dominated alteration
Feldspar-destructive, muscovite-chlorite-biotite-rich mottled (strong chl-py-ser K-feldspar) alteration facies, (Figure 7). The bulk of the footwall alteration zone consists of the mottled facies, which shows destruction of primary feldspars, formation of hydrothermal phyllosilicates, and precipitation of disseminated pyrite. In comparison mineral assemblages in modern submarine hydrothermal systems with sulfide mineralization on the seafloor suggests that hydrothermal phyllosilicates included sericite, chlorite and clay minerals, such as illite and smectite (Binns and Scott, 1993). The muscovite-chlorite-biotite mineral assemblages observed in mottled alteration facies represent the metamorphic equivalents of these hydrothermal phyllosilicates.
The textural diversity of the mottled alteration facies and the abundance of apparent clastic textures indicate that multistage alteration processes were common. These processes may have been related to variations in fluid composition, temperature and changing water/rock ratios. The continuous lateral extent of the mottled alteration facies suggests that upwelling of hydrothermal fluids was diffuse (Figure 8).
The mottled alteration facies has been intersected by drill holes down to a stratigraphic depth of at least 150 m below the San Nicolás favorable unit (Figure 10); however, its full extent into the footwall is unknown. Broad, feldspar-destructive alteration zones of similar lateral dimensions as at San Nicolás have been recognized below Mesozoic VMS deposits in the Francisco I. Madero district, in central Zacatecas, (Vassallo, 1995). These can be traced for up to 300 m into the footwall stratigraphy where they become narrower and more confined. Hence, the apparently stratabound footwall alteration zone at San Nicolás may represent the upper part of a similarly broad, transgressive zone of hydrothermal fluid upflow.
Quartz-K feldspar alteration facies type has rarely been reported from alteration zones associated with VMS deposits. Nevertheless, K feldspar has been observed in the outer parts of alteration systems associated with the Kuroko deposits in Japan (Shirozo, 1974, Shikazono et al. 2008) and the Que River deposit, Tasmania, Australia, (McGoldrick and Large, 1992). At Que River, K feldspar is associated with silicification and Au-rich mineralization, interpreted to have formed by mixing of ore fluids of moderate temperature (200-250 °C) and seawater.
By analogy with these studies and based on the observation that quartz-K feldspar alteration facies is restricted to the outer (cooler?) parts of the San Nicolás hydrothermal system, it is inferred that this type of alteration took place under relatively low temperature conditions and low water/rock ratios. The formation of K feldspar rather than sericite suggests that the pH of the hydrothermal fluids increased from acidic in the central parts of the hydrothermal alteration system to near-neutral on its fringes.
It is inferred that mixing of hydrothermal fluids with seawater at the margin of the hydrothermal system could have caused simultaneous cooling and neutralization of the fluid, resulting in the formation of the quartz-K feldspar alteration facies. Variations in the influx rate, temperature, composition, and pathways of the hydrothermal fluids probably led to changes in the location of quartz-K feldspar alteration and, overprinting with the mottled alteration facies.
Mineralizing alteration
The fault zone of the quartz-pyrite alteration facies is interpreted to represent the principal pathways of hydrothermal fluids during mineralizing hydrothermal activity (Figures 5, 6). This interpretation is supported by the high abundance of disseminated pyrite and pyrite veins, the substantially elevated concentrations of S, base metals, and trace elements, such as As, Mo, and Bi, and the observed connection between massive sulfides and quartz-pyrite alteration facies. However, the mechanism that controlled discharge of hydrothermal fluids at San Nicolás is the growing faults systems. Synvolcanic faults have been recognized (Vassallo, 2003), and a comparison of volcanic facies architecture and the distribution of alteration facies showed that intense hydrothermal fluid flow was controlled by Jurassic faulting and in part by lithofacies. The mineralizing event was between 148.9 Ma and 146.5 Ma, whereas all the volcanic process involved lasted from 148.9 Ma to 117.94 Ma, about 31 Ma (Figure 4).
The quartz-pyrite alteration facies also exists as stratiform zones immediately below the massive sulfides. These probably formed as a result of lateral flow of hydrothermal fluids below the paleo-seafloor. This process appears to have been particularly important in southern part of San Nicolás where the stratiform ore zone is up to 50 m thick (Figures 5, 6).
In contrast to the quartz-pyrite alteration facies, which is ubiquitous below the lowest San Nicolás sulfide lenses, the chlorite-pyrite alteration facies is restricted to a narrow zone underlying massive sulfides in San Nicolás (Figures 5, 6). This alteration facies (samples BSN-07-08) is distinctive in having the highest enrichment in Mg, Fe, and substantial depletion of SiO2. Possibly, a relatively short lived episode of hydrothermal alteration characterized by Mg-rich, Si-poor fluids forming zones of chlorite-pyrite alteration facies occurred in upper San Nicolás. Subsequently, the conditions of alteration and/or the composition of the hydrothermal fluid may have changed promoting quartz-pyrite alteration.
