Introduction
Mangrove ecosystems are defined by their hydrology and type of vegetation, which is adapted to varying inundation, salinity, and reduced soil conditions (Kuenzer et al. 2011). The different types of mangroves provide refuge, nursery, and foraging areas for fishes (Vaslet et al. 2015), and play a role in nutrient input and removal processes (Moroyoqui-Rojo et al. 2015).
Resources, regulators, and hydroperiod are environmental factors that determine the structure and function of mangroves (Twilley and Rivera-Monroy 2005). Resources (nutrients) are associated with water sources and microbial activities (Cardona-Olarte et al. 2006, Whigham et al. 2009, Reef et al. 2010). Regulators (stress factors), such as salinity, sulfides, and temperature, have an affect on the ecophysiology of mangrove plants. Salinity is one of the main factors regulating recruitment (successful incorporation into a population of new individuals) (McKee 1995, Ricklefs and Miller 2000) and mangrove development (Feller et al. 2010). Hydroperiod is defined by inundation frequency (number of floods in a given period of time), flooding duration (how long flooding persists), and water level, and is considered a key factor for mangrove growth (Krauss et al. 2008, Bashan et al. 2013).
Topography and hydroperiod maintain a direct relationship with soil properties and mangrove vegetation (Komiyama et al. 1996, Flores-Verdugo et al. 2006, Dahdouh-Guebas et al. 2007). Topography is therefore another important factor that defines the hydroperiod and allows the establishment of different mangrove species (Flores-Verdugo et al. 2007). The subtropical mangrove species from the American continent differ in their tolerance to flooding. Differences in growth and survival are attributed to diverse physiological factors since each mangrove species responds to local environments and ecological controls (Monroy-Torres et al. 2014).
Regeneration occurs via biological processes and under favorable environmental conditions. A disturbance can limit mangrove growth and development. Hence, the regeneration of mangroves is directly influenced by the reigning environmental conditions that will affect the stages of plant development (Sousa et al. 2003). Changes in hydroperiod can thus affect growth and survival during the seedling and juvenile stages (McKee 1995, Kitaya et al. 2002, He et al. 2007). In a perturbed habitat, seedling recruitment occurs when the values of the regulators are close to those of a natural ecosystem (Bosire et al. 2008). Consequently, this indicator can help us understand the response of a mangrove forest to a natural disturbance and can be used as a measure of ecological restoration.
Natural and human-caused disturbances have different effects (positive, negative, or neutral) on mangroves (Flores-Verdugo et al. 2006). The time a mangrove ecosystem takes to recover will depend on its resilience or its historical development trajectory (SER 2004). When it can no longer recover independently, some active intervention is necessary. Restoration activities should be designed taking into consideration information of the pre-existing structure, composition, and function of the ecosystem or of a reference ecosystem (SER 2004). Mangrove restoration activities include hydrologic rehabilitation, reforestation, or a combination of both (SER 2004). In any case, each method requires basic knowledge of the regional hydroperiod, which in turn requires knowledge of the tidal regime and microtopography (Flores-Verdugo et al. 2007). Restoration success will depend on the measures implemented, but environmental monitoring is necessary to determine the recovery trajectory (Zaldívar-Jiménez et al. 2010).
In the Yucatán Peninsula (Mexico), anthropogenic activities have reduced the ability of mangroves to respond to disturbances such as storms and hurricanes (Zaldívar-Jiménez et al. 2010, Adame et al. 2013). The hydrologic system has been modified by roads that disrupt water flow, by changes to barrier islands, and by the dumping of dredged sediments (Teutli-Hernández 2008, García de Fuentes et al. 2011). In the state of Yucatán, several restoration projects have been carried out along the coast but have not been as successful as expected because the hydrologic conditions and sedimentary characteristics necessary for mangrove regeneration were not taken into account (Zaldívar-Jiménez et al. 2010). To implement mangrove restoration strategies based on hydrologic rehabilitation and monitor the natural regeneration, a restoration program was developed that focused on the desilting of tidal channels to rehabilitate the hydroperiod and on modifying the topography to improve natural recruitment and development of the black mangrove (Avicennia germinans) (Zaldívar- Jiménez et al. 2010) . The purpose of the present study was to assess the natural regeneration of A. germinans by monitoring its growth and development in relation to hydroperiod, salinity, topography, and soil properties after the restoration actions. Our hypothesis is that the natural regeneration of a mangrove species differs depending on environmental gradients.
