Servicios Personalizados
Revista
Articulo
Indicadores
-
Citado por SciELO
-
Accesos
Links relacionados
-
Similares en SciELO
Compartir
Revista mexicana de fitopatología
versión On-line ISSN 2007-8080versión impresa ISSN 0185-3309
Rev. mex. fitopatol vol.35 no.2 Texcoco may. 2017
https://doi.org/10.18781/r.mex.fit.1611-6
Scientific articles
Antagonism of Saccharicola sp. against phytopathogens of the root of jalapeno pepper (Capsicum annuum)
1Instituto de Fitosanidad, Colegio de Postgraduados, km 36.5 Carretera México-Texcoco, Montecillo, Estado de México, C.P. 56230.
2Instituto de Edafología, Colegio de Postgraduados, km 36.5 Carretera México-Texcoco, Montecillo, Estado de México, C.P. 56230.
3Instituto de Fitosanidad, Colegio de Postgraduados, km 36.5 Carretera México-Texcoco, Montecillo, Estado de México, C.P. 56230.
The antagonist capacity of Saccharicola sp against Phytophthora capsici, Fusarium oxysporum and Rhizoctonia solani was evaluated in vitro and under greenhouse conditions. Through dual confrontation tests its impact was evaluated on the pathogen´s growth and the number of days when the antagonist invaded the pathogen mycelia, and also the viability of the invaded mycelia, and the production of pathogen propagules was determined. Saccharicola sp caused a reduction of 44.8 % and 45.1 % in P. capsici and F. oxysporum growth 3 days after confrontation (dac), and 36.2 % in R. solani at 2 dac. The microculture technique was used to determine its mycoparasitic potential. At 72 h, the antagonist winded around P. capsici mycelia. In R. solani starting at 24 h ac, different types of clefts were observed on its walls, at the points where it was in contact with the antagonist. Although Saccharicola sp in vitro conditions showed a negative effect against phythopathogens and there was evidence of mycoparasitism, under greenhouse conditions disease incidence and severity did not decrease nor promoted plant growth.
Key words: biological control; mycoparasitic; chili wilting; Phytophthora capsici; Fusarium oxysporum; Rhizoctonia solani
Se evaluó la capacidad antagónica de Saccharicola sp in vitro e invernadero contra Phytophthora capsici, Fusarium oxysporum y Rhizoctonia solani. Mediante confrontaciones duales se evaluó la reducción de crecimiento del fitopatógeno y se registró el número de días en que el antagonista invadió el micelio de los patógenos, se determinó la viabilidad del micelio invadido y la producción de propágulos del fitopatógeno. Saccharicola sp causó reducción de 44.8 % y 45.1 % en el crecimiento de P. capsici y F. oxysporum a los 3 días posteriores a la confrontación (dpc) y en R. solani 36.2 % a los 2 dpc. Para determinar su potencial micoparasítico se realizaron microcultivos. A las 72 h se observó el enrollamiento del antagonista alrededor de P. capsici. En R. solani desde las 24 h se observaron hundimientos en los sitios de contacto con el antagonista. A pesar que en condiciones in vitro Saccharicola sp mostró un efecto negativo contra los fitopatógenos y hubo evidencia de micoparasitismo, en invernadero no redujo la incidencia y severidad de la enfermedad, ni promovió el crecimiento de las plantas.
Palabras clave: control biológico; micoparásito; marchitez del chile; Phytophthora capsici; Fusarium oxysporum; Rhizoctonia solani
Chili pepper (Capsicum annuum) is one of the most important agricultural crops in Mexico (Castro-Rocha et al., 2012), as well as a basic component of the Mexican population diet (Rico-Guerrero et al., 2004). According to the Sistema de Información Agroalimentaria y Pesquera (SIAP), in 2014 the area grown to chili pepper was 148,968.51 ha with a national average yield of 19.05 ton/ha and estimated value of over 17 million pesos. However, from the area sown to chili pepper only 143,465.17 ha were harvested. One of the factors affecting chili pepper production is phytopathogenic damage to root caused by Rhizoctonia solani, Fusarium spp, Pythium spp and Phytophthora capsici. (Velásquez-Valle et al., 2001). The latter is considered a lethal pathogen able to infect both root and above-ground plant parts (Hwang and Kim, 1995; Erwin and Ribeiro, 1996; Ristaino and Johnston, 1999; Lamour et al., 2012), because it causes plants to wilt along with a fungal complex in which R. solani and Fusarium spp are repeatedly isolated (Ramos-Sandoval et al., 2010). Fungal control has been focused mainly on P. capsici using cultural practices, resistant varieties and chemicals, being chemicals the most commonly used. However, the high cost of production and problems caused by the frequent use of chemicals make it necessary to find alternatives for managing these pathogens.
An alternative for disease management is biological control through the introduction of antagonistic organisms, which combined with other methods, may significantly contribute to the effective management of root diseases (Ait-Lahsen et al., 2001). The Saccharicola sp fungus produces secondary metabolites known as “polyketides” (Rojas et al., 2011; Chapla et al., 2012) that act as antibiotics (erythromycin and tetracycline), anticancer (epothilones), immunosuppressants (rapamycin) and anticholesteremics (lovastatin) (Kwan and Schulz, 2011; Rojas et al., 2011).
