Introduction
The house fly (Musca domestica) is a ubiquitous pest of animal agriculture and public health because it is a vector of many diseases of humans and other animals (Khan, 2018). This insect is responsible for the mechanical transmission of pathogens (including parasites, bacteria, fungi, and viruses), which occurs when pathogens are transmitted from one host to another without amplification or development of the pathogen within the vector (Issa, 2019; Khamesipour et al., 2018), principally through contamination of the fly's external body surface (Park et al., 2019). This should be considered when designing effective management plans according to local conditions that may differ between geographical regions and environments and to prevent zoonotic transmission (Liu et al., 2023; Park et al., 2019). The house fly is present in most animal production facilities, where the fly develops in feces and decaying organic matter (Park et al., 2019). This pest is difficult to manage due to its high fecundity, short development time, and propensity for developing resistance to insecticides (Khater & Geden, 2019). Chemical control using synthetic insecticides is the primary strategy for controlling house flies. However, adverse effects have accompanied the misuse of these chemicals on human health and the environment, and the development of pesticide resistance (Soyelu et al., 2020). In this scenario, there is a need to develop novel insecticides of a biological nature to achieve adequate control with ecotoxicologically acceptable characteristics (Chintalchere et al., 2021). Moreover, there are circumstances and environments in which conventional insecticides cannot be used due to their toxicity toward mammals and other non-target organisms (Khan, 2018). Essential oils are natural alternatives to complement commercial synthetic insecticides and repellents since they contain toxic components to insects but are notably less harmful to mammals (Alavez-Rosas et al., 2022; Isman, 2020).
Essential oils are mixtures of plant secondary metabolites, primarily mono- and sesquiterpenes, their oxygenated derivatives, and other minor compounds (Isman, 2020). The importance of these plant-derived products relies on their high bioactivity due to their chemical composition; they can be cheap and are also biodegradable, effective, and selective compared to their synthetic counterparts (Alavez-Rosas et al., 2022; Isman, 2020). Several studies have revealed the larvicidal, ovicidal, adulticidal, repellence, and ovipositional deterrence effects of essential oils (Chauhan et al., 2018; Isman, 2020; Subaharan et al., 2021). Studies have focused on the bioactivity of individual essential oils, attributing the strength of essential oils to the combination of their different chemical constituents (Isman, 2020; Subaharan et al., 2021). Therefore, blends of essential oils can be valuable for protecting and controlling insect pests or disease vectors, as their mixture enriches the diversity of active compounds. For example, exposure to mint, Mentha arvensis Linnaeus, (Lamiales: Lamiaceae) and lavender, Lavandula angustifolia Miller, (Lamiales: Lamiaceae) essential oils resulted in high mortality to larval and adult houseflies (Khater & Geden, 2019; Soonwera, 2015).
There is a need to find environmentally and human-health-friendly alternatives to control M. domestica, and a blend of essential oils could be one such alternative. In the context of Integrated Pest Management (IPM), we are currently studying the control of M. domestica by developing semiochemicals-derived attractants to be used in traps to capture adults, as well as essential oil-based biopesticides or repellents for use in a “push-pull” system. In this study, we evaluated the insecticidal effect of a blend of essential oils reported as individually toxic to the house fly (Khan et al., 2017). Thus, we blended the commercial essential oils from citronella [Cymbopogon nardus Linnaeus, (Poales: Poaceae)], clove [Eugenia caryophyllata Thunb, (Myrtales: Myrtaceae)], lavender (L. angustifolia), mint (M. arvensis) and rosemary [Rosmarinus officinalis Linnaeus, (Lamiales: Lamiaceae)]. The blend was tested on larvae and adults of M. domestica. Additionally, we evaluated the impact of this blend on the pupation and adult emergence of houseflies. Finally, as the insecticidal activity of essential oils is attributed to their richness of molecules, we determined the chemical composition of the blend. To better characterize the chemical constituents, we identified the compounds present in the liquid blend and the volatiles released from it.
