INTRODUCTION
The prevalence of childhood obesity continues to grow worldwide1. Increased sugar consumption is considered to be a contributor to the global epidemics of obesity and diabetes and their associated cardiometabolic risks2. Overconsumption of added sugar is associated with obesity, non-alcoholic fatty liver disease (NAFLD), and insulin resistance (IR)3-5. Some of these associations may be the result of hepatic de novo lipogenesis stimulation by dietary sugars6,7. Among added sugars, fructose is emerging as a potentially harmful component7,8. The intake of large amounts of fructose creates an unregulated source of carbon precursors for hepatic lipogenesis9, which contributes to the development of NAFLD10 and hepatic IR9,11. Hepatic IR is characterized by an inability of insulin to suppress glycogenesis and glycogenolysis, and it is closely associated with a dysfunction of the adipose tissue12. The liver has been shown to be the main site of production of the insulin-like growth factor binding protein-1 (IGFBP-1) in humans, which has been proposed as a hepatic insulin-resistance marker13,14. IGFBP-1 is unique among other hepatic insulin-resistance markers because it is regulated by insulin and predicts glucose tolerance worsening; moreover, it is less labile than insulin13,15. Since IGFBP-1 is inversely correlated with liver fat, it is independent of obesity. Hepatic fat accumulation is tightly linked to hepatic IR and is characterized by a decreased ability of insulin to suppress hepatic glucose and triglyceride (TG)-rich, very-low-density-lipoprotein particle production in the liver14. On the other hand, one of the weaknesses of IGFBP-1 is that its reproducibility in serum, such as other indicators of IR, has been reported to decline with deteriorating glucose tolerance13. Previous research has shown that IGFPB-1 promotes the regeneration of β cells16 and that high levels of IGFBP-1 are associated with a reduction in diabetes risk15,17. Low serum IGFPB-1 levels have also been observed in obesity, hyperinsulinemic conditions18,19, and NAFLD conditions20. A previous study in children with obesity in our group showed that 73.1% had a high homeostatic model assessment of IR (HOMA-IR) and low IGFBP-1 levels, which could be an indication of whole-body IR and hepatic IR21.
Few studies have been conducted in children, and to the best of our knowledge, there are no studies evaluating hepatic IR, NAFLD, and fructose consumption in children with obesity. Therefore, the aim of this study was to evaluate IGFBP-1 (used as a marker of hepatic IR), NAFLD and their relationship with fructose consumption in children with obesity.
METHODS
Study design and population
A cross-sectional study was performed in children with obesity. Children 6-11 years old and attending schools in the city of Leon, Mexico, were invited to participate in the study. A total of 83 children with obesity, clinically healthy and without nutritional treatment were included. A sample size was calculated according to the correlation between IGFBP-1 and body mass index (BMI) found by Reinehr et al.22, considering a power of 80% and an alpha of two sides of 0.01, obtaining a sample size of 68 participants. However, it was decided to increase the sample size by 20%, and 83 participants were included.
Anthropometric measures such as weight, height, and waist circumference were taken using a Tanita HD-357 scale, a Seca 406 stadiometer, and a Lufkin measuring tape23. The weight and height were used to calculate BMI (weight/height2). Children were considered as having obesity if their BMI after adjustment for gender and age, were higher than the adult equivalent of 30 kg/m2, according to the international tables of Cole et al.24. Blood pressure was measured using a mercury sphygmomanometer according to the National High Blood Pressure Education Program Working Group25.
Biochemical measurements
A venous blood sample was obtained after 12 h of fasting to measure glucose by an enzymatic method (Lakeside, Mexico City, Mexico). TGs and cholesterol were also measured by the enzymatic method (Spinreact, Girona, Spain). Serum aliquots were stored at −80°C until the further determination of IGFPB-1, measured by enzyme-linked immunosorbent assay (ELISA, Mediagnost, Reutlingen, Germany), and insulin, by radioimmunoassay (CisBio®). For IGFPB-1, only 68 samples were processed due to insufficient serum.