Recently, Saccharicola sp was isolated from tule plants (Typha domingensis) (Silva, 2015), and knowing its potential as toxic metabolites producer, preliminary in vitro tests were performed to prove its antimicrobial properties. Chapla et al. (2012) previously reported the antifungal effect of Saccharicola sp against Fusarium spp, Cladosporium cladosporioides, C. sphaerospermum and Microbotryum violaceum. The Saccharicola genus is an endophyte that has been isolated from different Saccharum species (Leme et al., 2013) and aquatic plants such as Eichhornia azurea and E. crassipes (Almeida et al., 2015), among other species. Based on this information, it was considered relevant to investigate the potential of the Saccharicola sp fungus as antagonist of the root pathogens responsible for the greatest losses in chili pepper production. Thus, the objective of this study was to evaluate the antagonistic effect of Saccharicola sp against P. capsici, F. oxysporum and R. solani, under in vitro and greenhouse conditions.
Materials and methods
This study was conducted in laboratories and greenhouses of Colegio de Postgraduados en Ciencias Agrícolas, Campus Montecillo, located in the municipality of Texcoco, State of Mexico. The isolates used were Phytophthora capsici 6143, provided by Dr. Fernández-Pavía, from Universidad Michoacana de San Nicolás de Hidalgo; Saccharicola sp, Fusarium oxysporum and Rhizoctonia solani were provided by the laboratory 209 from Physiology Plant-Pathogen Interaction of Colegio de Postgraduados, campus Montecillo.
Antagonism tests in vitro
Dual confrontations were performed in order to evaluate the antagonistic effect of Saccharicola sp. A trial with seven treatments was established: 1) Antagonist-Phytophthora capsici (A-Pc), 2) Antagonist-Fusarium oxysporum (A-Fo), 3) Antagonist-Rhizoctonia solani (A-Rs), 4) Antagonist control (A), 5) Phytophthora capsici control (Pc), 6) Fusarium oxysporum control (Fo) and 7) Rhizoctonia solani control (Rs). Each treatment included 10 replications. In each Petri dish 90 mm in diameter filled with Potato-Sugar-Agar Infusion (IPAA), a cylinder 5 mm in diameter containing mycelium of the antagonist was placed 1 cm from the periphery and then kept in darkness at 27±1 °C. Four days later, a cylinder 5 mm in diameter filled with IPAA containing Pc, Fo or Rs mycelium was placed at the opposite end of the dish. A cylinder filled with Pc, Fo or Rs was placed at each end of the control dishes. The dishes were kept under the conditions previously mentioned.
Evaluated variables
Mycelial growth in the different treatments was measured (in cm) every 24 h and the number of days when hyphae of the confronted isolates came into contact was recorded for each treatment. When the mycelium of both the antagonist and the pathogen came into contact, the growth reduction percentage of the phytopathogen was calculated using the formula proposed by Sid-Ahmed et al. (1999). For this calculation we considered the growth average (in cm) of the pathogens in the control dishes, as well as the growth average from the dual confrontation.
The dishes were kept under observation during 30 days to record the number of days when the antagonist developed on the pathogens’ mycelium. The viability of the mycelium of the pathogens confronted with the antagonist was also evaluated when it developed on the pathogens colony. When the antagonist developed on P. capsici, F. oxysporum and R. solani colonies, five Petri dishes were taken and from each dish three cylinders containing medium and showing mycelial growth of the invaded phytopathogen, and sown in IPAA to determine whether Pc, Fo and Rs mycelium was viable. After sowing, observations were made every 24 h during 5 days to determine if the phytopathogen was developing.
To know the antagonist effect on phytopathogen propagule production (zoospores or spores) at 21 dpc for P. capsici, and at 30 dpc for F. oxysporum, the following procedure was used: for F. oxysporum, from five dishes containing A-Fo treatments and their corresponding control (Fo), three cylinders 5 mm in diameter filled with mycelium were taken from the side where the pathogen developed and placed in tubes (Sarstedt®) filled with 5 ml of sterile distilled water; then the cylinders were shaken, and, using a micropipette, 200 µl of suspension were taken to count micronidia using a hemacytometer (Marienfeld®).
For P. capsici, from each of the five Petri dishes, three cylinders 1 cm in diameter filled with mycelial growth were taken and placed in dishes 50 mm in diameter filled with sterile distilled water and incubated at 27±1 °C for 48 h under white light to induce sporangia formation. After this period, and to induce zoospore release, the dishes were kept at 4 °C for 20 min, and then at 27±1 °C for 1 h. Observations were made under a stereoscopic microscope to record the relative abundance of zoospores in relation to that of the control.
Mycelial interaction between Saccharicola sp-P. capsici and Saccharicola sp-R. solani
To know if the Saccharicola sp antagonist infects P. capsici and R. solani mycelia, microcultures were prepared following the technique described by Riddell (1950) with slight modifications. The experiment consisted of five treatments, and observations were made to four replications every 24 h during 15 days with a total of 60 Petri dishes for each treatment. The evaluated treatments were: 1) Antagonist-P. capsici (A-Pc); 2) Antagonist-R. solani (A-Rs); 3) antagonist control; 4) Pc control; and 5) Rs control.
