Servicios Personalizados
Revista
Articulo
Indicadores
-
Citado por SciELO
-
Accesos
Links relacionados
-
Similares en SciELO
Compartir
Revista mexicana de fitopatología
versión On-line ISSN 2007-8080versión impresa ISSN 0185-3309
Rev. mex. fitopatol vol.39 no.3 Texcoco sep. 2021 Epub 13-Dic-2021
https://doi.org/10.18781/r.mex.fit.2105-3
Scientific articles
Biological control of Fusarium oxysporum causal agent of gladiolus corm rot by streptomycetes
1 Centro de investigación y asistencia en tecnología y diseño del estado de Jalisco, A.C. Subsede sureste. Tablaje catastral 31264 km 5.5. carretera Sierra Papacal-Chuburná Puerto. Parque Científico y Tecnológico de Yucatán. C.P. 97302. Mérida, Yucatán, México.
Fusarium oxysporum causes gladiolus corm rot and production damage could reach up to 100%. Fusarium isolates were selected from infected corms, one of them was morphologically and molecularly identified, and was selected from pathogenicity testing. One strain from 22 streptomycetes isolates that showed a 40% antagonist activity against Fusarium was selected. Bioactive extract (BE) was obtained by Solid Phase Fermentation and the minimum inhibitory concentration (MIC) and minimum lethal concentration (MLC) were determined by the microdilution method. A MIC of 0.19 mg mL-1 and an MLC of 0.38 mg mL-1 were obtained, which was confirmed with a conidia germination test at 8 h, which showed inhibition percentage of 17 and 98% for ¼ and ½ of the MIC. The effect of BE was evaluated at 1 and 2 MIC’s concentration against corm rot in infected gladiolus corm, obtaining a protective effect of gladiolus corms and maintaining their hardness after 15 days, in comparison with the fungicide Carbendazim. These results indicate Streptomyces sp., as a potential biological control agent against F. oxysporum.
Key words: Gladiolus grandiflorus; Actinobacterias; fungal activity; corms; protective effect
Fusarium oxysporum causa la pudrición del cormo en gladiolo provocando pérdidas de hasta el 100%. Se seleccionaron aislados de Fusarium a partir de cormos infectados, se identificó morfológica y molecularmente y se seleccionó un aislado a partir de prueba de patogenicidad. Se seleccionó entre 22 aislados de estreptomicetos una cepa que presentó una actividad antagonista del 40% contra Fusarium. Se obtuvo el Extracto Bioactivo (EB) mediante Fermentación en Fase Sólida y se determinó la concentración mínima inhibitoria (MIC) y concentración mínima letal (MLC) por el método de microdilución. Se obtuvo una MIC para el EB de 0.19 mg mL-1 y una MLC de 0.38 mg mL-1, que se confirmó con un ensayo de germinación de microconidios a 8 h, mostrando un porcentaje de inhibición del 17 y 98% para ¼ y ½ de la MIC. Se evaluó el efecto del EB a 1 y 2 MIC’s de concentración contra la pudrición en cormos de gladiolo infectados, obteniendo un efecto protector en los cormos al mantener su dureza después de 15 días, en comparación con el fungicida Carbendazim. Los resultados indican a Streptomyces sp., como un potencial agente de control biológico contra F. oxysporum.
Palabras clave: Gladiolus grandiflorus; Actinobacterias; actividad antifúngica; cormos; efecto protector
In Mexico, the gladiolus crop (Gladiolus grandiflorus) is considered the third most economically important ornamental crop, with a production value of over 1.1 billion pesos in 2020 (SIAP, 2020). However, several factors may reduce the volume and quality of the gladiolus production, including phytosanitary problems caused by fungi, bacteria and viruses (Elmer and Kamo, 2018).
Corm rot, induced by Fusarium oxysporum f sp. gladioli, is one of the most important disease in the gladiolus, since it causes the wilting and yellowing of leaves and a dry rot in the base of the corm that affects the quality of the floral rod (Khan et al., 2017). The appearance of the corm rot is a change in coloring which goes from light maroon to dark maroon to black and ends with the mummification of the corm. When the infection takes place in refrigerated corms, it causes a severe infection which may lead to the death of the plant and/or economic losses of 60 to 100% of the production (Khan et al., 2017; Elmer y Kamo, 2018).
Fusarium oxysporum is a cosmopolitan fungus that can survive for years in the soil without the presence of the host. On the other hand, the use of fungicides based on benzimidazoles and azoles is the commonly used control method. Considering that the persistence of the pathogen increases the use of agrochemicals for the phytosanitary control of the crops, another series of problems arises, such as the selection of strains of the pathogen that resist these products, the increase in production costs, and the risk of damages to the environment and human health, which are increasingly larger (Al-Hatmi et al., 2019; Marx-Stoelting et al., 2020).
Considering this, it is crucial to search for cheap and ecologically viable alternatives for the control of this pathogen (Alizadeh et al., 2020). One of the cheap and environmentally sustainable alternatives is the use of microorganisms to control diverse fungi and phytopathogenic bacteria (Goredema et al., 2020). In this regard, bacteria of the genus Streptomyces have been reported as antagonists to several pathogenic fungi. The antagonism of the streptomycetes is due to the production of secondary metabolites with fungicidal activity in combination with extracellular enzymes of the type of chitinases and pectinases (Pedroza et al., 2019; Danial et al., 2020; Tlemsani et al., 2020; Zhang et al., 2020). The strain CACIS-1.16CA (Streptomyces sp.) is a species that produces a mixture of yellow metabolites, which are soluble in water and have an inhibiting effect on the growth of phytopathogenic fungi in the genera Curvularia, Aspergillus, Helminthosporium, Fusarium, Alternaria, Phytophthora, Colletotrichum and Rhizoctonia (Evangelista-Martínez, 2014). In the biopesticide market there are two commercial products that are based on Streptomyces species, such as S. lydicus WYEC108 and S. griseoviridis K61, both of which control species of Fusarium spp. in different crops (Evangelista-Martinez et al., 2020; Shrestha et al., 2020). The aim of this investigation was to select antagonistic streptomycetes against F. oxysporum and to evaluate, in vitro and in vivo, the inhibiting activity of the bioactive extract of Streptomyces sp. isolations to control the rotting of corms in gladiolus.