Materials and methods
Study area
The study area is located within the Chelem Lagoon system, in the northern part of the Yucatán Peninsula (Fig. 1). The soils are of karstic origin and the area is characterized by high evaporation and scant rainfall (mean annual rainfall of 587 mm). Mean annual temperature is 25.8 ºC; during the coldest season the temperature is 22.5 ºC. The climate is mainly hot and dry, but hot and humid in summer (Orellana and Espadas 2003).

Figure 1. Map of the study area showing the location of the 6 sampling sites: 3 restored mangrove sites (sites 1-3), 1 natural mangrove site (reference, site 4), and 2 sites with higher and lower elevation relative to the other sites.
The mangrove habitat in the study area has been affected by anthropogenic activities. Dredged sediments from the port of Yucalpetén were dumped in the area and modified the topography, hydroperiod, and consequently the physical and chemical properties of the soil, affecting the structure of the mangrove forest community. Prior to the ecological restoration, bulk density was 0.81 ± 0.19 g cm-3 and litterfall was 2.94 ± 0.25 g m-2 d-1 (Adame et al. 2013).
Mangrove degradation was primarily caused by the silting up of natural channels because of the disposal of degraded material in surrounding areas. Hydrologic rehabilitation thus became the main restoration action. Rehabilitation activities were carried out between 2009 and 2010, and consisted in manually desilting tidal channels and modifying the topography. With the help of the Chelem community, 2,000 m of tidal channels were desilted to restore the hydrology in the degraded area. As a result of this effort, A. germinans propagules and recruitment were subsequently observed in the restoration area.
Sampling sites
To determine the characteristics of the soil, hydrology, and natural regeneration of A. germinans, permanent sampling units were established at 6 sites (Fig. 1). Sites 1, 2, and 3, located within the area selected for restoration activities, had dead trees and were void of vegetation at the beginning of the study. At site 4, the adjacent reference site, the man-grove forest was in a natural state. Two other reference sites were established, one at a higher elevation (+0.24 meters above sea level [masl]) and the other at a lower elevation (- 0.04 masl) relative to the other sites. The latter was permanently flooded and seedlings were not observed at either of these 2 sites.
Soil characteristics
The characteristics of the water and soil were monitored from 2008 to 2012. For the analysis, data were divided into those obtained before (2008 and 2009) and after (2010 to 2012) implementing the restoration activities. Soil was characterized at each site based on bulk density, organic matter content, and microtopography (Chen and Twilley 1999). Bulk density and organic matter were analyzed in 2008 (before) and 2013 (after). The microtopography was classified in 2013 using a STONEX STS2-R Total Station; elevation was established relative to mean sea level using datum WGS84.
Hydrologic characteristics
Porewater salinity and hydroperiod were measured at each site. Soil salinity was measured in situ every 2 months with a YSI model 30 multiparameter probe. Hydroperiod was determined with a pressure sensor (HOBO, Onset Computer Corporation, USA) installed in the study area; the collected data were converted into water levels. Measurements were carried out continuously over one year and used to determine inundation frequency, water level, and flooding duration.