Five mm cylinders containing the antagonist mycelium were sown in Petri dishes with IPAA at 1 cm from the periphery. A previously sterilized 18 x 18 mm cover slip was taken with a sterile tweezer and placed in the middle of each dish. After 72 and 96 h, a 5 mm cylinder containing Pc or Rs mycelium was placed at a distance of 1 cm. In all cases, the dishes were kept in darkness at 27±1 °C. The same procedure was used for the controls. Every 24 h during 15 days observations were made to cover slips in four replications of each treatment; when mycelium of both microorganisms was found in the middle of the cover slip, it was removed from the culture medium using a sterile razor. A drop of cotton blue was added to the cover slip and observed under a 100 X Olimpus® model CX31 compound microscope.
Scanning electron microscopy (MEB): The analyzed samples were taken from the interaction areas of confrontation between the antagonist and the pathogen (P. capsici) in IPAA medium. The samples were analyzed at the Red de Estudios Moleculares Avanzados, Instituto de Ecología A. C., Cluster BioMimic®, Xalapa, Veracruz, Mexico.
Antagonism tests in greenhouse: A trial was established from November 2015 to January 2016 using soil coming from the field and collected in a plot naturally infested with P. capsici. One portion of the soil was sterilized (SE) twice (2 h each) in an autoclave at 65 °C and 20 lb pressure, and then ventilated during 7 days. The other portion was not sterilized (SN). Eleven treatments were tested using a random blocks design with 10 pots (a jalapeño pepper plant of the “M” variety in each pot filled with 500 g of soil).
The treatments used were: 1) Control (SE-T), 2) SE-F. oxysporum (SE-Fo), 3) SE-P. capsici (SE-Pc), 4) SE-R. solani (SE-Rs), 5) SE-Antagonist (SE-A), 6) SE-Antagonist-F. oxysporum (SE-A-Fo), 7) SE-Antagonist-P. capsici (SE-A-Pc), 8) SE-Antagonist-R. solani (SE-A-Rs), 9) SE-Antagonist-F. oxysporum-P. capsici-R. solani (SE-A-Fo-Pc-Rs), 10) SN-Antagonist-F. oxysporum-P. capsici-R. solani (SN-A-Fo-Pc-Rs), and 11) SN-F. oxysporum-P. capsici-R. solani (SN-Fo-Pc-Rs). As reference of the pathogenicity of the P. capsici isolate used, chili plants of the highly susceptible variety “Yollow wonder” were also inoculated. The trial was repeated one more time.
Seed germination
Seeds of the jalapeño pepper M variety were disinfected with 2 % commercial sodium hypochlorite for 3 min and rinsed three times with sterile distilled water. Seeds from the treatments including the antagonist were introduced in a 1X108 colony-forming unit (ufc) of Saccharicola sp suspension; the other treatments were kept in sterile running water. The seeds were placed in sterile paper towels (sanitas) and left to germinate in darkness at 27±1 °C for 11 days.
Seedlings were transferred to polyestirene trays containing a previously sterilized mixture of Peat Mos and Agrolita (2:1). To the seedlings from the treatments including the antagonist a 1X108 ufc suspension (10 ml/seedling) was added immediately after being transferred to the trays. The trays were kept in a growth room with photoperiods of 14 h light and 10 h in darkness at 25±1 °C until seedlings developed the first pair of true leaves.
Preparing antagonist and pathogen inoculum, and inoculation.
Inoculum of the antagonist and of R. solani was prepared in 500 ml Erlenmeyer flasks containing potato-sugar infusion (IPA). The flasks were sterilized in autoclave at 120 °C for 15 min. Five cylinders 5 mm in diameter containing the antagonist or R. solani were aseptically transferred to the flasks and incubated for 10 days in a Lab-Line® shaking incubator at 110 revolutions per minute (rpm); mycelium was removed from the flasks and grinded in a blender.
From Petri dishes containing F. oxysporum or P. capsici mycelium, cylinders 5 mm in diameter were taken and sown in IPAA and in V8 juice agar (V8A), respectively; then, they were incubated in darkness at 27±1 °C. Seven days later, 10 ml of 0.9 % (Pisa®) sodium chloride isotonic solution were added to the Petri dishes containing P. capsici. Later, the solution was removed, and, using a dissection needle, the medium was divided in six fragments. Each fragment was transferred to a Petri dish containing 10 ml of sterile running water.
The dishes were kept under light for 48 h at 26 °C and 48 h in darkness at 28 h °C. After this period, they were placed at 4 °C for 20 min and then at 27±1 °C for 1 h to induce zoospore release. The number of antagonist and pathogen propagules was counted in a hemocytometer and adjusted to the inoculum density at 1X108 for the antagonist, and at 1X107 ufc for F. oxysporum and P. capsici; for R. solani, 1 g of previously grinded mycelium was added. The antagonist inoculation was made in seeds, when the seedlings were transferred to trays, plus two 10 ml applications three days later, one day before transferring the plants to pots, and immediately after transplanting them to pots. The patoghen inoculation was made three days after the antagonist was inoculated. All of them were applied at the base of the stem.
Evaluated variables
Records were taken of the incidence (%) of plants showing disease symptoms and severity (damage level), plant height, diameter of the base of the stem, as well as root and canopy dry weight. Observations were made every day to record the first two variables. P. capsici severity was evaluated using the scale proposed by Morán-Bañuelos et al. (2010); F. oxysporum, using the scale proposed by Marlatt et al. (1996), and R. solani severity, using the scale proposed by Navarrete-Maya et al. (2009). When the experiment ended (3 months after transplanting), the height of the plants and the diameter of the base of the stem were measured. Roots were cut at the base of the stem. Both canopy and roots were placed in Kraft paper and dried at 60° C on a stove (Felisa®) during 4 days. Five plants from each treatment were randomly taken to record their dry weight.