Materials and methods
Isolation and characterization of the pathogenic fungus. From a plot of 60 gladiolus corms of the borrega blanca variety from the State of Mexico and with symptoms of corm rot (Figure 1), six corms were chosen, out of which three isolations of the fungus Fusarium sp. were isolated and purified (GL1, GL2 y GL3). Monosporic cultures were obtained, following the protocol reported by González-Pérez et al. (2009). They were then stored in Petri dishes with a potato, dextrose and agar (PDA) culture medium, and incubated in the dark at a temperature of 27 °C.
The morphological characterization of the fungi was carried out according to the characteristics described by Leslie et al. (2006). The identification at a genus level was carried out by comparing the morphological characteristics of the culture (shape and color of the culture, type of hyphae, presence of chlamidospores, macro and microconidia) and the shape, length and width of 50 macroconidia and 50 microconidia, chosen at random, were registered.
Molecular identification. Out of one of the three Fusarium spp. isolations, one monosporic culture of the isolation GL1 was chosen for its pathogenicity and growth rate in vitro. The molecular identification of the fungus was carried out with DNA extraction, following the extraction protocol with DNazol (Life Technologies), with some modifications. The amplification by PCR of the fragments of the gene that codifies for β-Tubulin was carried out with oligonucleotides T1 (5´-AACATGCGTGAGATTGTAAGT-3´) and T2 (5´-TAGTGACCCTTGGCCCAGTTG-3´) (O´Donell and Cigelnik, 1997), Histone 3 with oligonucleotides H3-1b (5´-CGCGGCGAGA CTGGATGTCCTT-3´), H3-1a (5´-ACTAAGCA GACCGCCCGCAGG-3´) (He et al., 2017), elongation factor 1α with oligonucleotides EF-1 (5′-ATGGGTAAGGA(A/G)GACAAGAC-3′), EF-2 (5′-GGA(G/A)GTACCAGT(G/C)ATCATG TT-3′) (Herkert et al., 2019), and finally, region ITS, using the universal oligonucleotides ITS4 (5′-TCCTCCGCTTATTGATATGC-3′) and ITS5 (5′-GGAAGTAAAAGTCGTAACAAGG-3′), following the protocol and amplification conditions reported earlier (Betancourt-Resendes et al., 2012; Uc-Varguez et al., 2018). The amplified fragments were purified and sent for sequencing to MACROGEN in Korea. The resulting sequence was edited using a sequence editing program (DNASTAR) and later compared with sequences reported in the NCBI data base, using the BLAST algorithm available on the Internet.

Figure 1 Corms from asymptomatic gladiolus of the variety borrega blanca (A). Corms with symptoms of rotting where F. oxysporum was isolated (B), which were reproduced with two cuts made with three punctures and the addition of 5 μL of a conidia solution at a concentration of 2.4 x106 conidia mL-1 of F. oxysporum GL1 (C). The perpendicular cut in the place of the wounds shows that 20 days after inoculation, rotting progresses inside the corm (D) and after three to four months, the corm displays a dry and dark rot (E).
Preparation of the inoculant. The conidial suspension used to obtain the MIC, MLC and for the corm infection experiments was prepared from a culture of the fungus grown for 11 days in Petri dishes with PDA medium. One Petri dish with the fungus was added 5 mL of sterile distilled water to remove the mass of conidia. The suspension was filtered through several layers of gauze and cotton to discard mycelial residues. The suspension was centrifuged at 6000 rpm/10 min at 25 °C. The conidial precipitate was washed twice with sterile distilled water, then resuspended and, using a Neubauer chamber, the concentration was adjusted to 1×105 conidia mL-1 or 2.4 × 106, according to the activity to be carried out in vitro or in vivo, respectively.
Pathogenicity test of the Fusarium sp. isolation. The pathogenicity of the fungus isolations was verified by inoculating 30 gladiolus corms. All corms were disinfected by submersion into a 0.3% NaOCl solution for 2 min, rinsed with sterile distilled water for 1 min, followed by submersion in 70% ethanol for 2 min and rinsed with sterile distilled water for 1 min. Finally, the excess moisture was eliminated using sterile paper towels (González-Pérez et al., 2009).
The disinfected corms were inoculated experimentally, with two cuts made in the upper part of the corm with three punctures (per cut) with a sterile toothpick. Later, in each cut, we placed 5 µL of a solution of 2.4x106 conidia mL-1 of F. oxysporum, obtained from a culture with 11 days of growth in a PDA medium. All corms, including the controls inoculated with sterile distilled water, were incubated in the dark in a bioclimatic chamber at 25 °C and 70% humidity. Symptoms were recorded in intervals of five days for 30 days. Finally, the corms were kept for four months under the same conditions and only the final symptom was recorded (González-Pérez et al., 2009).
Growth of Streptomyces spp. Cultures of 22 strains of Streptomyces were obtained from the Actinomycetes germplasm bank in the CIATEJ of the southeast, taken from soils from the states Aguascalientes, Campeche, Hidalgo and Yucatan. Additionally, in the trials, Streptomyces lydicus WYEC 108 was used as a control strain. All strains were grown in an International Streptomyces Project 2 (ISP 2) agar medium, at a temperature of 29 °C for 14 days. For the antagonism experiments, a general inoculant (GI) was prepared, consisting of spores from each strain at a concentration of 108 UFC mL-1 (Evangelista-Martínez, 2014).