Natural regeneration of Avicennia germinans
To assess the natural regeneration of A. germinans, permanent sampling units of 314.16 m2 were established. All individuals within each unit were labelled with a plaque for subsequent monitoring. Plant species, identification number, and height were recorded. Height (cm) was measured with a graduated ruler from the soil to the highest apex of the plant. Measurements were carried out every 6 months for plant density, relative growth rate in height (RGRH), and survival. Density was calculated as the number of individuals per unit (i.e., per 0.01 ha). RGRH (mm d-1) was measured relative to time one (Rodríguez-Ramírez et al. 2004).
Statistical analysis
Two-way ANOVA was used to determine the differences among sites and restoration period (before or after) with respect to water and soil variables. A redundancy analysis (RDA) was carried out to determine the association between the response (natural regeneration) variables and the soil and hydrologic variables. RDA explains the response variables as a function of the explanatory variables. It can also be seen as an extension of the principal components analysis. What distinguishes RDA is that it produces a predictive model based on response variables with a linear combination of the predictor variables. The total proportion of variance can be explained by the linear combination of the predictor variables (Tabachnick and Fidell 1996, Legendre and Legendre 1998). Analyses were done using the R (v2.11.1) command console and Tinn-R editor (R Core Team 2012).
Results
Soil characteristics
Changes in bulk density (P < 0.01) were observed at sites 1, 2, and 3 (restoration area) during the study period, decreasing from 1.33 ± 0.23 g cm-3 (before implementing restoration actions) to 0.60 ± 0.01 g cm-3 (after). It also decreased at site 4 (reference), from 0.95 ± 0.18 to 0.55 ± 0.08 g cm-3 (Table 1). Changes in bulk density were not recorded at the higher and lower elevation sites. Organic matter content differed among sites (P < 0.0001), the lower and higher elevation sites having the highest and lowest values (23 ± 0.40% and 14 ± 0.39%, respectively) (Table 1).
Table 1 Comparison of the soil variables before and after implementing restoration actions (mean ± SE).
Site | |||||||
Restoration variable | n | 1 | 2 | 3 | 4 | Lower elevation | Higher elevation |
Before | |||||||
Salinity | 32 | 78.40 ± 3.60 | 79.50 ± 3.80 | 70.00 ± 2.40 | 71.60 ± 1.80 | 67.40 ± 4.00 | 85.80 ± 4.30 |
Bulk density (g cm-3) | 8 | 1.15 ± 0.60 | 1.05 ± 0.53 | 1.80 ± 0.71 | 0.95 ± 0.18 | 0.47 ± 0.02 | 1.89 ± 0.05 |
Organic matter (%) | 8 | 16.90 ± 0.93 | 19.10 ± 1.29 | 16.90 ± 1.37 | 17.40 ± 0.25 | 22.50 ± 0.53 | 13.50 ± 0.55 |
After | |||||||
Salinity | 40 | 71.70 ± 3.50 | 72.70 ± 3.30 | 66.50 ± 1.70 | 64.50 ± 1.80 | 63.40 ± 2.30 | 75.90 ± 2.60 |
Bulk density (g cm-3) | 12 | 0.63 ± 0.09 | 0.59 ± 0.05 | 0.60 ± 0.14 | 0.55 ± 0.09 | 0.40 ± 0.01 | 1.81 ± 0.03 |
Organic matter (%) | 12 | 17.30 ± 1.67 | 19.30 ± 0.73 | 20.00 ± 2.00 | 17.80 ± 1.18 | 23.50 ± 0.57 | 14.50 ± 0.50 |
Topographic elevation at site 4 was +0.14 ± 0.02 masl, and at the higher and lower elevation sites it was +0.24 ± 0.03 and -0.04 ± 0.01 masl, respectively. The natural regeneration of A. germinans occurred between 0.087 and 0.14 m above mean sea level (Table 2) . Seedling establishment was not observed at the higher and lower elevation sites.
Table 2 Variation of hydroperiod and porewater salinity at several mangrove sites (mean ± SE). Values for topographic elevation are meters above sea level (masl).