Statistical analysis: Data from the evaluated variables in all the trials were used to perform normal distribution (Shapiro-Wilk and Kolmogorov-Smirnov) and variance homogeneity (Bartlett) tests. For data obtained from the greenhouse trial we had to use a logarithmic transformation. Variance analysis (ANOVA) and means comparison (Tukey, P≤0.05) were performed using the SAS version 9.0 software. The analysis was performed with data from the two experiments.
Results
Antagonism tests in vitro: Under in vitro conditions, Saccharicola sp showed a significant reduction of 44.8 % and 45.1 % in P. capsici and F. oxysporum mycelial growth 3 days after confrontation (dpc); R. solani showed a reduction of 36.2 % at 2 dpc (Table 1). At 21 dpc, the antagonist grew on the P. capsici colony, and at 30 dpc, on the F. oxysporum colony. On R. solani, the growth of both reached the middle of the dish at 30 dpc and stayed there. Although the antagonist did not invade the pathogen, the absence of microsclerotia was evident on the R. solani colony on the side of the cylinder containing the pathogen, but they developed in both cylinders containing the control (Figure 1).
Table 1. Effect of Saccharicola sp (A) on P. capsici (Pc), F. oxysporum (Fo) and R. solani (Rs) mycelial growth, and on P. capsici sporangia and zoospore production.
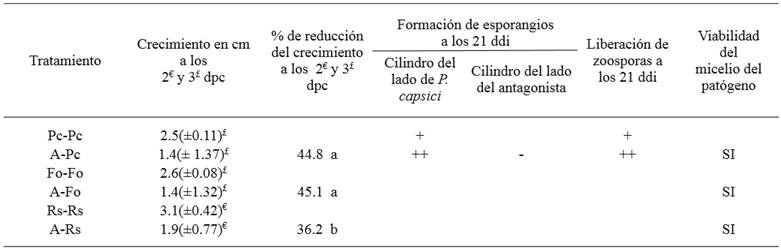
dpc= days after confrontation, ddi=days after the pathogen was invaded by the antagonist; Pc-Pc= P. capsici control; A-Pc= Saccharicola sp-P. capsici; Fo-Fo= F. oxysporum control; A-Fo= Saccharicola sp-F. oxysporum; Rs-Rs= R. solani control; A-Rs= Saccharicola sp-R. solani. (-), (+) and (++): denote absence and limited and regular presence of sporangia and zoospores, respectively. Values with the same letter are not significantly different according to Tukey’s test (P≤0.05).
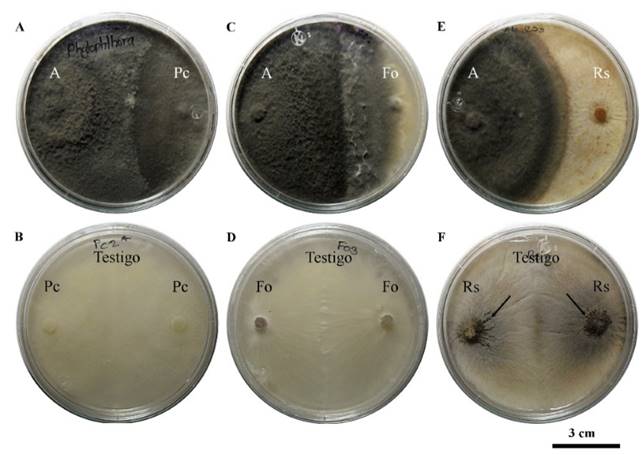
Figure 1. Saccharicola sp (A) antagonism against P. capsici (Pc), F. oxysporum (Fo) and R. solani (Rs) at 21 and 30 days after confrontation (dpc). A) A-Pc, B) Pc control at 21 dpc, C) A-Fo, D) Fo control, E) A-Rs and F) Rs control at 30 dpc showing microsclerotia development.
Saccharicola sp reduced the mycelial growth of F. oxysporum and P. capsici 72 h after confrontation (hpc) compared with their corresponding controls, which showed greater growth; growth reduction of R. solani was recorded starting at 24 hpc (Figure 2). Three days after sowing (dds), mycelial growth was observed in the cylinders containing mycelium, which were taken from the side of the pathogens invaded by the antagonist (Table 1) (Figure 3). In the cylinders containing the antagonist-F. oxysporum treatment, taken from the side of the pathogen, a lower number of micronidia was observed compared with those of the control (Table 2). In the antagonist-R. solani treatment microesclerotia were observed. We observed antagonist growth on P. capsici mycelium at 5 dds only in the cylinders taken from the antagonist-P. capsici treatment (Figure 3); in the cylinders from the same treatment placed in water, higher zoospore release was observed compared with that of the control cylinders (Table 2).
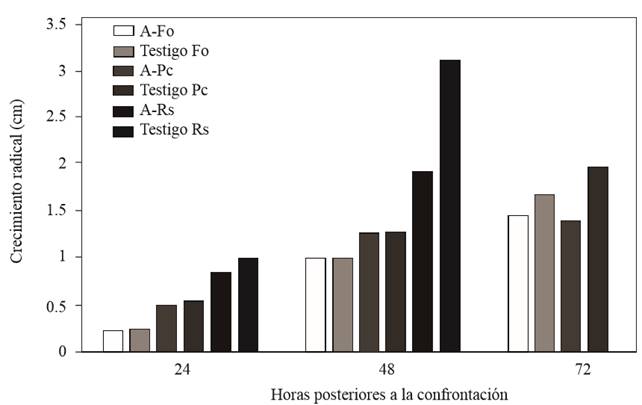
Figure 2. F. oxysporum (Fo), P. capsici (Pc) and R. solani (Rs) growth at a 24, 48 and 72 h after confrontation with Saccharicola sp.