Materials and methods
Insects. Adult houseflies were collected by sweep-netting from a cow farm located in Tapachula (14.893 N; 92.273 W), Chiapas, Mexico. About 300 flies were collected and brought to the laboratory for rearing. The adults were kept in meshed plastic jars (30 × 30 × 30 cm) and fed on a diet of powdered milk mixed with wheat bran in a 1:1 ratio by weight. Water was provided in a separate Petri dish using soaked cotton. After mating, the larvae were reared on a medium of powdered milk and wheat bran 1:1 by weight with 65 ml of water. All the insects were maintained at 25 ( 2 °C, 60-70 % relative humidity (RH), and 12:12 h (L:D) photoperiod.
Chemicals and essential oils. Lambda-cyhalothrin was obtained as the suspension concentrate Karate Zeon 5SC® (Syngenta, Madrid, Spain; containing 5% active ingredient, a.i.), whereas solvents (HPLC grade) were obtained from Sigma Aldrich (Toluca, Mexico). Essential oils of citronella (C. nardus), lavender (L. angustifolia), mint (M. arvensis), rosemary (R. officinalis), and clove (E. caryophyllata) were obtained from Pharmakos Rambal (Tuxtla Gutiérrez, Chiapas, Mexico). All oils were reported to have > 99% purity and were used without further purification. Standard compounds used for the identification (> 95%): α-pinene, camphene, ß-pinene, p-cymene, limonene, eucalyptol, linalool, citronellal, camphor, citronellol, (E)-geraniol, bornyl acetate, eugenol, and n-hexadecane were obtained from Sigma Aldrich (Toluca, Mexico).
The blend of essential oils was performed by mixing the pure oils in equal volumes; this mixture was labeled as 100 % v/v. For the insecticide lambda-cyhalothrin, a commercial product Karate Zeon 5% w/v, was labelled as 100 % v/v. Then, serial dilutions were performed to obtain the 50, 25, 12.5, 6.3, 3.1, 1.6, 0.78, 0.39, 0.20, 0.01, 0.005, 0.025, 0.012, 0.006, 0.003 and 0.0015% (v/v) dissolutions for the blend and lambda-cyhalothrin (equivalent to 2.5% - 0.000075% of lambda-cyhalothrin a.i.). Dilutions were performed in hexane for the blended oils and in water for lambda-cyhalothrin.
Larvicidal assay. Bioassays were conducted to determine the effects of the blend of essential oils and lambda-cyhalothrin on fly larvae using previously described exposure methods (Burgess et al., 2020). Late third instars were treated (in the dorsal thorax) with 5μL of different concentrations [(100, 50, 25, 12.5, 6.3, 3.1, 1.6, 0.78, 0.39, 0.20, 0.01, 0.005, 0.025, 0.012, 0.006, 0.003 and 0.0015% (v/v)] of the essential oil blend or lambda-cyhalothrin. Hexane was used as the control for the essential oils blend, and distilled water was used as the control for the lambda-cyhalothrin. We used ten larvae in each replicate and performed four replicates per treatment. Treated larvae were placed in 4.5 cm diameter Petri dishes, with 1 g of artificial diet (described above). Petri dishes were closed and placed in an incubator at 27 ± 2 ºC. Dead larvae were counted after 24 h. Mortality was defined as the inability of a larva to right itself when placed on its dorsal side and unable to engage in directed locomotion if it was right-side-down.
Adulticidal assay. Procedures for this assay were as previously described (Khater & Geden, 2019) with modifications as follows: plastic bottles (diameter = 2.5 cm, height = 9 cm, capacity = 45 mL) were treated with 50μL of different concentrations of the essential oils blend or lambda-cyhalothrin [(100, 50, 25, 12.5, 6.3, 3.1, 1.6, 0.78, 0.39, 0.20, 0.01, 0.05, 0.025, 0.012, 0.006, 0.003 and 0.0015% (v/v)]. We used solvent as control: hexane for the blend and water for the insecticide. Bottles were rotated to facilitate an even distribution of the treatments over the inner surface of the bottles. After the evaporation of the solvent, ten adult flies of both sexes (2 to 5 days old) were placed in each bottle, and then the bottles were capped with fabric caps. Dead flies were counted 24 hours after placement in the bottles. The criterion for mortality was no movement of any of the housefly body parts 24 hours after exposure. Four replicates were used for each treatment and the control groups.
