Introduction
The Northern Dead Sea Fault (NDSF) is widely recognized as an active tectonic structure in the eastern Mediterranean region evidenced by many large historical earthquakes (Ambraseys and Barazangi, 1989; Ambraseys and Jackson, 1998; Sbeinati et al., 2005). The fault forms a major continental transform plate boundary between the Arabian plate to the east and the African plate to the west, and it accommodates their differential shift. Thus, the system has become the focus of attention for many researchers who studied its seismotectonic and geologic evolution (Barazangi et al., 1993; Butler et al., 1997; Brew et al., 2001; Meghraui, 2015). Although the NDSF is marked by a rich historical catalogue of large earthquakes, it currently shows an apparent lack of seismicity with a prevailing status of quiescence. Moreover, analysis of recent seismic data revealed that the earthquake activity along most segments of the NDSF in western Syria is presently distinguished by producing a little number of low magnitude seismic events (Al-Hilal and Abdul-Wahed, 2018). Furthermore, Meghraoui et al. (2003) found evidences for ~830 years of seismic quiescence along the NDSF at Al-Harif site of Missyaf fault segment. Additionally, recent Global Positioning System (GPS) measurements along the plate boundary in NW Syria showed a fairly small relative motion between the Arabian and African plates with average geodetic velocities of ~0.6 mm/year (Alchalbi et al., 2010). However, these results seem to be inconsistent with the long historical record and the consequences of field observations that bear out the activity and seismogenic potential of the concerned fault system. Thus, even though the concerned region seems nowadays to pass through a period of seismic quiescence, this temporal status is interpreted by most seismologists as a probable reflection of the slow rate of strain accumulation in a locked fault system (e.g. Alchalbi et al., 2010; Kobeissi et al., 2015).
From tectonic point of view, faults are described as complex structures that are generated under the actions of high stresses coupled with accumulated strains which commonly result in significant crustal deformations with massive zones of crushed and broken rocks. Hence, faults often exhibit higher permeability environment compared to the surrounding unaltered rocks. This property may turn most faulted zones into favored paths for upward migration of emanated soil gases during their way to the Earth’s surface. Among these gases, radon, a natural radioactive element constantly generated from soil and rocks is emitted to the atmosphere, particularly through weak zones such as faults (Al-Hilal and Abdul-Wahed, 2016; Mojzes Andrej et al., 2017). Radon (222Rn, with a half-life of 3.825 days) is a progeny nuclide of radium (226Ra), which in turn is generated from the natural decay series of uranium (238U). The gaseous, radioactive and inert nature of radon makes it a significant tracer which can be used to investigate some crustal activities that induce gas emission in the ground (King et al., 1996; Richon et al., 2010).
Radon is commonly transported within the earth’s crust by two basic mechanisms, diffusion and/or convection of the gas flow (Tanner, 1964; Etiope and Martinelli, 2002). However, there are some important factors which could facilitate the migration process of radon and enhance the distance reached in the ground. Among these factors is the groundwater flow which plays a major role as driver of radon transport through permeable geologic medium, and could be brought upwards to the surface by a rising flux of some other natural gases, (e.g. CO2 and He) which act as radon carriers via fractures and rupture zones. Accordingly, the primary goal of this work is to investigate the effects of tectonic activity on soil radon emanations across two active fault structures - the Qastoon and Al-Harif segments that are located along the NDSF in northwestern Syria.
The Study Area
The Qastoon and the Al-Harif faults represent prominent structural components of the NDSF in western Syria (Fig. 1). The Qastoon segment is located at the eastern boundary of the Ghab basin, at latitude of (35°.41'.30N) and longitude of (36°.24'.05E). The geological setting of the fault zone and its surroundings is characterized by thick Pliocene lacustrine sediments consisting of calcareous marls, silt, pebbles and clays alternating with repetitive sequences of sandstones and well cemented conglomerate beds at depth. The site is bordered from the north and the east by notable outcrops of Pliocene basalts along with massive deposits of chalky/clayey limestone which belong to the Middle and Upper Eocene (Devyatkin et al., 1997). On the other hand, the north trending Al Harif fault segment is situated along the eastern flank of the Syrian Coastal Mountains Range, about 4 km north of the Missyaf city, at latitude of (35°.06'.01N) and longitude of (36°.20'.05E). The fault interacts with geologically young formations of late Pleistocene and Holocene that are composed mainly of alluvial deposits, sandy loams, clays, flood plains, coarse and fine gravels in a sandy matrix (Ponikarov, 1966).