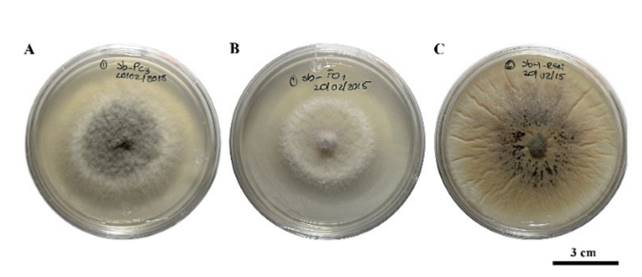
Figure 3. Viability of P. capsici (Pc), F. oxysporum (Fo) and R. solani (Rs) from cylinders containg mycelium taken from the side of the pathogen invaded by the antagonist. A) Pc and antagonist growth 5 days after cylinder sowing (dds), B) Fo growth without the antagonist, and C) Rs growth with the presence of microsclerotia and without the presence of the antagonist.
Table 2. Effect of Saccharicola sp (A) on P. capsici propagule production (sporangia and zoospore), F. oxysporum (microconidia) and R. solani (microsclerotia).

dpc= days after confrontation; Pc-Pc= P. capsici control; A-Pc= antagonist-P. capsici; Fo-Fo= F. oxysporum control; A-Fo= antagonist-F. oxysporum; Rs-Rs= R. solani control; A-Rs= antagonist-R. solani; relative abundance of propagules (-) none, (+) scarce and (++) regular.
Mycelial interaction between Saccharicola sp-P. capsici and Saccharicola sp-R. solani.
Under in vitro conditions, interaction between Saccharicola sp and P. capsici was observed 24 h after mycelia of both microorganisms came into contact, and the attachment phenomenon was observed (Figure 4). At 48 h, formation of Saccharicola sp pseudoparenchymal cells was observed on P. capsici hyphae, and at 72 h, growth around P. capsici hyphae (winding) (Figure 4). At 96 h, formation of P. capsici sporangia was observed, but in the controls sporangia appeared until day 5. At day 7, P. capsici hyphae showed vacuoles, cytoplasm granulation, deformation, and appeared to be empty (Figure 4).

Figure 4. Mycelial interaction between Saccharicola sp-P. capsici. A) Saccharicola sp winding around Pc hyphae 72 h after mycelia of both organisms came into contact (hpem), B) hyphae showing vacuoles (v) and cytoplasm granulation, C) deformed hyphae and seeming to be empty 7 days after mycelium of both microorganisms came into contact, D and E) P. capsici control at 72 h and 7 days after, respectively.
In R. solani, starting 24 h after mycelia of both microoganisms came into contact, sinking was observed in R. solani hyphae at the points of contact with Saccharicola sp (Figure 5); at those points we also observed the formation of small structures on Saccharicola sp. hyphae. At 96 h, at the points of contact with Saccharicola sp, R. solani hyphae showed deformation, and the mycelium, less intense coloration (Figure 5). At the points of interaction between the antagonist hyphae and the pathogens, we observed that the antagonist hyphae were smaller in diameter, which suggests that young mycelium is the one that comes into contact with the pathogen hyphae.
Scanning electron microscopy (MEB)
In the interaction Saccharicola sp-P. capsici, winding of the antagonist hyphae around P. capsici mycelium was observed (Figura 6), as well as formation of anchored structures on the same phytopathogen.
Saccharicola sp antagonist activity in the greenhouse
Under greenhouse conditions, P. capsici and F. oxysporum were pathogenic to chili pepper. The first caused 100 % disease incidence and 50 % mortality in infected plants. In F. oxysporum, 75 % incidence was recorded and 50 % dead plants; R. solani was not pathogenic to chili pepper. When the antagonist was inoculated along with the three pathogens, both in sterile soil and naturally infested soil, there was 100 % incidence in the chili pepper plants. The antagonist had no favorable effect on the diameter of the base of the stem, height or canopy and root dry weight. It was obvious that the application of Saccharicola sp did not protect the chili pepper plants from phythogens attack nor improved the plant development.
Discussion
Under in vitro conditions, Saccharicola sp caused a significant reduction of 44.8 % and 45.1 % in P. capsici and F. oxysporum mycelial growth 3 days after confrontation (dpc), but the reduction in R. solani was of 36.2 % at 2 dpc (Table 1). Chapla et al. (2012) also referred a similar effect for Saccharicola sp against Fusarium spp, Cladosporium cladosporioides, C. sphaerospermum and Microbotryum violaceum. The fact that Saccharicola sp mycelium come into contact with the pathogens mycelium at 3 and 2 dpc suggests that there is antagonist aggressiveness and pathogen susceptibility (Benhamou and Chet, 1993).
Regarding phytopathogens mycelial growth, Dennis and Webster (1971) mentioned that the production of antibiotics by biological control agents may inhibit the phytopathogen growth rate during an intense competition for nutrients. Thus, Saccharicola sp capacity to grow on P. capsici and F. oxysporum mycelium may result from the competition for nutrients in the culture medium and parasitosis that involves enzyme production (cellulases, xylanases, amylases and pectinases) (Paganini-Marques, 2013).