Site | Topographic elevation (masl) | Water level (m) | Flooding duration (h mo-1) | Frequency of inundation (no. tides mo-1) | Porewater salinity |
1 Site 1 | +0.12 ± 0.01 | 0.13 ± 0.09 | 481 ± 4.69 | 8 ± 0.64 | 75.16 ± 2.78 |
1 Site 2 | +0.09 ± 0.02 | 0.14 ± 0.09 | 524 ± 4.44 | 7 ± 0.70 | 76.79 ± 3.16 |
1 Site 3 | +0.08 ± 0.01 | 0.15 ± 0.09 | 529 ± 4.40 | 7 ± 0.70 | 69.01 ± 2.21 |
1 Site 4 | +0.14 ± 0.02 | 0.11 ± 0.08 | 439 ± 4.75 | 8 ± 0.64 | 69.23 ± 1.86 |
1 Lower elevation | -0.04 ± 0.01 | 0.28 ± 0.10 | 680 ± 2.74 | 4 ± 0.58 | 64.83 ± 3.46 |
1 Higher elevation | +0.24 ± 0.03 | 0.08 ± 0.08 | 282 ± 4.33 | 8 ± 0.64 | 80.88 ± 3.57 |
2 Florida, USA | +0.46 | 0.18 | 424 | No data | 70 |
3 Punta Guatales, Honduras | No data | 0.16 | 350 | 8 | 84.2 |
4 Sinaloa, Mexico | 0.55 | 0.64 | No data | No data | 65 |
Reference: 1 this study; 2Lewis (2005), Chen and Twilley (1998); 3Castañeda-Moya et al. (2006); 4Flores-Verdugo et al. (2007).
Hydrologic characteristics
Differences in salinity were observed between sites (P < 0.0001) and between restoration time (P < 0.003). After the restoration actions, the lowest salinity value (64.50 ± 1.80) was recorded at site 4 (reference) and the highest (75.90 ± 2.61) at the higher elevation site (Table 1).
Differences in water level and flooding duration were observed between sites (P < 0.05) and between restoration time (P < 0.05) (Table 2). Four floods per months were recorded at the lower elevation site, whereas 7 to 8 floods per months were recorded at the other sites. Water level was highest (0.28 m) at the lower elevation site and lowest (0.08 m) at the higher elevation site. Mean water level was 0.14 m at sites 1-3 and 0.11 m at site 4 (reference) . Mean flooding duration decreased as elevation increased. It was highest at the lower elevation site (680 h mo-1), followed by sites 1-3 (529 h mo-1), site 4 (439 h mo-1), and the higher elevation site (282 h mo-1).
Natural regeneration of Avicennia germinans
The density of A. germinans plants showed differences between sites (P < 0.05). Density was highest at site 4 (reference), reaching 307 individuals per 0.01 ha, but decreased to 88 individuals at the end of the monitoring period. It was lowest at site 2, though it increased from 6.78 to 12.42 individuals per 0.01 ha during the monitoring period. Plant density at the restoration sites (1, 2, and 3) was lower than at the reference site (4); however, the mean value obtained for site 3 (50.95 individuals per 0.01 ha) was the closest to that obtained for site 4 (Fig. 2a).

Figure 2. Variables used to assess the natural regeneration of Avicennia germinans: (a) density, (b) survival, (c) height, and (d) relative growth rate (mean ± SE). Plant density and height are shown for the years 2008-2013. Survival and growth rate are shown for the following time intervals: 0.7, 1.8, 2.4, and 3.8 years.
There were no differences in the survival of A. germinans plants between sites. Survival at the restoration sites tended to increase over time, especially at site 1, where it increased from 42.85% (0.7 years) to 99.28% (3.8 years) . Survival values remained constant at sites 1-3 over the 3.8 years, indicating the success of the restoration actions. Survival at the reference site (4) decreased from 100% to 29.79% (Fig. 2b).