Antagonistic evaluation of streptomycetes against F. oxysporum. The antagonistic activity of the 22 Streptomyces strains was carried out by dual confrontation tests in an ISP2 medium (Evangelista-Martínez et al., 2020). We inoculated 3 mL of the GI on the edge of the Petri dish with the ISP 2 medium, and in the middle of the dish, we placed an agar disk with active mycelia from a F. oxysporum culture with an 11-day growth in PDA. The cultures were kept at 29 °C for 11 days and the growth of the fungus was measured, in the direction of a streptomycete, using a digital caliper (Caldi-6MP Truper). The percentage of inhibition (PI) was calculated using the formula
, where “FR” represents the growth radius (mm) of the fungus in the control dish and “AR” is the radius of the fungal growth in the direction of the growth of Streptomyces (Dikhoba et al., 2019).
Table 1 Registered macroscopic and microscopic characteristics of the monosporic cul ture of F. oxysporum GL1 isolated from gladiolus corms with symptoms of rot.
Características morfológicas | zDescripción |
---|---|
Color de la colonia | Tonalidades naranjas y blancas, micelio aéreo |
Morfología del macroconidio | Fusiforme, ligeramente curvo |
Morfología de la célula apical | Cónica y curva |
Morfología de la célula de la base | Ovalada |
Largo de macroconidios (µm) | 52.9 |
Ancho de macroconidios (µm) | 7.4 |
Número de septos | 3 |
Morfología del microconidio | Ovalada |
Largo de microconidio (µm) | 20.4 |
Ancho de microconidios (µm) | 7.3 |
zThe measurements and records under the microscope were carried out by direct observation and measurements of de 50 macroconidia and 50 microconidia of the monosporic culture of the fungus at 40x.
Obtaining the Bioactive extract (BE) of Streptomyces sp. The bioactive extract of the two isolations with the greatest antagonistic activity (Streptomyces sp. GCAL-9 and CACIS 1.6 CA) was obtained by fermentation in a solid phase (FES), using polyurethane foam (Evangelista-Martínez et al., 2020). The support measured 12 x 10 x 2.5 cm, and 70 mL of ISP2 broth, inoculated with a suspension of spores from each one of the streptomycetes was added, adjusting the turbidity to a DO450 of approximately 1.0. The FES was carried out in sterile plastic containers for the in vitro cultivation of plants. The ISP2 broth was inoculated. The dishes were then closed and incubated in the dark at 29 °C for 21 days. The BE produced was separated from the cells by a series of filtrations, starting with Whatman No 3. Filter paper, followed by Whatman No. 1, 0.65 µm micropore filter, and ending in a 0.22 µm Micropore filter. The extract was stored at 4 °C until use (Evangelista-Martínez et al., 2020).
MIC and MLC with the method of microdilution. The minimum inhibiting concentration of the bioactive extract was carried out using 96 well plates in the potato dextrose broth medium (PDB) as per the Clinical and Laboratory Standards Institute (CLSI, 2017). The MIC tests were carried out in sterile microplates with 96 wells containing 100 µL of PDB broth. Later, the samples to be evaluated were added in each well of the first column, ending with a volume of 200 µL. The wells of the first column with the different samples were homogenized and 100 µL were taken from each well and placed in the wells of the second column, and so on, so the concentration in the first well is half of that of the concentration of the solution (two-fold dilutions). After finishing the dilutions, 100 µL of conidia were added, adjusted to 1 X 105 conidia mL-1; they were mixed and incubated at 29 °C. The inhibition of the fungal growth was determined after 24, 48 and 72 h by adding resazurin (Barua et al., 2017). The resazurin was used as a fungal growth indicator, and when the solution turned blue, it indicated growth inhibition, whereas when it was pink, it indicated growth or the absence of inhibition. The MIC was defined as the lowest concentration of the BE that inhibited the growth of F. oxysporum.
The value of the minimum lethal concentration (MLC) was determined when subcultivating directly from the plate used to calculate the MIC, 5 µL for the cell mass of the fungus of each well in the microplate with PDB broth. The cultures were kept at 29 °C for three days until mycelial growth was observed. The fungal growth after this period indicated fungistatic control, whereas the absence of growth indicated fungicidal activity of the BE. The MLC was defined as the lowest concentration in which there was no growth of the fungus. The BE of Streptomyces sp., GCAL-9 and CACIS 1.6 CA was analyzed in duplicate in two independent experiments. As the growth control, the product carbendazim (Syngenta) was used, prepared at a concentration of 72.8 mg mL-1.
Effect of the BE on the germination of microconidia. The inhibiting activity of the BE was evaluated with a test of the inhibition of the germination of the conidia. The test was carried out on a slide with a well with a final volume of 40 µL containing 20 µL of PDB broth mixed with 10 µL of the suspension of F. oxysporum conidia (105 conidia mL-1) plus 10 µL of the BE solution at different concentrations. The concentrations evaluated were ¼, ½, 1, 4, 6 and 8 MIC’s (0.0475, 0.095, 0.19, 0.38, 1.14, 1.52 mg mL-1). A slide was then placed and kept in a humid chamber at 29 °C in the dark. Sterile distilled water was used as a control. The effect on germination was determined every hour for 8 hours of growth, using an inverted microscope (Olympus), counting 100 conidia at random, separating the germinated conidia from the yet ungerminated ones (Evangelista-Martínez et al., 2020). The experiment was carried out in triplicate and the evaluation was based on the presence of the germination tube. A conidium was considered germinated if the germination tube reached a diameter twice that of the diameter of the conidium. Results were expressed as the percentage of germinated conidia (PG) in comparison with the control, based on the following formula (Zhang et al., 2020):
Table 2 Antagonistic activity of 22 streptomycetes on Fusarium oxysporum, deter mined with the percentage of inhibition of the fungal growth rate in the di rection of the actinomycete in dual antagonism trials.