The intra- and inter-assay variation coefficients for IGFPB-1 were 6.2% and 7.4%, and for insulin, they were 2.7% and 5.8%, respectively. There are no studies defining cutoff values for IGFPB-1; therefore, for this study, a low IGFBP-1 level was considered as ≤ 6.67 ng/mL and a high level was > 6.67 ng/mL, according to our previous work21.
Whole IR was calculated with HOMA-IR according to Matthews et al.26, and children were classified as having IR according to cutoff values from previous work in Mexican population27. The TG/high-density lipoprotein cholesterol index (I-TG/HDL-C) was calculated, which is an indirect method for IR proposed by Quijada et al.28, and values > 2.0 were considered as a cardiovascular risk factor29.
Nutritional assessment
Each child completed a 24-h dietary recall (2 during the week and 1 on the weekend) with the help from their parents. Quantification of macronutrients (proteins, lipids, and carbohydrates) was carried out using the software Food Processor 2015®. The total fructose intake was calculated according to the fructose of foods, called free fructose, and the fructose released from sucrose during digestion, using the formula (total fructose = free fructose [g/day] + 1/2 free sucrose [g/day])30. Additional analysis by food groups rich in fructose was carried out to detect which one had a major contribution to total fructose intake. The food groups analyzed were five: vegetables, fruit, 100% fruit juice, sugar-sweetened foods, and sugar-sweetened beverages30. Once the results were obtained, the average consumption was calculated from the three dietary recalls.
Ultrasonographic evaluation of NAFLD
NAFLD was assessed by ultrasound using a General Electric Logic 400 MD (General Electric, Boston, MA, USA) with a convex transducer of 3.6 MHz. The ultrasonographic evaluation was done in a blinded manner by two experienced radiologists, and in the event of contradictory results, a third evaluation was requested from another radiologist. Different degrees of fatty infiltration were considered: (a) light, when an increase was observed in echogenicity and hepatomegaly; (b) moderate, when sound attenuation was added; and (c) severe, when the walls of portal vessels and diaphragm were not visible31. Finally, the intra-operator reliability of NAFLD measurements was excellent for three operators, with intraclass correlation coefficient (ICC) of 0.88 (95% confidence interval [CI]: 0.90-0.87), and inter-operator reliability was good, with ICC of 0.74 (95% CI: 0.78-0.70).
Ethical considerations
The study was approved by the Institutional Committee for Bioethics in Research of the University of Guanajuato (CIBIUG) (CIBIUG-P03-2016), and if both the child and the parent or tutor agreed to participate, they signed informed consent.
Statistical analysis
Descriptive statistics for data with a normal distribution are presented as mean and standard deviation and the data without normal distribution as median and ranges. A Students t-test or a MannWhitney U-test was used for the difference between groups. For the differences between the degrees of NAFLD, an analysis of variance or KruskalWallis test with a post hoc Tukey test was performed, and to carry out the statistical analysis according to the degree of hepatic steatosis, the children with moderate and severe NAFLD were grouped together, since the latter group only had nine children. A Spearman correlation test was used to determine the association between variables. In addition, a multiple regression analysis using the IGFBP-1 as a dependent variable and the significant variables from the correlation analysis as regressors were used. Listwise deletion of missing data was used for the multiple regression analysis. With respect to the ultrasonographic diagnosis of NAFLD, inter- and intra-observer variability was calculated using an ICC. The software Statistica 7.0 for Windows (StatSoft, Tucson, AZ) was used for all the analyses, and a value of p < 0.05 was considered as significant.
RESULTS
A total of 83 children were studied with a mean age of 9.2 ± 1.3 years; 48.2% were male and 51.8% were female. About 93% of the girls presented IR, and 72.1% had a TG/HDL-C index > 2. The main contributor to fructose intake was the sugar-sweetened beverages group, with 42.75 ± 19.72 g/day; it was followed by the sugar-sweetened foods group, with 14.56 ± 9.39 g/day; the fruit group, with 4.69 ± 5.52 g/day; and finally, the vegetables and natural juices groups, with < 1.0 g/day.