Impact of essential oils blend on pupation and adult emergence. To evaluate the impact on pupation and adult emergence parameters, we used the same exposed larvae for the larvicidal assays (see above). For pupation, we added 10 g of sawdust to each Petri dish. The dishes were held in the incubator at 27 ± 2 ºC until pupation and adult emergence. Then, the numbers of pupae and adults were counted. The experiment was replicated four times for each treatment.
Volatile collection. Due to adults' exposure to the volatiles from the blend in bioassays, we were interested in analyzing the blend's headspace and comparing the chemical composition of volatiles with the liquid blend.
For this, headspace volatiles were collected using two different methods. Both techniques possess strengths and weaknesses, and combining both collection methods improves the accuracy of chemical identification (Alborn et al., 2021).
1)Solid-phase microextraction (SPME) using a poly-dimethylsiloxane fibre (SUPELCO, Deisenhofen, Germany). A 1 mL volume of the essential oil blend was placed in a vial and covered with aluminum foil. An SPME fibre was then inserted into the vial through the foil and exposed to the lure volatiles. The exposure time was 12 hours. An identical volatile extraction chamber was used simultaneously to determine the chemical background (emitted by the chamber or in the surrounding air). We used the same SPME fibre for each replicate, four replicates in total.
2) Dynamic aeration of headspace volatiles from a vial containing 1 mL of the mixture, the vial was placed inside a glass aeration chamber (10 cm long × 8 cm i.d.). The volatiles were collected by airflow at 2.0 L/min, (previously purified by an activated charcoal filter). The volatiles were captured in a Tenax ® adsorbent (35-60 mesh, 100/50 mg; Merck, Darmstadt, Germany). The volatile collection lasted 12 h, after which the volatiles were eluted from the adsorbent with 1 mL of hexane (HPLC-grade), and then the extract was concentrated to 100 μL using a gentle N2 airstream. Extracts were stored in small glass vials (1 mL) at −20 °C until analysis, and four replicates were performed.
Chemical analysis. To identify the compounds, SPME samples, extracts from dynamic aeration, and the liquid blend of essential oils were analyzed on a GC-MS Shimadzu GC-2010 plus, Triple-Quadrupole TQ8040 (Texas). A DB-5MS non-polar capillary column (30 m by 0.25 mm I.D., Supelco, Bellafont, PA) was used. The injector was operated in split mode. Helium was used as the carrier gas at 1.0 mL/min. The oven temperature was programmed at 50°C for 2 min, then 15°C for 1 min to 280°C and held for 10 min. Ionization was by electron impact at 70 eV. The compounds were identified using retention indices (arithmetic and Kovats) and mass spectra that matched the NIST library. A comparison with pure standards was performed when available.
Statistical analysis. Data were analyzed using the statistical software R version 4.1.3 (R Development Core Team, 2023). We used a Kaplan-Meir survival curve analysis (α = 0.05) to assess the mortality effect of different concentrations of the blend and lambda-cyhalothrin on larvae and adults. The same analysis was used to evaluate the impact on the pupation and adult emergence of M. domestica. We performed the log-rank test to compare the survival of groups (lambda-cyhalothrin and essential oils blend), taking the whole follow-up period into account. For the chemical analysis of the blend, the areas of the peaks in each sample were transformed into percentages (each compound was divided by the sum of the area of all compounds in the corresponding chromatogram). Percentages were analyzed by principal component analysis (PCA). To evaluate the degree of similarity of the groups formed in the PCA, the Mahalanobis distances among data were measured and compared through a one-way analysis of variance (ANOVA), including the interaction as a factor and the distance as the response variable. Means of the distances were compared with the Tukey test (α = 0.05).