In the interaction R. solani- Sacharicola sp no inhibition or anchoring structures formation on the pathogen at 30 dpc was observed. However, R. solani did not develop microsclerotia (Figure 3). Dennis and Webster (1971) stated that antibiotics produced by some biological agents have inhibitory effects that may vary depending on the phytopathogen fungus.
Saccharicola sp has been found as endophyte in some plants. An endophyte isolate obtained from sugarcane produced secondary metabolites known as polyketides (Rojas et al., 2011); in another isolate obtained from Eugenia jambolana bioactive metabolites with antifungal activity were detected (Chapla et al., 2012; Honório, 2013; Borges, 2016). It is possible that the effect produced by Saccharicola sp in R. solani may have resulted from its capacity to produce such metabolites and that they have inhibitory action on microesclerotia formation. This effect is important to biological control, since many fungi form resistance propagules that allow them suvive under environmental harsh conditions even more than 20 years (Higuera-Moros et al., 2003).
In this study, the antagonist affected the capacity of the phythopathogen to produce fungus propagules: in P. capsici it was stimulated, but in F. oxysporum it was reduced. The greater number of sporangia and higher release of P. capsici zoospore when exposed to the Saccharicola sp antagonist (Table 2) is a phenomenom that has been reported by other researchers. For example, Sid-Ahmed et al. (1999) observed the production of P. capsici sporangia in the presence of Trichoderma harzianum. These authors stated that the stimulation of sporangia production was associated with the inhibition of P. capsici vegetative growth as a result of the antagonist action (Brasier, 1975; Sid-Ahmed et al, 1999) and the development of a defense mechanism by the pathogen (Dennis y webster, 1971; Elad et al., 1999).
The attachment observed in this study is an indicator of the chemical composition affinity of mycelia hyphae walls of both microorganisms and it is considered as the beginning of mycoparasitism (Pérez-Moreno, 1992). Saccharicola sp hiphae winding around P. capsici is interpreted as a parasitism expression (Benítez et al., 2004).
In this study we observed the antagonist hyphae to wind around P. capsici. Such effect was proven using scanning electron microscopy, which showed development of anchoring structures and vacuolization, granulation and deformation of P. capsici hyphae with appearance to be empty (Figure 4). This effect may have been caused by Saccharicola sp capacity to produce cellulases (Paganini-Marques, 2013). Those enzimes are involved in cellulose disintegration, which is the main component of cell walls (Köhl and Schösser, 1991; Castro-Rocha et al., 2012). The same effect was reported by Sid-Ahmed et al. (1999), who observed P. capsici vacuoles and hyphae disintegration in the presence of Trichoderma harzianum.
In R. solani, sinking in hyphae was observed at the points of contact with Saccharicola sp, where also formation of small papillated structures emerging from Saccharicola sp was detected. R. solani hyphae showed deformation and less intense coloration (Figure 5), possibly because of the effect of bioactive metabolites produced by Saccharicola sp (Chapla et al. 2012; Honório, 2013; Borges, 2016).
Although under in vitro conditions, the phytopathogens mycelial growth was reduced, and in the mycelial interaction tests we observed the beginning of Saccharicola sp parasitism on P. capsici, as well as mycoparasitism on R. solani, this action was not expressed in the greenhouse, possibly because of soil homeostasis that limits or prevents the establishment of any introduced species (Bautista-Calles et al., 2014). The antagonist applications could be more successful if they are frequently made during the crop cycle. For this reason, it is important to determine if Saccharicola sp weekly applications could help reduce phytopathogens incidence and severity in chilli pepper or other crops. It is also important to know which secondary metabolites have in vitro effect on P. capsici, F. oxysporum and R. solani mycelial reduction.
Conclusions
Under in vitro conditions Saccharicola sp significantly reduced Phytophthora capsici, Fusarium oxysporum and Rhizoctonia solani mycelial growth. However, under greenhouse conditions, Saccharicola sp did not protect the chilli pepper plants from phytopathogen attacks nor improved the plants development.
Acknowledgments
To Colegio de Postgraduados for funding this research, to Consejo Nacional de Ciencia y Tecnología (CONACYT) for the scholarship granted, and to M.Sc. Greta Hanako Rosas Saito from the Red de Estudios Moleculares Avanzados, Instituto de Ecología A. C., Cluster BioMimic® for samples analysis.