Cepa | PI (%) | Cepa | PI (%) |
---|---|---|---|
Suelos del Parque Nacional El Chico, Hidalgo | |||
CACIA 1.3 HGO | 17.1 | CACIA 1.5 | 10.1 |
CACIA 1.33 HGO | 14.1 | CACIA 1.7 | 14.1 |
Suelos de la Reserva de la Biósfera Los Petenes, Campeche | |||
CACIS 1.16 CA | 43.4 | CACIS 2.30 CA | 19.1 |
CACIS 2.16 CA | 30.3 | CACIS 2.3 CA | 24.2 |
CACIS 2.17 CA | 32.3 | CACIS 2.5 CA | 19.1 |
CACIS 2.26 CA | 20.2 | ||
Suelos de campo de cultivo de chile serrano, Aguascalientes | |||
AGS 4 | 28.2 | AGS 13 | 32.3 |
AGS 6 | 24.2 | AGS 32 | 15.1 |
AGS 9 | 25.2 | AGS 44 | 26.2 |
AGS 10 | 35.10 | AGS 50 | 29.2 |
AGS12 | 23.2 | AGS 58 | 31.7 |
Suelos de Yucatán | |||
GCAL 9 | 40 | Streptomyces lydicus wyec 108z | 41.1 |
zCommercial strain used as a reference.
Fungicidal activity of the BE. The fungicidal potential of the BE on F. oxysporum was evaluated by carrying out spore germination tests at different concentrations and following up on different times of contact with the conidia. The experiments were carried out using the microdilution method described earlier. The concentrations of the BE were 1, 2 and 3 MIC’s. Carbendazim at 72.8 mg mL-1 was used as a positive control. After mixing the PDB broth and the BE, 100 µL of the conidia suspension were added and homogenized. Every hour for 4 h, 5 µL of the suspension were taken from each mixture and placed in Petri dishes with a PDA medium and incubated at 29 °C for three days. The optimal time of contact for the fungicidal effect was determined in the samples where no growth was observed.
Protective effect of the BE on gladiolus corms. The evaluation of the effect of the BE for the control of rot in gladiolus corms was carried out using 50 gladiolus coms of the Red Beauty variety for each treatment. The disinfection process of the corms and of the inoculation with the F. oxysporum conidia suspension was carried out as described earlier.
The treatments evaluated were a) corms inoculated with F. oxysporum and treated with water; b) corms inoculated with F. oxysporum and treated with Carbendazim (72.8 mg mL-1); c) corms inoculated with F. oxysporum and treated with BE MIC (0.19 mg mL-1) and d) corms inoculated with F. oxysporum and treated with BE and double the MIC (0.38 mg mL-1). Later, the corms in all treatments were stored in the dark in a bioclimatic chamber at 25 ºC and 70% humidity.
The symptoms were registered every 5 days from time zero until 20 days after inoculation, in five corms per treatment. The variable hardness or firmness of the corms was the parameter used to determine the protective effect of the BE on the inoculated corms, using a Shimadzu® EZ-SX digital texturometer with a needle, 5 mm in diameter, on a standard base table. The compression test was carried out at a speed of 3mm/s at a depth of 0.5mm. The firmness of the corms was registered in Newtons (Morales-Pérez et al., 2014).
Data analysis. The data registered for the PG were analyzed statistically using a multifactorial ANOVA and a test of separation of means using LSD. The data registered for corm firmness were analyzed statistically using a simple ANOVA and a Tukey test of separation of means, using the program Statgraphics centurion XVIII.
Results and discussion
Isolation and characterization of the pathogen. Three isolations (GL1, GL2 and GL3) with morphological characteristics of the genus were taken from gladiolus corms with symptoms of rotting related to the presence of Fusarium spp. From the three Fusarium isolations, monosporic strains were produced, characterized by the development of an aerial mycelium with whitish to orange colors, without curled hyphae. Fusiform and slightly curved macroconidia were also found, with a conical apical cell and an oval-shaped base cell, with one to three septa; the microconidia presented an oval morphology and without septa (Table 1). These characteristics coincide with those reported for F. oxysporum (González-Pérez et al., 2009; Hafizi et al., 2013; Leslie et al., 2006).
Molecular identification of the fungus isolated from corms with rot. The analysis of the sequence of the ITS fragments (584 pb) of the Fusarium oxysporum GL1 isolation showed, when comparing with sequences registered in the GenBank, a similarity of 96% with ITS sequences of the genus F. oxysporum. The same result was obtained when analyzing the sequences of the genes that codify β-Tubulin (549 pb, 95% similarity), histone 3 (458 pb, 94% similarity) and elongation factor of 1α (300 pb, 98% similarity). Considering the morphological characteristics and the close molecular similarity with species of the Fusarium genus, isolation GL1, isolated from corms with rot, belongs to F. oxysporum.
Pathogenicity test of the isolation of F. oxysporum. Isolation GL1 induced the appearance of symptoms, therefore molecular identification, as well as the in vitro and in vivo trials described were carried out using this strain. The inoculated corms presented an initial symptom after 15 days (Figure 1), which was characterized by a brown color inside the corm, as well as a loss of turgidity; superficially, the symptom was imperceptible (Figure 1C), but when the corm was cut perpendicularly, the color changed from the place of inoculation (Figure 1D). The corms dried three to four months after inoculation, becoming dark brown (Figure 1E). This corresponds with the symptoms described for the rot of gladiolus corms caused by Fusarium (González-Pérez et al., 2009; Michel-Aceves et al., 2014; Pedroza et al., 2019).