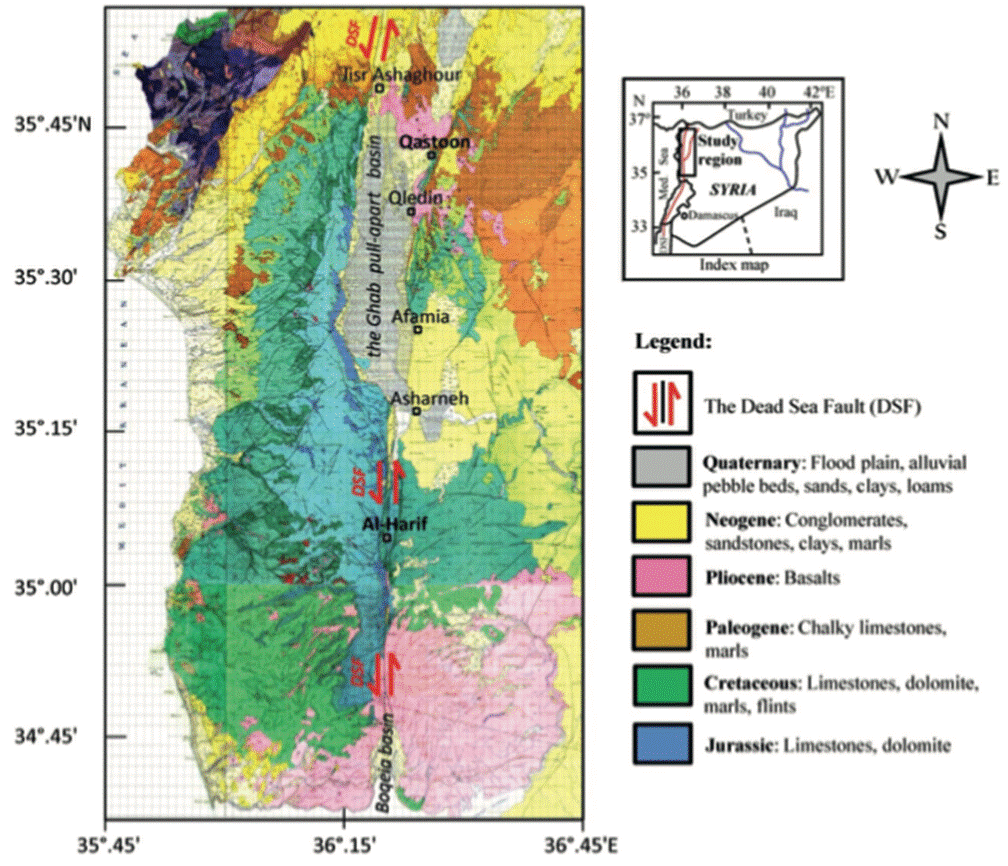
Figure 1 Geological map of western Syria showing the locations of the radon measurement sites at the Qastoon and the Al Harif faults.