Table 1 shows a comparison by gender for general and metabolic characteristics; the girls showed greater waist circumference (p = 0.002), TGs (p = 0.009), VLDL (p = 0.01), HOMA-IR (p = 0.00001), and TG/HDL-C index (p = 0.012), and they had lower IGFBP-1 levels (p = 0.0001). No significant differences were found in fructose intake from the food groups analyzed (results not shown).
Table 1 Comparison of variables between the female and male gender
Variable | Gender | Value t/Z* | p | |
---|---|---|---|---|
Male (n = 40) | Female (n = 43) | |||
Age (years)* | 9 (6-11) | 9 (6-11) | 0.59 | 0.55 |
BMI (Kg/m2) | 26.2 ± 2.5 | 26.9 ± 2.5 | 1.28 | 0.20 |
Waist circumference (cm) | 84.9 ± 7.2 | 90.0 ± 7.4 | 3.16 | 0.002 |
Systolic blood pressure (mmHg)* | 95 (80-115) | 90 (80-120) | 0.11 | 0.91 |
Diastolic blood pressure (mmHg)* | 60 (50-80) | 60 (50-85) | 0.73 | 0.47 |
Metabolic Variables | ||||
Triglycerides (mmol/L) | 1.44 ± 0.47 | 1.77 ± 0.64 | 2.7 | 0.009 |
Total-Cholesterol (mmol/L) | 4.17 ± 0.66 | 4.37 ± 0.70 | 1.29 | 0.20 |
HDL-C (mmol/L) | 1.46 ± 0.21 | 1.44 ± 0.19 | −0.56 | 0.58 |
VLDL (mmol/L) | 0.65 ± 0.22 | 0.8 ± 0.3 | 2.58 | 0.01 |
LDL (mmol/L) | 2.0 ± 0.53 | 2.12 ± 0.67 | 0.89 | 0.37 |
Glucose (mmol/L) | 4.97 ± 0.52 | 4.94 ± 0.50 | −0.28 | 0.78 |
Insulin (µIU/mL)* | 1.55 (0.60-5.04) | 2.41 (0.81-5.01) | 4.65 | 0.00001 |
HOMA-IR* | 2.3 (0.9-8.2) | 3.7 (1.1-8.5) | 4.46 | 0.00001 |
IGFBP-1 (ng/ml)* | 3.71 (0.16-23.9) | 0.91 (0.06-8.81) | −3.82 | 0.0001 |
Triglyceride/HDL-C index | 2.3 ± 0.83 | 2.9 ± 1.2 | 2.57 | 0.012 |
Dietetic Variables | ||||
Energy (Kcal/day) | 2315.6 ± 539.0 | 2174.5 ± 479.1 | −1.26 | 0.21 |
Protein (g/day) | 82.3 ± 20.2 | 78.0 ± 16.9 | −1.03 | 0.30 |
Lipids (g/day) | 86.2 ± 25.6 | 74.1 ± 20.4 | −2.39 | 0.019 |
Carbohydrates (g/day) | 310.7 ± 71.3 | 306.6 ± 77.1 | −0.25 | 0.80 |
Total fructose (g/day) | 61.8 ± 19.9 | 65.5 ± 21.9 | 0.82 | 0.41 |
Fructose Density (g/Kcal) | 0.027 ± 0.007 | 0.03 ± 0.006 | −2.07 | 0.042 |
The variables with normal distribution are presented as mean ± SD and the variables that did not follow normal distribution
*were reported as median (range). BMI: body mass index, HOMA-IR: homeostatic model assessment of insulin resistance, IGFBP-1: insulin-like growth factor binding protein-1, HDL-C: high-density lipoprotein cholesterol, VLDL: very low-density lipoprotein.