Results
Larvicidal contact/fumigant assay. A low larvicidal effect was observed for all concentrations of lambda-cyhalothrin, none of which reached 50% of mortality in the tested population. However, a larvicidal effect of the essential oil blend was observed at a concentration of 25 % v/v (LC50 = 25 % v/v; 95 % CI= 20.3 - 29.3 % v/v). Comparison of survival curves showed a significant difference between the tested treatments (χ 2 = 32.4, df = 1, P < 0.0001), the essential oil blend was more effective than lambda-cyhalothrin against larvae. No mortality was observed in the control group (Fig. 1A).
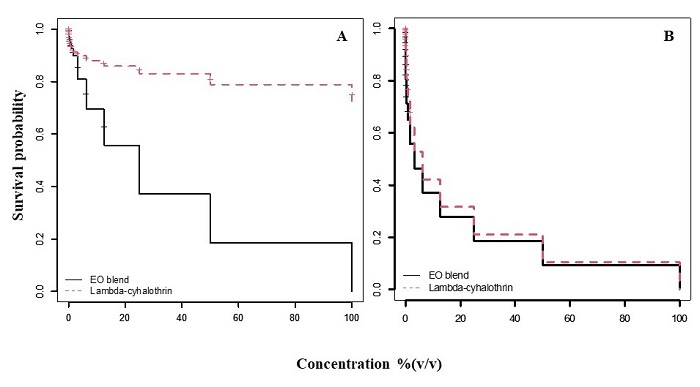
Figure 1 Kaplan-Meier survival curves for M. domestica over 17 different concentrations [(100, 50, 25, 12.5, 6.3, 3.1, 1.6, 0.78, 0.39, 0.20, 0.01, 0.005, 0.025, 0.012, 0.006, 0.003 and 0.0015% (v/v)] comparing the essential oils blend (continuous line) and lambda-cyhalothrin (dotted line) in larvae (A) and adults (B). The lambda-cyhalothrin product comprised 5% a.i.
Contact/fumigant toxicity for adults. Exposure to lambda-cyhalothrin (LC50 = 9.5 % v/v; CI= 8.3 - 10.2 % v/v; Fig 1B). and the essential oils blend (LC50 = 6.5 % v/v; CI= 6.4 - 7.6 % v/v) resulted in concentration dependent mortality in the tested insects. No mortality was recorded in the control insects. A lower concentration of the blend was needed to reach 50 % mortality compared to the commercial insecticide. However, comparison of survival curves showed no significant difference between the insecticide and the essential oil blend (χ 2 =2, df = 1, P = 0.16).
Impact of essential oils on pupation and adult emergence. After application of lambda-cyhalothrin and essential oils to M. domestica larvae, we recorded the number of developed pupae (Fig. 2A). Both treatments caused a similar reductive effect on the number of larvae that pupated (χ 2 = 1.4, df = 1, P = 0.23). Comparison of the survival curves also showed both treatments similarly affected the adult emergence (χ 2 = 3.1, df = 1, P = 0.08, Fig. 2B).
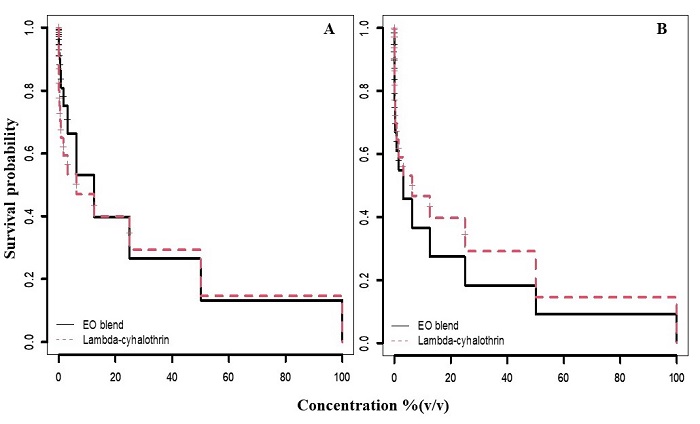
Figure 2 Kaplan-Meier survival curves for M. domestica pupae (A) and emerged adults (B) after application of treatments to larvae. Seventeen different concentrations were evaluated [(100, 50, 25, 12.5, 6.3, 3.1, 1.6, 0.78, 0.39, 0.20, 0.01, 0.005, 0.025, 0.012, 0.006, 0.003 and 0.0015% (v/v)] comparing the essential oils blend (continuous line) and lambda-cyhalothrin (dotted line). The lambda-cyhalothrin product comprised 5% a.i.