Tectonically, the left-lateral NDSF forms a key tectonic feature in western Syria that stretches over a distance of about 150 km in parallel to the Syrian Coastal Mountains Range (Brew et al., 2001). Along this distance, two major fault structures were considered important for the purpose of this study; the Qastoon and the Al Harif segments (Fig. 2). Field investigations in the study region revealed some significant geomorphic and topographic expressions which attest to the ongoing tectonic activities of the concerned fault zone. Evidences include the occurrence of recent lava flows; break in slope features; faulted young alluvial fans and the common occurrence of distinct deflected streams which indicates the role of tectonic activity in influencing the outline of drainage system (Meghraui, 2015). Besides, the formations of distinguished colluvial wedges which are commonly associated with recurrent ruptures of fault where each fault rupture creates a scarp with a sharp free face. Then, sediments that eroded from the free face is shed across the fault and filling up on the footwall of the fault due to slope failure as a result of erosion and re-deposition during successive dip slip of faulting, producing a colluvial wedge (Fig. 3). In our case, the observed colluvial wedges along the eastern Ghab fault were developed mostly due to tectonically induced gravity landslide of Pliocene-Quaternary unconsolidated sediments that are eroded from the free face of the fault scarp and accumulated at the footwall of the fault plane, producing a typical colluvial wedge, as shown in Fig. 3a, b and c. Accordingly, the interpretation of colluvial wedge stratigraphy and development may assist paleoseismologists to decode an ‘event-stratigraphy’ of past earthquakes (McCalpin, 2009).

Figure 2 Tectonic map of western Syria showing the general trend of the northern Dead Sea Fault System (DSFS), including the Missyaf, Al-Harif, Qastoon and the Ghab faults.

Figure 3 Field Photographs illustrating the development of typical colluvial wedges that formed along the eastern bounding fault of the Ghab pull-apart basin. The dashed red and white lines delineate the fault plane and the colluvial wedge, respectively.
From seismic point of view, the NDSF in western Syria and southern Turkey has been the site of several strong earthquakes (Ms~7.0) that occurred during the last millennium (Ambraseys and Jackson, 1998; Sbeinati et al., 2005; Akyuz et al., 2006). The return period of large earthquakes in Syria was estimated to be about 200-350 years (Ambraseys and Barazangi, 1989). However, new evidences from palaeoseismology, archaeoseismology and historical seismicity along the NDSF in Syria revealed a seismic gap of ~830 years along the Missyaf fault segment (Megrhaoui et al., 2003). Although such temporal seismic quiescence may imply that the fault has been seismically inactive in the last few centuries, it might be a sign of high level of seismic hazard in the region due to a probable slow rate of interseismic strain accumulation in a locked fault (Alchalbi et al., 2010). However, analysis of seismic data in Syria during the period (1995-2012) revealed that the earthquake activities in the country produce a little number of low magnitude events (Al-Hilal and Abdul-Wahed, 2018). However, Barazangi et al. (1993) concluded that the apparent lack of recent seismicity along the NDSF should not be interpreted as minimizing the potential hazard and risk in the region. Thus, realizing the active crustal deformation, particularly in terms of strain accumulation in the fault, and the average timing for the recurrence interval of large earthquake, are essential issues for seismic hazard assessment in the region.
Radon Measurements
A total of eighty soil gas radon measurements carried out across the Qastoon and the Al-Harif faults, which represent two remarkable tectonic segments located along the northwestern margin of the Arabian plate in Syria (Figs. 4 & 5). The selection of these two structures for radon measurements was based on the results drawn from previous extensive field works which revealed clear evidences of current active tectonics associated with the structural evolution of the NDSF. Accordingly, the radon measurements in the Al-Harif site were oriented in the light of evidences obtained from earlier results of archaeoseismic, palaeoseismic and historical seismicity carried out in the same area (Meghraoui et al., 2003). Similarly, the radon investigations at the Qastoon site were mainly guided by the outcome of previous geophysical surveys and morphotectonic mapping performed in the region by Asfahani et al. (2010). The ultimate conclusions of these studies attested to the ongoing tectonic activity and seismogenic potential in both the Qastoon and Al-Harif zones. In view of that, radon surveys at these two selected structures were completed using AlphaGUARD PQ2000 Pro radon monitor. In practice, radon gas is automatically drawn out into the ionization chamber of the device with a steady continuous pumping rate of 0.3L/min. For each measurement, the radon activity is sampled in 10-min intervals over a period of one hour. The system is equipped by an ionization chamber with a detection limit of 2-2000.000 Bq.m-3. The applied technique based on the principle that the action of tectonic stresses and shearing processes that commonly occur along the rupture zones of faults could remarkably enhance the level of radon emissions owing to increasing rock permeability of the deformed structures (Yuce et al., 2017). Consequently, considerable information concerning the trend and location of the fault zone, besides understanding the nature of its tectonic activity, could be achieved through analyzing the distribution pattern of soil radon emission and defining the spatial tendency of the anomalous halos in the survey area (King et al., 1996; Baubron et al., 2002; Richon et al., 2010; Al-Hilal and Al-Ali, 2010). The results in most of these researches revealed a clear association between the occurrence of high radon variations and the general trends of fault zones. This finding implies that the movement of radon in the ground is greatly dependent on the degree of soil permeability and the extent of fracturing in bedrocks.