Table 2 shows the comparison of metabolic variables according to low or high IGFBP-1 levels. The group with low IGFPB-1 was older (p = 0.008) and had higher BMI (p = 0.00002) and waist circumference (p < 0.0001), as well as higher HOMA-IR (p < 0.0001). In relation to dietary variables, a higher energy consumption (p = 0.012), carbohydrates (p = 0.051) and total fructose intake (p = 0.022) were found in the group with low IGFPB-1. No significant differences were found in fructose intake from the food groups analyzed (results not shown).
Table 2 Comparison of variables according to the low or high serum level of IGFBP-1
Variable | IGFBP-1 | Value t/Z* | p | |
---|---|---|---|---|
Low (<6.66) (n = 51) | High (≥6.67) (n = 17) | |||
Age (years)* | 10 (6-11) | 8.0 (6-11) | 2.66 | 0.008 |
BMI (Kg/m2) | 27.2 ± 2.4 | 24.3 ± 1.5 | 4.62 | 0.00002 |
Waist circumference (cm) | 89.6 ± 6.9 | 80.4 ± 4.8 | 5.09 | 0.000003 |
Systolic blood pressure (mmHg)* | 95 (80-120) | 90.0 (80-105) | 1.52 | 0.12 |
Diastolic blood pressure (mmHg)* | 60.0 (50-85) | 60.0 (50-80) | 1.49 | 0.13 |
Metabolic variables | ||||
Triglycerides (mmol/L) | 1.63 ± 0.65 | 1.48 ± 0.53 | 0.86 | 0.39 |
Total-Cholesterol (mmol/L) | 4.27 ± 0.72 | 4.32 ± 0.56 | -0.27 | 0.79 |
HDL-C (mmol/L) | 1.45 ± 0.21 | 1.45 ± 0.21 | -0.11 | 0.90 |
VLDL (mmol/L) | 0.73 ± 0.3 | 0.67 ± 0.24 | 0.86 | 0.39 |
LDL (mmol/L) | 2.08 ± 0.57 | 2.02 ± 0.55 | -0.36 | 0.72 |
Glucose (mmol/L) | 4.96 ± 0.48 | 4.92 ± 0.49 | 0.22 | 0.82 |
Insulin (µIU/mL) * | 2.06 (0.94-5.01) | 0.99 (0.6-2.06) | 4.95 | 0.000001 |
HOMA-IR * | 3.0 (1.1-8.4) | 1.5 (0.9-3.0) | 4.77 | 0.000002 |
Triglyceride/HDL-C index | 2.6 ± 1.2 | 2.4 ± 0.95 | 0.80 | 0.43 |
Dietetic variables | ||||
Energy (Kcal/day) | 2321.4 ± 508.1 | 1963.1 ± 452.2 | 2.58 | 0.012 |
Protein (g/day) | 80.6 ± 18.5 | 73.3 ± 17.5 | 1.42 | 0.16 |
Lipids (g/day) | 82.5 ± 23.4 | 70.7 ± 21.6 | 1.84 | 0.07 |
Carbohydrates (g/day) | 322.6 ± 75.8 | 264.3 ± 58.5 | 2.89 | 0.0051 |
Total fructose (g/day) | 67.5 ± 23.1 | 53.4 ± 15.4 | 2.34 | 0.022 |
Fructose Density (g/Kcal) | 0.029 ± 0.006 | 0.028 ± 0.008 | 0.47 | 0.641 |
The variables with normal distribution are presented as mean ± SD and the variables that did not follow normal distribution
*were reported as median (range). BMI: body mass index, HOMA-IR: homeostatic model assessment of insulin resistance, IGFBP-1: insulin-like growth factor binding protein-1, HDL-C: high-density lipoprotein cholesterol, VLDL: very low-density lipoprotein, LDL: low-density lipoprotein.