Chemical analysis. In the GC-MS analysis of the blend, we found 33 compounds (terpenes, terpenoids and one hydrocarbon, Table 1, Fig. 3). In the liquid blend, the most abundant compound was eugenol (mean ± SE: 13.29 ± 0.68 %), followed by eucalyptol (8.60 ± 0.60 %), linalool (6.76 ± 1.20 %), isoborneol (6.83 ± 1.57 %), linalyl acetate (6.13 ± 1.58 %) and citronellal (7.07 ± 0.16 %). In the dynamic aeration extract, the most abundant compounds were linalyl acetate (21.43 ± 0.34 %) and eugenol (19. 53 ± 0.40 %). For the volatiles captured via SPME, the most abundant compounds were α-pinene (22.78 ± 0.94 %), camphene (13.47 ± 1.40 %), β-pinene (14.79 ± 1.61 %) and eucalyptol (11.56 ± 2.04 %). For a better comparison of the three methods used, we performed a principal component analysis (PCA) with the percentages of compounds (Fig. 4), which explained 81.6 % of the total variance of the data. The plot of the first two components showed the formation of three different groups, corresponding to the liquid mixture and the volatiles of the dynamically aerated extract and the SPME sample (Fig. 4). The chemical similarity of the liquid extraction was higher with the dynamic aeration extraction than with the SPME extraction (based on a lower Mahalanobis distance). A more dissimilar chemical composition was observed between the dynamic aeration extraction method and SPME extraction, according to a higher Mahalanobis distance (Table 2).
Table 1 Chemical composition (percentage ± SE) of the essential oil blend and headspace volatiles analysed by two techniques.
Entry | LRI | KRI | ARI | Compound | Liquid blend | DA | SPME |
1 | 934 | 941 | 938 | α-pinene a,b | 3.21 ± 0.17 | 0.93 ± 0.03 | 22.78 ± 0.94 |
2 | 954 | 959 | 956 | Camphene a,b | 1.89 ± 0.36 | 0.34 ± 0.05 | 13.47 ± 1.40 |
3 | 989 | 986 | 984 | β-pinene a,b | 2.67 ± 0.19 | 0.67 ± 0.02 | 14.79 ± 1.61 |
4 | 1019 | 1024 | 1025 | p-Cymene a,b | 2.07 ± 0.94 | 0.39 ± 0.03 | 9.80 ± 2.40 |
5 | 1032 | 1037 | 1034 | Limonene a,b | 3.03 ± 0.31 | 1.37 ± 0.05 | 5.74 ± 1.41 |
6 | 1044 | 1042 | 1040 | Eucalyptol a,b | 8.60 ± 0.60 | 2.63 ± 0.77 | 11.56 ± 2.04 |
7 | 1109 | 1105 | 1105 | Linalool a,b | 6.76 ± 1.20 | 5.69 ± 0.72 | 1.18 ± 0.42 |
8 | 1151 | 1159 | 1157 | Citronellal a,b | 7.07 ± 0.16 | 6.42 ± 0.11 | 4.71 ± 0.50 |
9 | 1160 | 1162 | 1161 | Isopulegol a | 2.95 ± 0.25 | 2.75 ± 1.20 | 1.55 ± 0.12 |
10 | 1166 | 1165 | 1163 | Camphor a,b | 4.31 ± 0.23 | 2.35 ± 0.47 | 3.08 ± 0.37 |