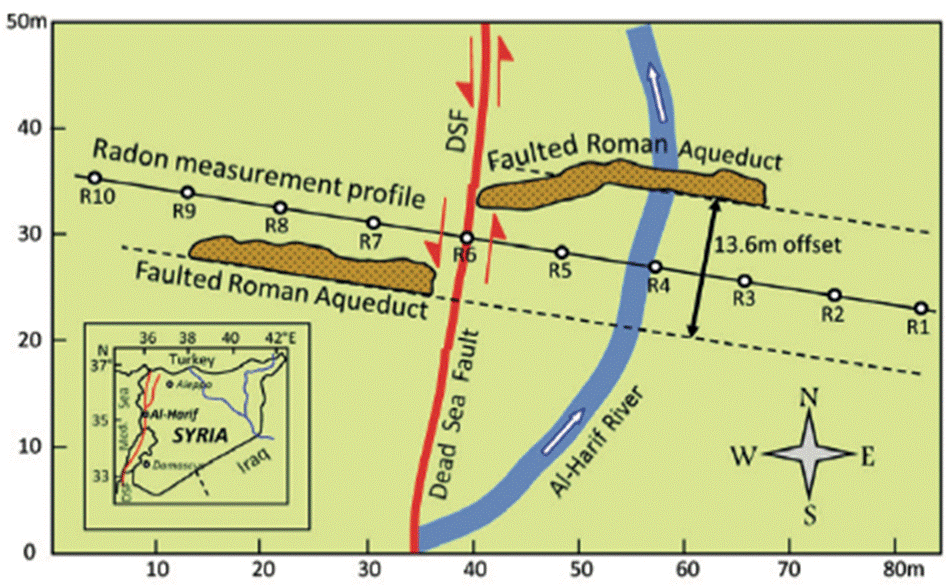
Figure 4 A sketch map of the Al-Harif site showing the trend of the Dead Sea Fault (red solid line), the radon profile (black solid line) and the location of the Al-Harif ancient aqueduct, which was faulted and displaced left-laterally by a total horizontal offset of ~13.6 m.