In the total group, 81% had NAFLD in its different levels (light 34% and moderate/severe 47%). The group with moderate/severe NAFLD had higher BMI (p = 0.009), waist circumference (p = 0.046), insulin (p = 0.022), HOMA-IR (p = 0.036), carbohydrates intake (p = 0.005), total fructose intake (p = 0.001) (Fig. 1), and higher fructose intake from sugar sweetened beverages (p = 0.007). In this group, we also found lower levels of IGFBP-1, but these were not statistically significant (Table 3).
Table 3 Comparison of variables according to the NAFLD degree
Variable | Normal (n = 16) | Mild (n = 28) | Moderate/Severe (n = 39) | F/H* | p |
---|---|---|---|---|---|
Age (years)* | 8.5 (6-11) | 9.0 (6-11) | 10 (7-11) | 3.7 | 0.15 |
BMI (Kg/m2) | 25.7 ± 1.9 | 25.9 ± 2.5 | 27.4 ± 2.4 | 4.1 | 0.009a,b |
Waist circumference (cm) | 84.4 ± 6.9 | 86.2 ± 7.8 | 89.9 ± 7.3 | 2.8 | 0.046c |
Systolic blood pressure (mmHg)* | 90 (80-110) | 90 (80-110) | 90 (85-120) | 3.8 | 0.49 |
Diastolic blood pressure (mmHg)* | 60 (50-75) | 60 (50-75) | 60 (50-85) | 4.6 | 0.09 |
Triglycerides (mmol/L) | 1.49 ± 0.61 | 1.64 ± 0.72 | 1.64 ± 0.47 | 1.1 | 0.35 |
Total-cholesterol (mmol/L) | 3.99 ± 0.50 | 4.32 ± 0.63 | 4.35 ± 0.77 | 1.9 | 0.12 |
HDL-C (mmol/L) | 1.36 ± 0.22 | 1.46 ± 0.19 | 1.47 ± 0.20 | 1.6 | 0.19 |
VLDL (mmol/L) | 0.67 ± 0.28 | 0.75 ± 0.33 | 0.75 ± 0.22 | 0.41 | 0.66 |
LDL (mmol/L) | 1.79 ± 0.5 | 2.1 ± 0.56 | 2.14 ± 0.66 | 2.07 | 0.13 |
Glucose (mmol/L) | 4.87 ± 0.52 | 5.00 ± 0.48 | 4.96 ± 0.53 | 0.5 | 0.68 |
Insulin (µIU/mL)* | 1.28 (0.60-5.01) | 1.86 (0.76-4.32) | 2.03 (0.81-5.04) | 7.7 | 0.022 |
HOMA-IR* | 1.9 (0.90-7.8) | 2.9 (1.20-6.8) | 3.0 (1.2-8.5) | 6.6 | 0.036d |
IGFBP-1 (ng/ml)* | 2.6 (0.16-18.6) | 2.5 (0.6-15.7) | 1.5 (0.11-23.9) | 1.63 | 0.44 |
Triglyceride/HDL-C index | 2.6 ± 1.2 | 2.6 ± 1.2 | 2.6 ± 0.9 | 0.5 | 0.67 |
Energy (Kcal/day) | 2110 ± 424 | 2077 ± 452 | 2416 ± 537 | 3.9 | 0.012e |