11 | 1161 | 1169 | 1168 | Isomenthone a | 3.00 ± 0.13 | 2.07 ± 0.33 | 1.84 ± 0.30 |
12 | 1182 | 1179 | 1178 | Menthone a | 1.98 ± 0.23 | 1.05 ± 0.15 | 0.58 ± 0.14 |
13 | 1183 | 1180 | 1179 | Isoborneol a | 6.83 ± 1.57 | 4.63 ± 1.33 | 2.29 ± 0.51 |
14 | 1184 | 1182 | 1181 | Isomenthol a | 2.88 ± 1.07 | 2.66 ± 1.34 | 0.47 ± 0.08 |
15 | 1187 | 1188 | 1187 | Borneol a | 0.34 ± 0.04 | 0.35 ± 0.16 | 0.13 ± 0.02 |
16 | 1190 | 1190 | 1190 | Menthol a,b | 0.78 ± 0.10 | 0.45 ± 0.11 | 0.12 ± 0.04 |
17 | 1233 | 1234 | 1233 | Citronellol a,b | 3.73 ± 0.13 | 2.04 ± 0.52 | 0.41 ± 0.08 |
18 | 1255 | 1252 | 1251 | Linalyl acetate a | 6.13 ± 1.58 | 21.43 ± 0.34 | 0.22 ± 0.05 |
19 | 1259 | 1259 | 1258 | (E)-Geraniol a,b | 3.51 ± 0.18 | 2.58 ± 0.06 | 0.33 ± 0.06 |
20 | 1296 | 1296 | 1296 | Bornyl acetate a,b | 4.37 ± 1.13 | 4.01 ± 0.65 | 1.49 ± 0.22 |
21 | 1297 | 1297 | 1297 | Menthol acetate a | 0.71 ± 0.47 | 1.66 ± 1.12 | 0.15 ± 0.02 |
22 | 1355 | 1353 | 1352 | β-Citronellyl acetate a | 1.10 ± 0.11 | 1.82 ± 0.01 | 0.17 ± 0.02 |
23 | 1370 | 1370 | 1369 | Eugenol a,b | 13.29 ± 0.68 | 19.53 ± 0.40 | 1.38 ± 0.10 |
24 | 1384 | 1382 | 1382 | Geranyl acetate a | 0.88 ± 0.09 | 1.51 ± 0.04 | 0.11 ± 0.02 |
25 | 1409 | 1403 | 1403 | β-Ellemene a | 0.75 ± 0.08 | 1.32 ± 0.01 | 0.24 ± 0.03 |
26 | 1449 | 1443 | 1442 | Caryophyllene a | 3.43 ± 0.33 | 4.84 ± 0.05 | 0.87 ± 0.10 |
27 | 1480 | 1480 | 1480 | Humulene a | 0.78 ± 0.12 | 0.74 ± 0.03 | 0.14 ± 0.01 |
28 | --- | 1503 | 1503 | {204 [M]+, 161 (100); 105 (75); 91 (55); 119 (45)} | 0.15 ± 0.02 | 0.37 ± 0.02 | 0.04 ± 0.01 |
29 | --- | 1517 | 1517 | {204 [M]+, 161 (100); 105 (70); 91 (60); 119 (45)} | 0.48 ± 0.06 | 0.37 ± 0.04 | 0.03 ± 0.01 |
30 | --- | 1537 | 1536 | {204 [M]+, 105 (100); 161 (65); 94 (25)} | 0.26 ± 0.04 | 0.48 ± 0.01 | 0,04 ± 0.01 |
31 | 1568 | 1569 | 1568 | α-Elemol a | 0.94 ± 0.11 | 1.52 ± 0.04 | 0.18 ± 0.02 |
32 | 1600 | 1600 | 1600 | n-Hexadecane a,b | 0.75 ± 0.08 | 0.66 ± 0.03 | 0.07 ± 0.06 |
33 | 1612 | 1612 | 1612 | Caryophyllene oxide a | 0.41 ± 0.07 | 0.37 ± 0.04 | 0.03 ± 0.01 |
RT Retention Time, KRI Kovats Retention Index, ARI Arithmetic Retention Index, retention indices on DB-5 MS column, differences of ± 10 units between experimental and reference values were considered; LRI: Literature Retention Index, according to the NIST librarya (https://webbook.nist.gov/chemistry/name-ser/) and with the co-injection of synthetic standardsb.