Antagonistic activity in vitro. The antagonistic activity of the 22 strains of streptomycetes against the isolation of F. oxysporum GL1 is shown in Table 2, where we can also see, for the streptomycetes in the soil of the states of Hidalgo and Aguascalientes, a PI lower than 20%, whereas for the streptomycetes from the soils of the Petenes, in two, the PI is slightly higher than 30%, and only for Streptomyces sp. CACIS-1.16CA is the PI higher than 40%. In the case of Streptomyces sp., GCAL-9, a PI of 40.4 was observed. Similar results were observed with control strain S. lydicus WYEC 108 (PI= 41.1%). In general, no differences were observed in the PI of S. lydicus and the streptomycetes CACIS-1.16CA and GCAL-9. Based on these results, these isolations were chosen to evaluate the corresponding BE. The PIs obtained with Streptomyces sp. CACIS-1.16 CA and Streptomyces sp. GCAL-9 are near to the results reported for other actinomycetes on F. oxysporum (PI de 58.1%), which confirms the capacity of some species of the genus Streptomyces to inhibit F. oxysporum (Goredema et al., 2020; Nguyen et al., 2020; Tlemsani et al., 2020). Earlier results with Streptomyces sp. CACIS 1.16CA showed antagonistic activity against other species of Fusarium, leading the fungal growth inhibition to fluctuate between 40 and 70% (Evangelista- Martinez, 2014).
Determination of the MIC and MLC of the BE against F. oxysporum . The bioactive extract (BE) of Streptomyces sp. GCAL-9, produced with a solid fermentation, as described in materials and methods, was used to obtain a minimum inhibiting concentration (MIC) and minimum lethal concentration (MLC) on F. oxysporum. The MIC obtained was 0.19 mg mL-1, while the MLC was 0.38 mg mL-1. The MIC obtained in the investigation is similar to that reported for other strains of Streptomyces, considered to have an outstanding microbial effect on different species of bacteria and fungi, including F. oxysporum (Hima-Bindu et al., 2017; Pérez-Rojas et al., 2015).
Effect of the BE on the germination of F. oxysporum conidia. The bioactive extract of the isolation CACIS 1.16 CA did not inhibit the germination of the Fusarium sp. GL1 conidia, suggesting that the antagonism it presented in the dual antagonism trials (Table 2) may be due to direct competition, and not to the production of extracellular compounds with antagonistic activity (Alizadeh et al., 2020). Considering this, subsequent trials were performed only with the BE of the strain of Streptomyces sp. GCAL-9.
The results of the evaluation of the effects of five concentrations of the BE of the Streptomyces sp. GCAL-9 strain on the germination of F. oxysporum conidia display significant differences (p≤0.05) in the percentage from applying ½ MIC, with a value of around 90% in comparison with the control. Higher BE concentrations completely inhibited germination (Figure 2A). At a morphological level, structural changes were found in the conidia with the BE at ½, 1 and 2 MIC´s in comparison with the conidia in the germination phase (Figure 2B). There is a clear disruption of the transition phase towards the formation of the germination tube, and as the concentration of the BE increases, the size of the conidia decreases, the elongated shape of the macroconidia changes to round and swollen, an excess of vacuoles forms in the cytoplasm, the septa are no longer visible, and an excess of vacuoles forms. These characteristics have been observed in other investigations (Cordova-Albores et al., 2016), particularly in the evaluation of the Jatropha curcas oil and other derived oils on the germination of F. oxysporum f. sp. gladioli conidia; the authors report some morphological effects on the conidia, such as irregular or swollen edges, few to no organelles and, in the germinated conidia, there was scarce growth of hyphae and the appearance of a high number of vacuoles in the cytoplasm.
Other authors reported that extracellular compounds from Streptomyces blastmyceticus affect the germination of Colletotrichum acutatum and F. oxysporum conidia, highlighting effects on the conidial membrane permeability, as well as changes in their morphology with a reduction in their size and the presence of a wrinkly surface in conidia and hyphae, in comparison with the smooth surface of not conidia not exposed to the compounds (Kim et al., 2019).
The optimum time for the fungicidal effect of the BE is determined by evaluating, for three days, the ability of germination of the conidia and the growth of the F. oxysporum mycelia after being exposed to different concentrations (1, 2 and 3 MIC´s) and times (0, 1, 2, 3 and 4 h) of exposure to the BE (Figure 3). The results show that the concentration of 1 MIC (0.19 mg mL-1), 2 MIC (0.38 mg mL-1) and 3 MIC (0.57 mg mL-1) inhibited the germination and growth of the mycelia of the fungus after on hour of being in contact with the BE of GCAL-9, and this effect was observed for the three days in which the subcultures were kept at 29 °C. Meanwhile, the Carbendazim in the evaluated doses (72.8 mg mL-1) helped reactivate the conidia and, therefore, the growth of the mycelia when transferred to the PDA medium (Figure 3). These results confirm the antimicrobial potential of the extracellular compounds released by the strain of Streptomyces sp. GCAL-9. On the other hand, they differ from the report by Alburqueque and Gusqui (2018), who mention that Carbendazim was 100% effective to inhibit the development of F. oxysporum 72 h after the treatment under in vitro conditions.