Protein (g/day) | 78 ± 20 | 76 ± 16 | 84 ± 19 | 1.9 | 0.12 |
Lipids (g/day) | 72 ± 20 | 76 ± 20 | 87 ± 26 | 2.1 | 0.10 |
Carbohydrates (g/day) | 297 ± 74 | 281 ± 70 | 333 ± 70 | 4.7 | 0.005f |
Total fructose (g/day) | 58 ± 16 | 54 ± 16 | 73 ± 22 | 5.7 | 0.0014g |
Fructose Density (g/Kcal) | 0.028 ± 0.006 | 0.026 ± 0.007 | 0.031 ± 0.007 | 2.4 | 0.07 |
Food groups | |||||
Vegetables (g/day) | 0.78 (0-5.23) | 0.37 (0-1.89) | 0.54 (0-1.74) | 1.4 | 0.16 |
Fruit (g/day) | 5.13 (0-34.8) | 5.41 (0-17.4) | 6.67 (0-27.1) | 0.3 | 0.76 |
100% fruit juices (g/day) | 1.16 (0-10.93) | 1.78 (0-21.87) | 1.28 (0-32.8) | −0.8 | 0.45 |
Sugar sweetened beverages (g/day) | 43.5 ± 29.8 | 38.1 ± 16.5 | 54.9 ± 20.5 | 5.32 | 0.007h |
Sugar sweetened foods (g/day) | 26.2 ± 18.2 | 24.7 ± 18.9 | 26.6 ± 17.0 | 0.09 | 0.91 |
The variables with normal distribution are presented as mean ± SD and the variables that did not follow normal distribution
*were reported as median (ranges). Non-alcoholic fatty liver disease (NAFLD). Significantly differences
a:None versus moderate and severe, p < 0.041,
b:Mild versus moderate and severe, p < 0.032,
c:None versus moderate and severe, p < 0.038,
d:None versus moderate and severe, p < 0.029,
e:Mild versus moderate and severe, p < 0.02,
f:Slight versus moderate and severe, p < 0.009,
g:None versus moderate and severe, p < 0.031,
h:Slight versus moderate and severe, p < 0.006. BMI: body mass index, HOMA-IR: homeostatic model assessment of insulin resistance, IGFBP-1: insulin-like growth factor binding protein-1, HDL-C: high-density lipoprotein cholesterol, VLDL: very low-density lipoprotein, LDL: low-density lipoprotein.
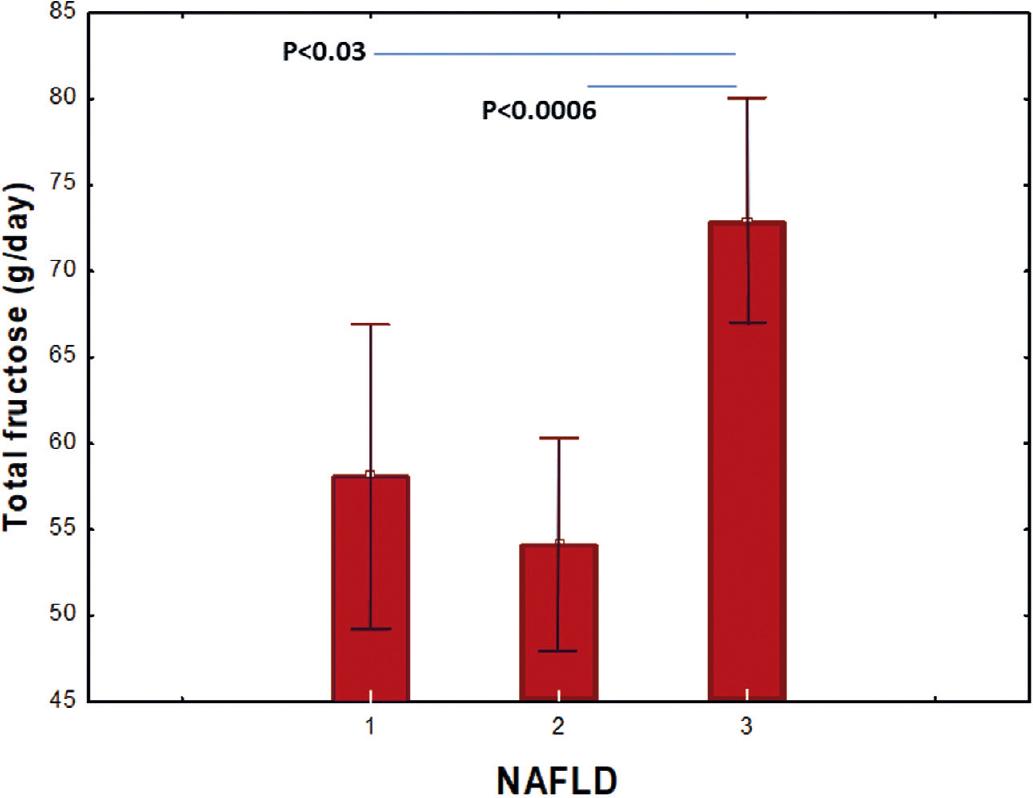
Figure 1 Comparison of fructose consumption according to the non-alcoholic fatty liver disease (NAFLD) degree. (1): none; (2): light; (3): moderate and severe NAFLD. NAFLD: non-alcoholic fatty liver disease.