Data Analysis
The set of our radon data was statistically analyzed in order to define their spatial distribution with respect to the fault trend. Such processes would assist the separation between normal radon concentrations from other anomalous or tectonic related values (Table 1). The analysis includes the estimation of minimum, maximum, mean value (x), standard deviation (SD) and the coefficient of variability (CV%), which reflects the degree of radon homogeneity in the investigated site, the higher the coefficient of variability, the lower the homogeneity of radon distribution in soil. The value of CV is generally calculated through the following formula:
Table 1 Results of soil radon measurement data carried out across the Qastoon and Al-Harif fault segments in northwestern Syria
Al-Harif Fault Rn±error (Bq.m-3) | The Qastoon Fault Rn±error (Bq.m-3) | ||||
---|---|---|---|---|---|
Round 1 | Round 2 | Round 1 | Round 2 | Round 3 | |
13570±1435 | 12170±1211 | 9740±1110 | 12345±1288 | 13300±1389 | |
16450±2100 | 16480±2110 | 8780±988 | 11734±1387 | 13678±1461 | |
16730±2170 | 13100±1390 | 14870±1890 | 10988±1322 | 11850±1390 | |
29622±3766 | 45750±6112 | 11488±1366 | 13456±1400 | 15300±2000 | |
68310±8533 | 62500±7355 | 18750±2220 | 12700±1406 | 19870±2411 | |
41350±5245 | 45940±6180 | 45800±6110 | 32891±4261 | 39113±4900 | |
46540±6645 | 39300±4980 | 40500±4960 | 25366±3000 | 36300±4767 | |
17800±2255 | 13670±1400 | 50280±6653 | 53870±7411 | 56100±7306 | |
18230±2344 | 15985±2210 | 38350±4566 | 33700±4110 | 42780±5409 | |
15433±2150 | 12811±1408 | 34650±4430 | 41258±5176 | 38200±4908 | |
- | - | 23500±2975 | 31870±4100 | 31320±3980 | |
- | - | 24500±3100 | 26250±3211 | 30840±3877 | |
- | - | 14950±2000 | 24880±3005 | 20950±2560 | |
- | - | 16150±2122 | 17450±2000 | 19740±2205 | |
- | - | 13477±1730 | 19630±2200 | 18300±2100 | |
- | - | 14334±1895 | 12744±1408 | 17677±1984 | |
- | - | 11199±1398 | 13100±1590 | 13170±1605 | |
- | - | 11645±1411 | 13644±1675 | 12800±1460 | |
- | - | 10790±1302 | 11488±1367 | 12022±1400 | |
- | - | 9320±1178 | 10633±1288 | 11920±1391 | |
Average | 28404±3664 | 27771±3436 | 21154±2670 | 21500±2630 | 23762±2925 |
Min | 13570±1435 | 12170±1211 | 8780±988 | 10633±1288 | 11850±1389 |
Max | 13570±1435 | 62500±7355 | 50280±6653 | 53870±7411 | 56100±7306 |
SD | 18178 | 18679 | 13284 | 12036 | 12890 |
CV (%) | 64 | 67 | 63 | 56 | 54 |
Additionally, in order to get a better insight into the variability of radon concentration in soil, radon data was standardized in terms of probability of magnitude, which could assist in identifying anomalous radon signals (Crockett and Holt, 2011). In general, the Standardized Radon Index (SRI) is a statistical procedure by which similar data received in various formats is converted into a common format that enhances the comparison process and helps to maximize compatibility. Accordingly, the monthly mean values of radon concentration (Ci) have been standardized (Cs) by using the following formula:
Where (x) and (SD) are the mean value and the standard deviation, respectively.
Results and Discussion
According to the data listed in Table 1, it can be observed that radon concentration in the Qastoon fault varied from 8780 to 56100 Bq.m-3 with mean average value of 22139 Bq.m-3, a standard deviation of 12737 and 58% coefficient of variability. While radon concentration in the Al-Harif fault ranged between 12170 and 68310 Bq.m-3 with mean average value of 28087 Bq.m-3, a standard deviation of 18428 and 66% coefficient of variability. In order to avoid possible effects of meteorological variables on soil radon concentration, the survey was performed under comparable weather conditions during dry seasons, where air temperature varied between (35-40 °C) and (25-30 °C) for the Qastoon and Al Harif sites, respectively. Besides, the prevailing atmospheric pressure varied between (990-995 mb) and (956-960 mb) for the Qastoon and Al Harif, respectively. Further, the normal background level of soil radon activity in the study area was established in a separate stationary site that is located far from the tectonic influence of the studied faults zones. Although the average background value of local soil radon emission was set at ~10000±1200 Bq.m-3, the maximum radon peaks that measured above the predictable traces of the Qastoon and Al Harif fault ruptures showed much higher concentrations, exceeding 56000 and 68000 Bq.m-3, respectively (Figs. 6 & 7). This implies that the value of “signal-to-background ratio” indicates clear fault-associated radon anomalies with six to seven fold increases that are found in a spatial agreement with the general trends of the concerned fault zones. Such finding may verify the possibility of using radon as a reliable indicator for characterizing fault structures, and providing an insight into the significant role of local active tectonics in influencing the activity level of soil radon emissions.