Geochemical proximity indicators to the San Nicolás deposit
The footwall geochemical halo of the San Nicolás deposit can be devided into an outer and an inner zone (Figures 5, 6). Furthermore, a small hanging wall halo was locally developed. Notwithstanding the mineralogical and geochemical variability of altered rhyolite there are several geochemical features that distinguish hydrothermally altered rhyolite from the surrounding, least altered volcanic rocks and are therefore important for the recognition of ancient, mineralizing hydrothermal systems in felsic volcanic successions. These include elevated MgO, S, AI, CCPI, Rb/Sr, Mo, Bi and As, and depletion of Na2O.
Some geochemical parameters show well-constrained correlations with proximity to ore at San Nicolás. For example, the CCPI values increase steadily from background values from 25 to values >90 just below the San Nicolás favorable unit in San Nicolás. High values of Tl, Ba, S, AI, CCPI, Rb/Sr, Mo, or As indicate immediate proximity to ore defining the inner footwall halo (Table A1, A2).
Alteration of the hanging-wall volcaniclastic rocks is generally characterized by weak alteration facies, which formed as a result of background alteration processes unrelated to the mineralizing hydrothermal system. Nevertheless, rare minor geochemical anomalies (elevated S, Zn, Pb, and Bi) in basalt 50 m above the massive sulfides suggest that some late-stage hydrothermal activity was locally ongoing at the time of basalts emplacement in lower Cretaceous, or when the faults were still moving during Cretaceous time (Figure 14).
CONCLUSIONS
A stratabound footwall alteration zone underlies the San Nicolás massive sulfide lenses and extends for at least 200 m stratigraphically below the deposit. It envelops discordant zones of intense hydrothermal alteration, which are directly connected to the massive sulfides ore lenses in the San Nicolás rhyolite Formation (146.5 Ma). Textural, mineralogical and geochemical evidence indicates that hydrothermal alteration in the footwall involved destruction of primary feldspar, silicification and formation of pyrite, calcite ± dolomite, K feldspar, and phyllosilicate (chlorite, sericite and clay minerals).
There are 6 alteration facies (Figure 7) from weak to intense degree: 1. Weak chlorite-sericite (weak chl-ser) alteration. 2. Chlorite-pyritesericite (chl-py-ser) alteration. 3. Feldspar-destructive, muscovitechlorite-biotite-rich mottled (strong chl-py-ser K-feldspar) alteration facies. 4. The quartz-K feldspar (intense qtz-py-ser) alteration facies. 5. Disseminated tremolite and patchy carbonate-tremolite-chlorite (Ab-calc-ep) alteration facies. 6. Intense, quartz-pyrite (silica alteration and cpy-py stringers) alteration facies.
These alteration facies diversity implies that the development of the San Nicolás hydrothermal system was a complex process, probably influenced by volcanic facies architecture, fluctuations in the supply of hydrothermal fluids, changes in fluid temperature and composition, variable water/rock ratios, and interaction of hydrothermal fluids with seawater.
Chlorite-carbonate alteration facies of variable intensity was the premetamorphic precursor to the disseminated tremolite alteration facies and carbonate-tremolite-chlorite alteration facies, which formed in the near seafloor environment. Diffuse upwelling of acidic, seawaterderived hydrothermal fluids caused destruction of primary feldspar, precipitation of pyrite and formation of hydrothermal phyllosilicates (muscovite-chlorite-biotite facies) in a broad zone below the paleoseafloor, presently occupied by the mottled alteration facies. Intense hydrothermal alteration, represented by quartz-pyrite alteration facies, exists in both discordant zones crosscutting the footwall and in stratiform zones immediately below the massive sulfide lenses. This alteration facies is inferred to have formed during mineralizing hydrothermal activity, and the fault zones are interpreted as feeder zones supplying metal-rich fluids to several hydrothermal fields separated in space along several Jurassic faults (Figures 6, and 7).
The geochemical composition of the altered rhyolite in the San Nicolás footwall alteration zone is strongly variable, reflecting the diversity of alteration processes during hydrothermal activity. However, regardless of alteration facies, Na2O depletion is an ubiquitous feature of moderate to strong hydrothermal alteration at San Nicolás. This is commonly coupled with an increase in AI values (except for carbonate alteration as seen in Figure 10). Moderately altered rhyolite represents the bulk of the footwall alteration zone, and is geochemically characterized by elevated values for Mg, Rb/Sr, AI, CCPI and trace elements, such as Mo, Bi and As. Immediate proximity to the massive sulfides is typically indicated by elevated As, Mo, and Tl concentrations and Ba enrichment. These geochemical characteristics, together with geologic and petrographic observations, may provide useful tools for the recognition of ancient mineralizing hydrothermal systems in felsic volcanic sequences and may help to guides exploration for massivesulfide deposits at the central part of Mexico along the Jurassic faults.