Negative correlations were found between IGFBP-1 and BMI (r = −0.42; p = 0.02), HOMA-IR (r = −0.61; p = 0.002), and total fructose intake (r= −0.25; p = 0.03).
The multiple regression analysis is presented in Table 4: IGFBP-1 was found to be associated with BMI, HOMA-IR, and total fructose intake (R2 = 0.41; F = 10.75 p < 0.0001), and this association persisted after adjustment by gender (R2 = 0.11; F = 10.75 p < 0.0001).
Table 4 Association of the IGFBP-1 serum levels
Dependent variable | Independent variables | Beta ± SE | t | p |
---|---|---|---|---|
R = 0.64; R2 = 0.41; F = 10.75; p < 0.00001 | ||||
IGFBP-1 (n = 68) | BMI | −0.57 ± 0.22 | −2.55 | 0.013 |
HOMA-IR | −0.67 ± 0.33 | −2.02 | 0.047 | |
Fructose consumption | −0.05 ± 0.02 | −2.05 | 0.044 | |
Gender | −2.9±1.12 | −2.60 | 0.012 |
BMI: body mass index, HOMA-IR: homeostatic model assessment of insulin resistance, IGFBP-1: insulin-like growth factor binding protein-1.
DISCUSSION
In Mexico, obesity is an important public health problem. The National Health and Nutrition Survey 2016 reported that the combined prevalence of overweight and obesity in children between 5 and 11 years was 33.2%. The obesity prevalence in girls was 12.2% and 18% in boys. In the past decades, high fructose intake has been associated with increased risk of obesity development and the consequent metabolic and inflammatory diseases3,32. Liver function is particularly vulnerable to an increasing fructose intake since this organ is responsible for approximately 90% of the total metabolism of this sugar33. The liver has also been found to be the main site of production of IGFBP-1 in humans, a marker of hepatic IR. However, few studies related to these markers have been conducted in children and none, to the best of our knowledge that evaluates hepatic IR, NAFLD, and fructose consumption in children with obesity. In this study, we evaluated school-age children and found that in the group with low levels of IGFBP-1, the children showed higher levels of IR and HOMA-IR, and IGFBP-1 showed a negative association with HOMA-IR. These results suggest that higher hepatic IR is present with higher whole IR. Similar results have been found by other authors in elderly men18 and nondiabetic patients on peritoneal dialysis34. An explanation for these results may be found in recent studies that proposed that IGFBP-1 promotes the regeneration of β-cells15, re-establishing the pancreatic function while the total IR persists or worsens. Reports from animal studies indicate that obesity is associated with the suppression of the hepatic expression of mRNA of IGFBP-1 and a marked reduction of total concentrations of circulating IGFBP-135.
In our study, the group with lower levels of IGFBP-1 had a higher consumption of carbohydrates and total fructose, and in the multivariate analysis, IGFBP-1 showed a negative association with fructose consumption. In this sense, the liver is the primary site of de novo lipogenesis, the process by which fatty acids are synthesized from dietary precursors, predominantly carbohydrates36. Excessive fructose consumption may also have significant effects on lipid metabolism, contributing both to steatosis and to increased circulating TG levels by means of the inhibition of the enzyme carnitine palmitoyltransferase 1A2. Changes in IGFBPs have been correlated with some metabolic syndrome (Mets) components, and predominantly with obesity37,38. Reinehr et al.22 found that IGFBP-1 correlated significantly with most of the Mets components (waist circumference, TGs, and HOMA-IR). Similar results were found in our group of children with obesity.