REFERENCES
Ait-Lahsen H, Soler AM, de la Cruz J, Monte E and Llobell A. 2001. An antifungal exo-a-1,3-glucanase (AGN13.1) from the biocontrol fungus Trichoderma harzianum. Applied and Environmental Microbiology 67:5833-5839. DOI: http://dx.doi.org/10.1128/aem.67.12.5833-5839.2001 [ Links ]
Almeida T.T., Orlandelli R.C., Azevedo J.L. y Pamphile J.A. 2015. Molecular characterization of the endophytic fungal community associated with Eichhornia azurea (Kunth) and Eichhornia crassipes (Mart.) (Pontederiaceae) native to the Upper Paraná River floodplain, Brazil. Genetics and Molecular Research 14:4920-4931. DOI: http//dx.doiorg/10.4238/2015.May.11.25 [ Links ]
Bautista-Calles J, Huerta-Lara M y García-Espinosa R. 2014. Antagonistas a Phytophthora capsici inoculados en sustratos de germinación de Capsicum annuum Leonian y Mucuna deeringiana Bort. Avances en investigación Agropecuaria 18:95-114. Disponible en línea: http://www.redalyc.org/articulo.oa?id=83731110007 [ Links ]
Benhamou N and Chet I. 1993. Hyphal interactions between Trichoderma harzianum and Rhizoctonia solani: ultrastructure and gold cytochemistry of the mycoparasitic process. Phytopathology 83:1062-1071. DOI: http://dx.doi.org/10.1094/phyto-83-1062 [ Links ]
Benítez T, Rincón AM, Limón MC and Condón A. 2004. Biocontrol mechanism of Trichoderma strains. International Microbiology 7:249-260. Disponible en linea: revistes.iec.cat/index.php/IM/article/viewFile/9480/9476 [ Links ]
Borges MS. 2016. Estudo da produção metabólica de Saccharicola sp., um fungo endofítico de Eugenia jambolana Lam. (Myrtaceae). Tesis de Maestría en Química. Universidade Estadual Paulista. Araraquara. 50-106 pp. Disponible en línea: http://hdl.handle.net/11449/136385 [ Links ]
Brasier CM. 1975. Stimulation of sex organ formation in Phytophthora by antagonistic species of Trichoderma. New Phytologist 74:183-194. DOI: http://dx.doi.org/10.1111/j.1469-8137.1975.tb02604.x [ Links ]
Castro-Rocha A, Fernández PS y Osuna AP. 2012. Mecanismos de defensa del chile en el patosistema Capsicum annuum-Phytophthora capsici. Revista Mexicana de Fitopatología 30:49-65. Disponible en línea: http://www.redalyc.org/pdf/612/61225129005.pdf [ Links ]
Chapla MV, Somensi A, Cavalheiro JA, Bolzani SV y Araujo AR. 2012. Substâncias acetilênicas produzidas por Saccharicola sp., um fungo endofítico isolado de Eugenia jambolana. 35a Reuniao Anual da Sociedade Brasileira de Química. Brasil.1p. Disponible en línea: http://sec.sbq.org.br/cdrom/35ra/resumos/T1941-1.pdf [ Links ]
Dennis C and Webster J. 1971. Antagonistic properties of species-groups of Trichoderma: I. Production of non-volatile antibiotics. Transactions of the British Mycological Society 57: 25-39. DOI: http://dx.doi.org/10.1016/s0007-1536(71)80077-3 [ Links ]
Elad Y, David DR, Levi T, Kapat A, Kirshner B, Gavrin E and Levin A. 1999. Trichoderma harzianum T39 mechanisms of biocontrol of foliar pathogens. 459-467 pp. In: Lyr H, Russell PE, Dehne HW and Sisler HD (eds.). Modern fungicides and antifungal compounds II. Intercept Publishers: Andover, England. 505 p. http://dx.doi.org/10.1016/S0031-9422(99)00417-3 [ Links ]
Erwin DC and Ribeiro OK. 1996. Phytophthora diseases worldwide. The American Phytopathological Society, St. Paul, Minnesota. 262-268 pp. DOI: http://dx.doi.org/10.1046/j.1365-3059.1998.0179a.x [ Links ]
Higuera-Moros A, Fontalvo J, Niño L, Sánchez J, Delgado J, Villalobos R y Montiel M. 2003. Crecimiento de Macrophomina phaseolina y Fusarium oxysporum en medios de cultivo de harina de semillas de frijol Vigna unguiculata (L.) Walp., frijol chino Vigna radiata L. y quinchoncho Cajanus cajan (L.) Millsp. Ciencia 11:14-21. Disponible en línea: http://www.produccioncientifica.luz.edu.ve/index.php/ciencia/article/view/9144/9133 [ Links ]
Honório AE. 2013. Prospecção química e biológica do fungo endofítico Saccharicola sp. isolado de Eugenia jambolana (Myrtaceae). Tesis de Maestría en Química. Universidade Estadual Paulista. Araraquara. 39-104 pp. Disponible en línea: http://repositorio.unesp.br/handle/11449/136385 [ Links ]
Hwang BK and Kim AH. 1995. Phytophthora blight of pepper and its control in Korea. Plant Disease 79:221-227. DOI: http://dx.doi.org/10.1094/pd-79-0221 [ Links ]
Köhl J and Schösser E. 1991. Antagonism against Rhizoctonia solani and cellulolytic activity of strains of Trichoderma. Developments in Agricultural and Managed Forest Ecology 23:160-164. DOI: http://dx.doi.org/10.1016/B978-0-444-88728-3.50029-9 [ Links ]
Kwan DH and Schulz F. 2011. The stereochemistry of complex polyketide biosynthesis by modular polyketide synthases. Molecules 16:6092-6115. DOI: http://dx.doi.org/10.3390/molecules16076092 [ Links ]