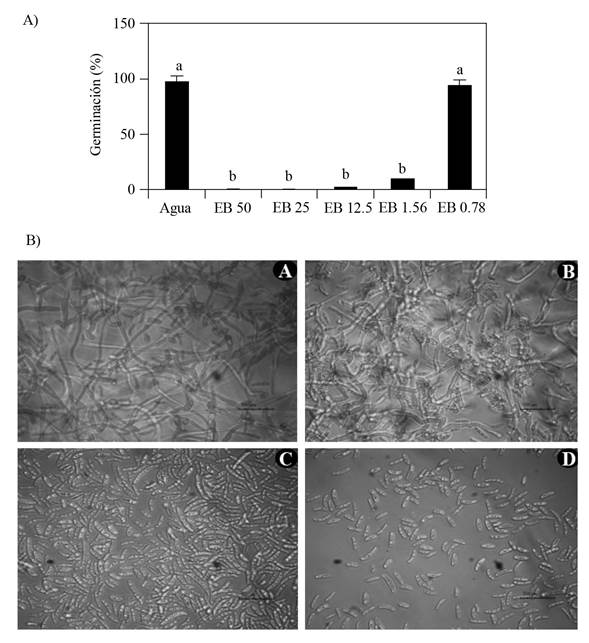
Figure 2 Effect of the bioactive extract (BE) on the germination of F. oxysporum conidia. 2A) Percentage of germination of conidia after 8 h of treatment with five concentrations of BE of Streptomyces sp., GCAL-9. Columns with the same letter are not statistically different, according to the LSD test (p≤0.05); 2B) Germination of the F. oxysporum conidia in water as a control A), was similar to the one that took place in 0.78% B) of the EB, while concentrations such as 25% C) and 12.5% D) inhibited the germination of the fungal conidia by 100%.
Control of rot in corms with the BE. The corms inoculated with F. oxysporum and protected with different concentrations of bioactive extracts did not show any differences in external symptoms in relation with the corms protected with Carbendazim the first 20 days after inoculation. On the other hand, the inoculated and unprotected corms displayed slight external symptoms, such as small dark brown spots in the inoculated areas, with creamy-white mycelia: These symptoms are similar to those reported for corm rot by Fusarium sp. (Pedroza et al., 2019; González-Pérez et al., 2009). Cuts of the inoculated rots displayed that the dark brown spot moved and expanded inside the corm (Figure 1). This suggests that there are differences between treatments after 15 days, but its estimation requires alternative methods to the symptom record used (González-Pérez et al., 2009).
Measurements of firmness taken with the Shimadzu digital texturometer showed that the corms inoculated with F. oxysporum and protected with the Streptomyces sp. G-CAL9 bioactive extract at 0.19 and 0.38 mg mL-1 were initially similar to the firmness of the control corms in the first 10 days after the treatment. However, the firmness of the corms treated with EB 15 (2MIC) and 20 days (1 MIC) after the treatment was greater in comparison with the controls treated with water and commercial fungicide (p≤0.05) (Figure 4), suggesting that the BE protected the corms from deterioration of the corms caused by the development of the pathogen inoculated (González-Pérez et al., 2009). The differences observed regarding the effectiveness in time of both concentrations of the BE may be due to factors such as differences in absorption, permanence and translocation of the BE, also influenced by differences in the surfaces of the corms used, as described by Mirabent (2012) as the main factors that exert an influence on the efficiency of the fungicides. Taking this into account, future evaluations will have to consider the use of coadjuvants that improve absorption, adherence, mobility, and therefore the efficiency of the BE.

Figure 3 Optimum time for the effect fungicidal of the BE of Streptomyces sp., GCAL-9. Mycelial growth in a Petri dish with a PDA medium after three days of subculture of the solution of F. oxysporum conidia from the microdilution on a plate with BE at 1, 2 and 3 MICs F and G), after 0 A), 1 B), 2 C), 3 D) and 4 E) hours in treatment with the BE.
On the other hand, the corms treated with the commercial Carbendazim-based fungicide displayed a similar firmness to that presented by the corms in the control treatment, suggesting that the commercial fungicide in the dose applied in the present study did not hinder the development of the fungus in the inoculated corms, since Carbendazim has an effect on the germination of the fungal conidia by inhibiting the assembly of the Beta-tubulin in mitosis and inhibiting the formation of the appressorium, the development of the mycelia of fungi (FRAC, 2019) as verified in the inhibition trials in microplates.
Conclusions
The study proved the antifungal potential of the bioactive extract obtained by fermentation in a solid phase of the strain of Streptomyces sp. GCAL-9, by inhibiting the total germination of conidia and the mycelial growth of F. oxysporum at a dose of 0.19 and 0.38 mg mL-1, and due to its protective effect, since it maintained the firmness of gladiolus corms inoculated with conidia of the fungus for 15 and 20 days, under controlled in vivo conditions.

Figure 4 Firmness of gladiolus corms experimentally inoculated with a solution of 5 μL of F. oxysporum conidia at 2 x 106 conidia mL-1 and protected with Streptomyces sp., GCAL-9 BE with one (0.19 mg mL-1) and two (0.38 mg mL-1) times the MIC concentration; registered 0, 5,10, 15 and 20 days after inoculation with a Shimadzu digital tex turometer. The test controls were corms inoculated with the fungus and protected with carbendazim at 72.8 mg mL-1 and water. Columns with the same letter are not significantly different, according to Tukey’s test (p≤0.05).
Acknowledgements
To the project “Desarrollo de un producto con metabolitos bioactivos producidos por bacterias Streptomyces para el control biológico de hongos patógenos que afectan frutos tropicales en la etapa de postcosecha” (Development of a product with bioactive metabolites produced by Streptomyces bacteria for the biological control of pathogenic fungi that affect tropical fruits in the postharvest stage). To the CONACYT FOINS-Problemas Nacionales NO. 2016-2900 fund for financing the article.
To the CONACYT through the scholarship for postgraduate studies granted to Tania Ameyally Rios Hernández for the study of her Master’s Degree in Floriculture Science in the CIATEJ of the Southeast.