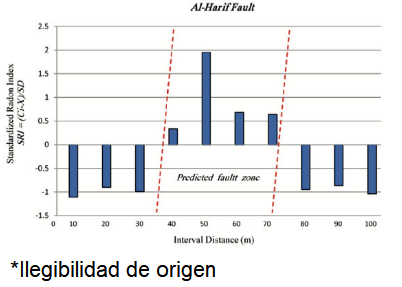
Figure 6 Standardized radon index (SRI), where anomalous radon peaks are correlated with the trace of the surface fault rupture, while negative values point to the background level.
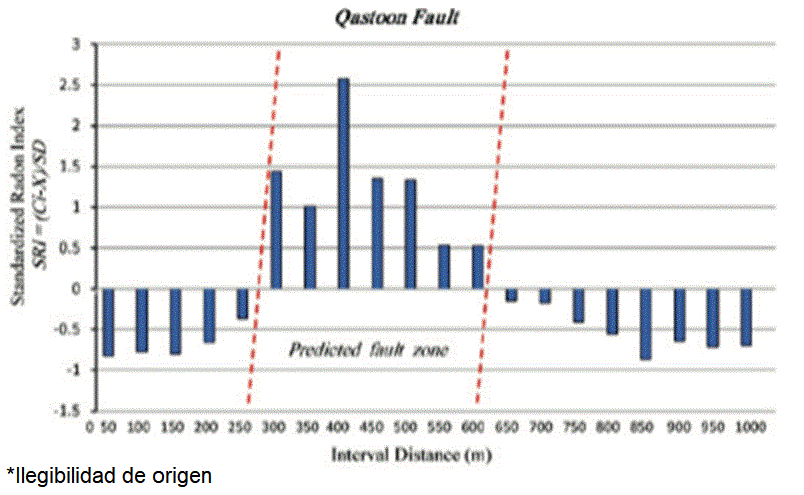
Figure 7 Standardized radon index (SRI), where anomalous radon peaks are correlated with the trace of the surface fault rupture, while negative values point to the background level.
As mentioned previously, the estimated rates of radon coefficient variability (CV) in the Qastoon and Al-Harif sites revealed values of 58% and 66%, respectively. Thus, it can be deduced that the common dispersal pattern of radon variations in both sites seems to be fairly high, and this may reflect low homogeneity model. Such disparity might be referred to the differences in the concentrations between zones with higher radon signals that are usually associated with the fault structures, and area with normal radon emission that are naturally emanated from the surrounding unaltered rocks. Additionally, so as to justify that the anomalously high radon values are mainly related to tectonic activities and not only derived from the decay of radium in soil, chemical analysis for 226Ra content in soil was accomplished in some samples collected from the same locations where the radon concentrations were measured. The results of the analysis revealed very low concentration of radium values ranging from 1.06±0.08 to 1.14±0.20 mg.kg-1, with some results below the detection level (DL= 1 mg.kg-1).