Lamour KH, Stam R, Jupe J and Huitema E. 2012. The oomycete broad-host-range pathogen Phytophthora capsici. Molecular Plant Pathology 13:329-337. DOI: http://dx.doi.org/10.1111/j.1364-3703.2011.00754.x [ Links ]
Leme, AC, Bevilaqua MRR, Rhoden SA, Mangolin CA, Machado MFPS and. Pamphile JA. 2013. Molecular characterization of endophytes isolated from Saccharum spp based on esterase and ribosomal DNA (ITS1-5.8S-ITS2) analyses. Genetics and molecular research12:4095-4105. DOI http://dx.doi.org/10.4238/2013 September 27.11 September 2013 [ Links ]
Marlatt ML, Correll JC and Kaufman P. 1996. Two genetically distinct populations of Fusarium oxysporum. f. sp. lvcopersici race 3 in the United States. Plant Disease 80:1336-1342. DOI: http://dx.doi.org/10.1094/pd-80-1336 [ Links ]
Morán-Bañuelos SH, Aguilar-Rincón V, Corona-Torres T y Zavaleta-Mejía E. 2010. Resistencia a Phytophthora capsici Leo. de chiles nativos del sur de Puebla, México. Revista Fitotécnia México 33:21-26. Disponible en línea: http://www.redalyc.org/articulo.oa?id=61014255004 [ Links ]
Navarrete-Maya R, Trejo-Albarrán E, Navarrete-Maya J, Prudencio-Sains JM y Acosta Gallegos JA. 2009. Reacción de genotipos de frijol a Fusarium spp. y Rhizoctonia solani bajo condiciones de campo e invernadero. Agricultura Técnica en México 35:455-466. Disponible en línea: http://www.scielo.org.mx/scielo.php?script=sci_arttext&pid=S0568-25172009000400011 [ Links ]
Paganini-Marques N. 2012. Prospecção de enzimas de degradação de material vegetal em fungos endofíticos. Tesis de Maestría en Biotecnología. Universidade Estadual Paulista. Araraquara. 31-86 pp. Disponible en línea: http://repositorio.unesp.br/bitstream/handle/11449/88017/000721712.pdf?sequence=1 [ Links ]
Pérez-Moreno J. 1992. Aspectos ecológicos; aislamiento de cepas, síntesis de micorriza y pruebas “in vitro” contra patógenos de diversos hongos ectomicorrizicos del parque nacional Zoquiapan, Edo. de México. Tesis de Maestría en Ciencias. Colegio de Postgraduados, Edo. de México. 87-90 pp. [ Links ]
Ramos-Sandoval RU, Gutiérrez-Soto JG, Rodríguez-Guerra R, Salcedo-Martínez SM, Hernández-Luna CE, Luna-Olvera HA, Jiménez-Bremont JF, Fraire-Velázquez S y Almeyda-León IH. 2010. Antagonismo de dos ascomicetos contra Phytophthora capsici Leonian, causante de la marchitez del chile (Capsicum annuum L.). Revista Mexicana de Fitopatología 28:75-86. Disponible en línea: http://www.redalyc.org/pdf/612/Resumenes/Resumen_61218468001_1.pdf [ Links ]
Rico-Guerrero L, Medina-Ramos S, Muñoz-Sánchez C, Guevara-Olvera L, Guevara-González R, Guerrero-Aguilar B, Torres-Pacheco I, Rodríguez-Guerra R y González-Chavira M. 2004. Detección de Phytophthora capsici Leonian en plantas de chile (Capsicum annuum L.) mediante PCR. Revista mexicana de Fitopatología 22:1-6. Disponible en línea: http://www.redalyc.org/pdf/612/61222101.pdf [ Links ]
Riddell RW. 1950. Permanent stained mycological preparations obtained by slide culture. Mycologia 42:265-270. DOI: http://dx.doi.org/10.2307/3755439 [ Links ]
Ristaino JB and Johnston SA. 1999. Ecologically based approaches to management of Phytophthora blight on bell pepper. Plant Disease 83:1080-1089. DOI: http://dx.doi.org/10.1094/PDIS.1999.83.12.1080 [ Links ]
Rojas JD, Durães SL, de Araujo WL, Garcez LMS, Ferreira da SL, Furlan RAR and Padilla G. 2011. The diversity of poliketide synthase genes from sugarcane-derived fungi. Microbial Ecology 63:565-57. DOI: http://dx.doi.org/10.1007/s00248-011-9938-0 [ Links ]
SAGARPA, Secretaria de Agricultura, Ganadería, Desarrollo Rural, Pesca y Alimentación. 2014. Servicio de información agroalimentaria y pesquera. Disponible en línea: http://www.gob.mx/siap/index (Consulta, febrero 2016). [ Links ]
Sid-Ahmed A, Pérez-Sánchez C, Egea C and Candela ME. 1999. Evaluation of Trichoderma harzianum for controlling root rot caused by Phytophthora capsici in pepper plants. Plant Pathology 48:58-65. DOI: http://dx.doi.org/10.1046/j.1365-3059.1999.00317.x [ Links ]
Silva VM. 2015. Saccharicola sp., agente de control biológico del tule (Typha domingensis Pers.). Tesis Maestría en Ciencias. Colegio de Postgraduados. Montecillo, Méx. 56 pp. Disponible en línea: colposdigital.colpos.mx:8080/jspui/handle/10521/48 [ Links ]
Velásquez-Valle R, Medina-Aguilar MM y Luna-Ruiz JJ. 2001. Sintomatología y géneros de patógenos asociados con las pudriciones de la raíz del chile (Capsicum annuum) en el Norte-Centro de México. Revista Mexicana de Fitopatología 19:175-181. Disponible en línea: http://www.redalyc.org/pdf/612/61219207 [ Links ]
Received: November 17, 2017; Accepted: April 03, 2017