DA Dynamic aeration, SPME solid-phase microextraction.
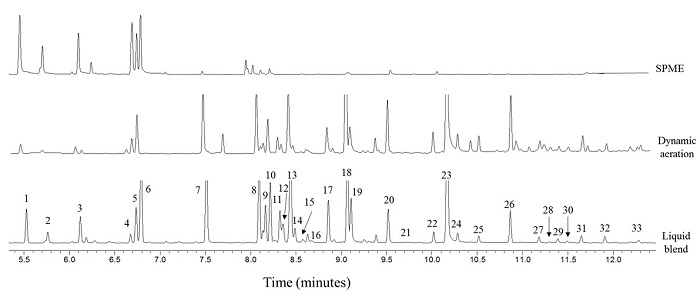
Figure 3 Representative chromatograms of liquid blend, dynamic aeration and SPME of blend headspace. Numbers correspond to those of table 1.
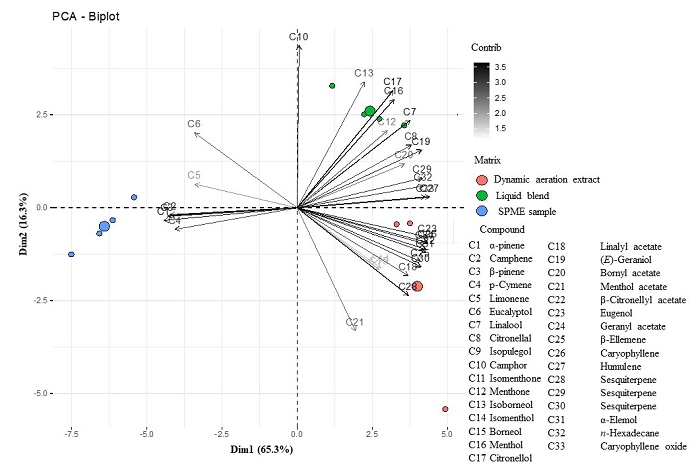
Figure 4 Principal component analysis of the chemical composition of the essential oil blend and its volatiles from two different extraction techniques
Table 2 Mahalanobis distances (mean ± SE) of the three groups formed in the PCA of the composition of the essential oil.
Interaction | Distance |
Liquid blend – Dynamic aeration extract | 877.2 ± 23.5c |
Dynamic aeration extract – SPME sample | 2750.2 ± 27.7a |
Liquid blend – SPME sample | 1985.5 ± 34.3b |
One-way analysis of variance: F2, 45 = 1067.3, P < 0.001. Means labelled with the same letter are not significantly different (Tukey’s test α = 0.05).
Discussion
We compared the insecticidal effect of a blend of five commercially available essential oils and the pyrethroid lambda-cyhalothrin against larvae and adults of M. domestica. The essential oils blend at higher concentrations had similar efficacy against adults and larvae as the pyrethroid. A further analysis revealed that the blend included 33 compounds: terpenes, terpenoids, and one hydrocarbon. The liquid blend and the volatiles evaporated from the blend presented a different ratio of compounds.