REFERENCES
Alizadeh M, Vasebi Y and Safaie N. 2020. Microbial antagonists against plant pathogens in Iran: A review. Open Agriculture 5(1): 404-440. https://doi.org/10.1515/opag-2020-0031 [ Links ]
Al-Hatmi AMS, de Hoog GS and Meis JF. 2019. Multiresistant Fusarium pathogens on plants and humans: solutions in (from) the antifungal pipeline? Infection and drug resistance 12: 3727-3737. https://doi.org/10.2147/IDR.S180912 [ Links ]
Alburqueque D y Gusqui R. 2018. Eficacia de fungicidas químicos para el control in vitro de diferentes fitopatógenos en condiciones controladas. Arnaldoa 25(2): 489-498. https://doi.org/10.22497/arnaldoa.252.25209 [ Links ]
Barua P, You MP, Bayliss K, Lanoiselet V and Barbetti MJ. 2017. A rapid and miniaturized system using Alamar blue to assess fungal spore viability: implications for biosecurity. European journal of plant pathology 148:139-150. https://doi.org/10.1007/s10658-016-1077-5 [ Links ]
Betancourt-Resendes I, Velázquez-Monreal JJ, Montero-Castro JC, Fernández-Pavía SP, Lozoya-Saldaña H y Rodríguez-Alvarado G. 2012. Fusarium mexicanum Agente causal de la malformación del mango en Jalisco, México. Revista Mexicana de Fitopatología 30:115-127. http://www.redalyc.org/articulo.oa?id=61230188002 [ Links ]
Cordova-Albores LC, Zapotitla ES, Ríos MY, Barrera-Necha LL, Hernández-Lopez M y Bautista-Baños S. 2016. Microscopic study of the morphology and metabolic activity of Fusarium oxysporum f. sp. gladioli treated with Jatropha curcas oil and derivatives. Journal of Microscopy and ultraestructure 4: 28-35. https://doi.org/10.1016/j.jmau.2015.10.004 [ Links ]
CLSI. 2017. Performance standards for antimicrobial susceptibility testing. 27th ed. CLSI supplement M100. Clinical and laboratory standards institute; Wayne, Pennsylvania USA. 282p. http://file.qums.ac.ir/ repository/mmrc/clsi%202017.pdf [ Links ]
Danial AM, Medina A, Sulyok M and Magan N. 2020. Efficacy of metabolites of a Streptomyces strain (AS1) to control growth and mycotoxin production by Penicillium verrucosum, Fusarium verticillioides and Aspergillus fumigatus in culture. Mycotoxin Research 36: 225-234. https://doi.org/10.1007/s12550-020-00388-7 [ Links ]
Dikhoba P, Mongalo N, Elgorashi and Makhafola T. 2019. Antifungal and anti-mycotoxigenic activity of select South African medicinal plant species. Heliyon 5(10): https://doi.org/10.1016/j.heliyon.2019.e02668 [ Links ]
Elmer WH and Kamo KK. 2018. Diseases of Gladiolus. Pp. 1289-1311 In: McGovern R., Elmer W. (eds) Handbook of Florists´ Crops Diseases. Handbook of Plant Disease Management. Springer, Cham. https://doi.org/10.1007/978-3-319-39670-5-47 [ Links ]
Evangelista-Martínez Z. 2014. Isolation and characterization of soil Streptomyces species as potential biological control agents against fungal plant pathogens. World Journal of Microbiology and Biotechnology 30(5):1639-1647. https://doi.org/10.1007/s11274-013-1568-x [ Links ]
Evangelista-Martínez Z, Contreras-Leal EA, Corona-Pedraza LF and Gastelum-Martínez E. 2020. Biocontrol potential of Streptomyces sp. CACIS-1.5CA against phytopathogenic fungi causing postharvest fruit diseases. Egyptian Journal of Biological Pest Control 30: 117. https://doi.org/10.1186/s41938-020-00319-9 [ Links ]
FRAC. 2019. FRAC Clasificación de fungicidas y bactericidas según el modo de acción. Primera edición, España. https://www.syngenta.es/sites/g/files/zhg516/f/2019/04/clasificacion-fungicidas-bactericidas-segun-modo-accion.pdf [ Links ]
González-Pérez E, Yáñez-Morales MJ, Ortega-Escobar HM and Velázquez-Mendoza J. 2009. Comparative analysis among pathogenic fungal species that cause gladiolus (Gladiolus grandiflorus Hort.) corm rot in Mexico. Revista Mexicana de Fitopatología 27(1): 45-52. https://www.redalyc.org/articulo.oa?id=61211414006 [ Links ]
Goredema N, Ndowora T, Shoko T and Ngadze E. 2020. In vitro suppression of pathogenic fungi by Streptomyces spp. African crop science Journal 28(2): 141-149. https://doi.org/10.4314/acsj.v28i2.1 [ Links ]
Hafizi R, Salleh B and Lattifah Z. 2013. Morphological and molecular characterization of Fusarium solani and F. oxysporum associated with crown disease of oil palm. Brazilian Journal of Microbiology 44(3): 959-968. https://doi.org/10.1590/s1517-83822013000300047 [ Links ]
He M-H, Li D-L, Zhu W, Wu E-J, Yang L-N, Wang YP, Waheed A and Zhan J. 2017. Slow and temperature-mediated pathogen adaptation to a nonspecific fungicide in agricultural ecosystem. Wiley Evolutionary Aplications. https://doi.org/10.1111/eva.12526 [ Links ]
Herkert P, Al-Hatmi A, Salvador G, Muro M, Pinheiro R, Nucci M, Queiros-Telles F, Hoog G. and Meis J. 2019. Molecular characterization and antifungal susceptibility of clinical Fusarium oxysporum species from Brazil. Frontiers in Microbiology 10: 737. https://doi.org/10.3389/fmicb.2019.00737 [ Links ]