Furthermore, in order to display the differences between normal radon variations from other anomalous or tectonically-induced radon values, we used in this study the method of Standardized Radon Index (SRI). The principle of this technique is comparable to the Standardized Precipitation Index (SPI) used in drought modeling, where negative values indicate dry period and positive values point to wet period due to high precipitations (Crockett and Holt, 2011; Al-Hilal, 2016). According to the SRI method, the spatial correlation between the rate of soil radon emission and the geodynamic activity along the location of the fault zone has been highlighted. Thus, the comparison process of data has been enhanced, in order to show clear radon peaks that are compatible with the assumed trace of the fault ruptures as presented in Figs. 6 & 7. One significant result of this study is the occurrences of remarkable soil radon increases observed across the Al Harif fault in spatial agreement with a gap located between two pieces of a faulted ancient Roman aqueduct named locally as Al-Harif aqueduct (Fig. 4). As mentioned earlier, results from archaeoseismic and palaeoseismic surveys revealed evidences that Al Harif aqueduct was fractured and repeatedly displaced left-laterally by a total horizontal offset of 13.6±0.2 m due to successive displacements of three seismic events occurred along the NDSF (Meghraoui et al., 2003). Additionally, field observations indicated that the displacement of the aforesaid ancient aqueduct is well correlated with clear leftward deflections of some local streams, coinciding with the fault scarp, and most likely implying recent active tectonics along this part of the NDSF. Furthermore, Asfahani et al., (2010) carried out integrated geophysical and morphotectonic surveys in the Qastoon area, which is located along the eastern bounding fault of the Ghab pull-apart basin of the NDSF (Fig. 5). The overall results of their investigations led to the detection of an active tectonic structure which was developed through the ongoing active tectonic processes of the NDSF. Accordingly, it is worth to mention that these findings are very well corresponded with the results obtained from the present study which revealed clear anomalous rises of soil radon signals that occurred in agreement with the general trends of the Qastoon and Al Harif fault lines. Therefore, it seems quite significant to shed more light on these tectonically active structures, particularly in terms of their potentiality to cause a probable seismic hazard to the study region. In other words, the consequences of our radon survey showed additional evidences of possible spatial fault-associated radon anomalies, and thereby verify the usefulness of using radon method as a reliable approach for characterizing some buried deformed structures and active faults in similar geologic environments elsewhere. In fact, the variability of soil gas radon concentration with regard to the tectonic activity of faults is basically related to the constant effects of the concentrated stress along with accumulated strains which usually result in high crustal deformations and thereby increase the degree of rocks permeability along the fault rupture zone. Such geodynamic changes will in turn enhance the mechanism of upward soil gases flow, and so increase the emanation rate of radon through the fractured bedrocks up to the ground surface.
Lastly, according to our results, and those reported in some other literatures, that the main advantages of using radon measurement technique as an important mean in some geologic and tectonic studies, can be summarized as follows: (1) the relatively low-cost and simplicity of conducting radon measurements; (2) the method is direct and the results are instantly available; (3) radon is a natural element, so this will avoid the addition of any synthetic materials to the environment. On the other hand, attention should be paid to some disadvantages that may encounter the application of radon methods, these include: (1) the variability of radon gas due its sensitivity to the ambient environmental conditions; (2) the effect of geology and the composition of the prevailing rocks at the measurement site; (3) and the relatively short half-life of radon, so it might be dispersed sometimes before reaching the ground surface. Thus, for better results and high accurate performance, it is essential to maintain the radon instrument calibrated before measurements start. Also it is recommended to carry out multiple radon samplings at the same measurement site as this would sufficiently minimize the possible occurrence of errors.
Conclusions
Systematic soil radon measurements have been performed across the Qastoon and the Al-Harif faults which represent two active tectonic segments of the northern Dead Sea Fault in northwestern Syria. The radon investigations were mainly guided by the results drawn from previous geophysical, morphotectonic, archaeoseismic and palaeoseismic surveys which were achieved in the same area and attested to the tectonic activity of the concerned fault structures. The overall results revealed the presence of a clear fault-associated radon anomaly with about six to seven fold increase of radon concentration, trending in accordance with the traces of the surface fault rupture at the Qastoon and Al-Harif, respectively. One significant result of this study is the observation of some anomalous radon increases that are remarkably corresponded with a gap located between two pieces of a faulted ancient Roman aqueduct at Al Harif site. The aqueduct was fractured and repeatedly displaced leftlaterally by a total horizontal offset of 13.6±0.2 m due to successive fault movements of three seismic events occurred along the Dead Sea Fault. Another point of interest is the notable occurrences of some anomalous radon emissions that are spatially consistent with the general trend of the Qastoon rupture fault zone which was detected and outlined by previous integrated geophysical and morphotectonic surveys. Ultimately, the aforesaid results could represent additional evidences regarding the possibility of using radon as a reliable and sensitive indicator for characterizing fault structures, and providing an insight into the significant role of local active tectonics in enhancing the level of soil radon emission.