In our study group, 81% of the children showed NAFLD at levels (light 34%, and moderate/severe 47%) higher than those found in previous studies in our group (42.5%)21. These children also had higher BMI and larger waist circumference; similar results were found in children from the general population and studies based on child obesity clinics, although the prevalence was lower39. The children with moderate/severe NAFLD showed higher HOMA-IR, which could be explained by reports that hyperinsulinemia leads to de novo lipogenesis, which is partially responsible for the accumulation of TGs in the hepatocytes and the development of steatosis40. However, the mechanisms by which high-fructose feeding causes hyperinsulinemia and IR remain uncertain2. Fructose-induced steatosis may contribute to hepatic IR through increased hepatic diacylglycerol accumulation, protein kinase C activation, and impairment of insulin-mediated Akt2 activation41,42. However, whether steatosis itself can cause hepatic IR remains controversial43,44. In our study, higher consumption of carbohydrates and fructose observed in the group with moderate/severe NAFLD was similar to other studies6,10,45. High carbohydrates intake exceeding the energetic requirements and above the liver storage capacity in the form of glycogen, are converted into fatty acids to be stored as triacylglycerol, leading to NAFLD46. On the other hand, a meta-analysis indicated that consumption of fructose has more damaging effects on the hepatic insulin sensitivity than does the isocaloric consumption of carbohydrates4.
Likewise, Gugliucci47 state that approximately 90% of fructose intake is metabolized in the liver, while a large part of the glucose consumed passes through the liver and finds its way to skeletal muscle, where it degrades to CO2, H2O, and adenosine triphosphate, and to the adipose tissue, where it is converted into phosphate glycerol for the synthesis of TGs and production of energy. Therefore, it is undeniable that in this multi-factorial pathology of NAFLD, elevated consumption of carbohydrates, especially fructose, is a major risk factor for its development.
A previous study in children by our group showed that the restriction of high-fructose foods with a decrease in caloric and carbohydrate intake, at 6 weeks induced a decrease of TG levels and hepatic steatosis48. In the present study, the group of children without steatosis and the group with mild steatosis had similar fructose intake; besides, they had similar metabolic and anthropometric characteristics. An explanation to these similarities could be that ultrasound is not sensitive enough to detect mild states of steatosis49.
It is important to highlight that our results show that girls have higher levels of TGs, HOMA-IR, I-TG/HDL, and lower IGFBP-1; all of them considered cardiovascular risk factors. A possible explanation is that girls had higher fructose consumption, which contributed to the development of hepatic IR, total IR, and higher TGs which could put them at higher risk of developing cardiometabolic diseases in early adulthood; thus, it is important to evaluate all of these risk factors from an early age.
This study had several weaknesses. First, the small sample size and that only 68 samples of IGFBP-1 were processed due to insufficient serum; also, the transversal nature of the study which did not allow us to evaluate causality; and finally, the use of ultrasound as a diagnostic tool for steatosis. Ultrasound detects fatty liver disease in patients with moderate and high-fat content but is limited in patients with low-fat content49. Furthermore, conventional ultrasound signs may be operator-dependent and subjective, although the intra- and inter-observer agreement in our study was good to excellent.
The group with lower levels of IGFBP-1 (marker of hepatic IR) was associated with BMI, HOMA-IR, and fructose consumption after adjustment by gender; the group with higher severity of NAFLD showed higher HOMA-IR and fructose consumption, and the metabolic alterations were more evident in females (higher TGs, HOMA-IR, and lower IGFBP-1). These results support the importance to evaluate children to prevent the development of chronic diseases.