Hima-Bindu BSSN, Muvva V, Munaganti RK, Naragani K, Konda S and Dorigondla KR. 2017. Production of antimicrobial metabolites by Streptomyces lavendulocolor VHB-9 Isolated from Granite Mines. Brazilian Archives of Biology and Technology 60: https://doi.org/10.1590/1678-4324-2017160385 [ Links ]
Khan MR, Shahid S, Mohidin FA and Mustafa U. 2017. Interaction of Fusarium oxysporum and Meloidogyne incognita on gladiolos cultivars and its management through corm treatment with biopesticides and pesticides. Biological Control 115: 95-104. https://doi.org/10.1016/j.biocontrol.2017.09.010 [ Links ]
Leslie JF, Summerell BA and Bulloc S. 2006. The Fusarium laboratory manual. First edition. Ed. Blackwell Publishing Iowa, USA. 388p. https://doi.org/10.1002/9780470278376 [ Links ]
Marx-Stoelting P, Knebel C and Braeuning A. 2020. The connection of Azole fungicides with xeno-sensing nuclear receptors, drug metabolism and hepatotoxicity. Cells 9(5): 1192. https://doi.org/10.3390/cells9051192 [ Links ]
Michel-Aceves AC, Ariza-Flores R, Otero-Sánchez MO, Barrios-Ayala A y Quiroz-Millán AM. 2014. Efectividad in vitro e in situ de fungicidas químicos y biológicos en el control de Fusarium oxysporum f. sp. gladioli y Uromyces transversalis en gladiola. AP Agroproductividad 7: 3-11. https://core.ac.uk/download/pdf/249320317.pdf [ Links ]
Mirabent R. 2012. Adyuvantes para Fungicidas. Phytoma España: La Revista professional de Sanidad Vegetal 240: 64-65. https://www.phytoma.com/images /240empresas fungicidas _croda.pdf [ Links ]
Morales-Pérez AA, Franco-Mora O, Castañeda-Vildozola A and Morales-Rosales EJ. 2014. The anti-senescence effect of resveratrol reduces postharvest softening rate in cherimoya fruit. Scientia Agropecuaria 5: 35-44. http://dx.doi.org/10.17268/sci.agropecu.2014.01.04 [ Links ]
Nguyen PA, Strub C, Lagrée M, Bertrand-Michel J, Schorr-Galindo S and Fontana A. 2020. Study of in vitro interaction between Fusarium verticillioides and Streptomyces sp. using metabolomics. Folia Microbiológica 65: 303-314. https://doi.org/10.1007/s12223-019-00725-z [ Links ]
O´Donell K and Cigelnik E. 1997. Two divergent intragenomic rDNA ITS2 types whitin a monophyletic lineage of the fungus Fusarium are nonorthologous. Molecular phylogenetics and Evolution 7(1): 103-16. https://doi.org/10.1006/mpev.1996.0376 [ Links ]
Pedroza RR, Ribeiro WS, Silva SM, Finger FL, Zanuncio JC, Correa EB, Fugate KK, Bezerra da Costa F and Araujo RH. 2019. Healing of Gladiolus grandiflora corms under refrigeration and Fusarium oxysporum infection. Plant Signaling & Behavior 14(10). https://doi.org/10.1080/15592324.2019.1652520 [ Links ]
Pérez-Rojas F, León-Quispe J y Galindo-Cabello N. 2015. Actinomicetos aislados del compost y su actividad antagonista a fitopatógenos de la papa (Solanum tuberosum spp. andigena Hawkes). Revista Mexicana de Fitopatología 33(2): 116-139. http://www.redalyc.org/articulo.oa?id=61242145001 [ Links ]
SIAP (Servicio de Información agrícola y pesquera), 2020. Anuario estadístico de la producción agrícola 2020. https://www.gob.mx/siap. Julio 2021 [ Links ]
Tlemsani M, Fortas Z, Dib S and Bellahcen M. 2020. In vitro antagonism between actinomycete isolates and Fusarium oxysporum f. sp. ciceri: The causative agent of chickpea vascular wilt. South Asian Journal of Experimental Biology 10(4): 255-267. https://doi.org/10.38150/sajeb. [ Links ]
Uc-Varguez A, López-Puc G, Góngora-Canul C, Martinez-Sebastián M and Aguilera-Cauich EA. 2018. Spatio-temporal spread of foot rot (Lasiodiplodia theobromae) in Jatropha curcas L. plantations in Yucatán, México. European Journal plant pathology 150: 991-1000. https:// doi.org/10.1007/s10658-017-1338-y [ Links ]
Kim YJ, Kim JH and Rho JY. 2019. Antifungal activities of Streptomyces blastmyceticus strain 12-6 against plant pathogenic fungi. Mycobiology 47(3): 329-334. https://doi.org/ 10.1080/12298093.2019.1635425 [ Links ]
Shrestha U, Dee ME, Piya S, Ownley BH, and Butler DM. 2020. Soil inoculation with Trichoderma asperellum, T. harzianum or Streptomyces griseoviridis prior to anaerobic soil disinfestation (ASD) does not increase ASD efficacy against Sclerotium rolfsii germination. Applied soil ecology 147: 103383. https://doi.org/10.1016/j.apsoil.2019.10338 [ Links ]
Zhang X, Wang H, Zhu W, Li W and Wang F. 2020. Transcriptome analysis reveals the effects of chinese chive (Allium tuberosum R.) extract on Fusarium oxysporum f. sp. radicis‐lycopersici spore germination. Current Microbiology 77: 855-864. https://doi.org/ 10.1007/s00284-020-01875-x [ Links ]
Received: May 31, 2021; Accepted: August 03, 2021