Individual essential oils from the blend have been adequate to control larvae and adults of the housefly, namely, lavender, Lavandula angustifolia (Khater & Geden, 2019), mint, Mentha spp. (Kumar et al., 2012), citronella, Cymbopogon nardus (Khan et al., 2017), and clove, Eugenia caryophyllata (Soonwera, 2015). Additionally, some blends of essential oils have been effectively used against larvae and adults in topical or fumigant assays (Said-Al Ahl et al., 2017). The LC50 of the blend presented here was comparable to those values reported in other studies performed with larvae and adults using individual essential oils (Benelli et al., 2018; Chintalchere et al., 2020; Rossi & Palacios, 2015; Tarelli et al., 2009). Apparently, larvae of the housefly were highly resistant to lambda-cyhalothrin despite this insecticide having been effectively used against M. domestica; this could be due to the lower concentrations we used, as dilutions were made from a commercially available insecticide comprising 5 % a.i. The extensive and injudicious use of pyrethroid insecticides has likely favored the development of resistance in this fly (Abbas et al., 2014). However, this insecticide prevented the development of insects. Meanwhile, the essential oil killed the larvae and could block the development of the insect. The blend of these five essential oils could be a potential tool for controlling M. domestica populations that have developed insecticide resistance and could be considered a promising biopesticide. However, this would require confirmation in field testing (Umetsu & Shirai, 2020). Integrated Vector Management (IVM) involves using a range of proven vector control methods, either alone or in combination (Gizaw et al., 2019) and this blend of essential oils could be tested in combination with the pesticides currently used for M. domestica control.
As the blend's biological activity is due to its chemical composition, in liquid form (larvicidal bioassays) or the contact + volatile exposure (bioassays with adults), it is crucial to determine which compounds and their proportions in the headspace of the blend. Thus, we examined the gaseous part of the blend by SPME and dynamic aeration using Tenax®. All compounds we found were previously reported as components of the essential oils we used (Alavez-Rosas et al., 2022; Guo & Wanf, 2020). Differences in the chemical composition of the blend volatiles could be attributed to limitations of SPME analysis for quantification ( Gaffke & Alborn, 2021; Romeo, 2009). Assuming that the headspace volatiles of the blend possess the same composition as the liquid blend, the dynamic aeration volatiles had the most accurate composition to the liquid blend. Dynamic aeration techniques provide higher collection efficiency and more reliability with the real composition of the blend (Alborn et al., 2021). In this scenario, adults were likely exposed to a similar proportion of the volatiles as the larvae. The insecticidal effect could be due to the combination of the most abundant terpenes and interactions among them, although these compounds would also be subjected to insect metabolic detoxification (Scalerandi et al., 2018). The most abundant compounds of the blend have been reported to be effective insecticides against pests. For example, linalool was effective for eliminating infestations of M. domestica (Beier et al., 2014; Senthoorraja et al., 2021). Eucalyptol was more effective against male than female flies (Sukontason et al., 2004). Eugenol, linalyl acetate, camphene, α- and β-pinene effectively killed larvae (Zhang et al., 2017). However, more studies are needed to determine the true composition of the headspace volatiles of the essential oil blend. Further studies are also needed to assess the effectiveness of the individual compounds. However, the isolation or the synthesis of individual compounds could be expensive and require testing for possible negative impacts on the environment or human health (Boxall et al., 2004; Gizaw et al., 2019).
Although the development of natural active ingredients requires substantial research and financial efforts, there is also a need for research on essential oil chemistry and biological activity to provide economic alternatives to synthetic insecticides (Alavez-Rosas et al., 2022). According to IVM theory, there is a need to optimize the use of available tools and resources for vector control, improving the efficacy, cost-effectiveness, ecological soundness, and sustainability of vector control interventions (Gizaw et al., 2019).
In summary, the present study found high adulticidal and larvicidal efficacy of a blend of essential oils in the fumigation assay and for contact toxicity, respectively. These effects were comparable to a synthetic insecticide used for house fly control. Additionally, at higher doses, the blend suppressed the pupation and adult emergence of the fly. The activity could be attributed to the major components present in the blend: the essential oils components, especially terpenoids, which have several biological effects and multiple modes of action and might be considered safe, available, and efficient alternatives to synthetic pesticides for the control of the housefly. These findings should, therefore, be subjected to field testing in the development of IVM as a tool for the control of this widely distributed vector.
Funding
The authors acknowledge to Grupo Herqueza for the financial support to project “Desarrollo de un atrayente para el monitoreo de la mosca domestica (Musca domestica L.)” Proyecto 25044.