Differences in plant height (P < 0.05) were observed between sites. At the end of the study, the highest mean value (99. 84 cm) was recorded at site 4 (reference) and the lowest (55 cm) at site 1. Within the restoration area, site 3 had the highest mean value (66 cm) (Fig. 2c).
There were no differences in RGRH between sites. During the first period (0.7 years), RGRH increased at all sites but was greater (0.067 mm d-1) at site 4 (reference). After 1.8 years, RGRH decreased at sites 2, 3, and 4. The largest increase in RGRH occurred after 2.4 years, the highest value (0.159 mm d-1) corresponding to site 4 and the lowest (0.11 mm d-1) to site 1. After 3.8 years, a decrease was observed at all sites, the values ranging from 0.078 to 0.087 mm d-1 (Fig. 2d).
Environmental variables influencing regeneration
We determined the relationship between the soil and hydrologic characteristics and the natural regeneration of A. germinans. Components RDA1 and RDA2 explained 91.2% of the total variance through new variables created from the original ones. RDA1 explained 64% of the variance, the most relevant variables being microtopography and hydroperiod (inundation frequency, water level, and flooding duration). RDA2 explained 27% of the variance, the most relevant variables being organic matter and porewater salinity (Table 3). Sites 1 and 4 correlated with the frequency of inundation and microtopography, explaining the response variables relative to plant density, height, and RGRH. Site 2 correlated with organic matter and porewater salinity. Site 3 correlated with water level and flooding duration. In this study, we determined that water level, flooding duration, inundation frequency, and topography were associated with the natural regeneration of A. germinans (Fig. 3).
Table 3 Results of the redundancy analysis (RDA) according to components RDA1 and RDA2 of the environmental variables. The values in bold indicate the variables that contributed the most to natural regeneration.
Variable | RDA1 | RDA2 | Site | RDA1 | RDA2 |
Organic matter | 0.471 | 0.653 | Site 1 | -0.674 | 0.116 |
Bulk density | -0.596 | -0.394 | Site 2 | 1.353 | 1.248 |
Microtopography | -0.766 | 0.233 | Site 3 | 0.677 | -1.702 |
Porewater salinity | 0.294 | 0.661 | Site 4 | -1.356 | 0.338 |
Water level | -0.734 | 0.280 | |||
Flooding duration | 0.765 | -0.220 | |||
Frequency of inundation | 0.761 | -0.241 |
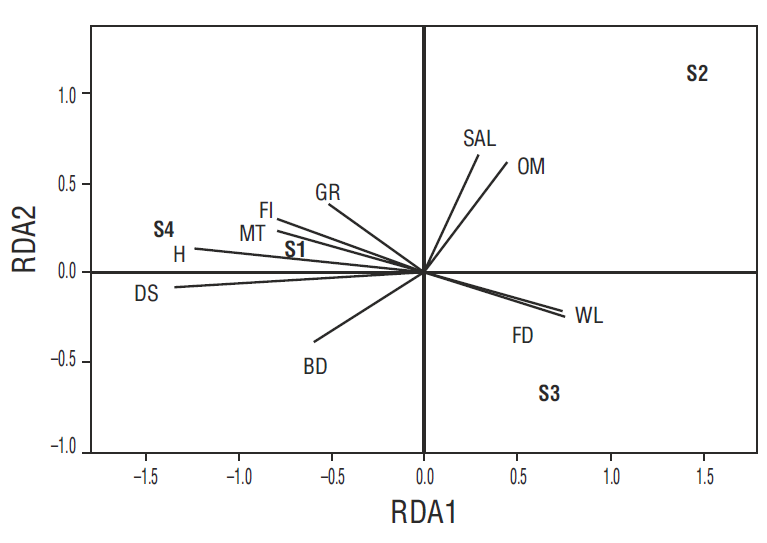
Figure 3. Redundancy analysis (RDA) plot according to components RDA1 and RDA2 of the environmental variables: bulk density (BD), organic matter (OM), microtopography (MT), porewater salinity (SAL), water level (WL), flooding duration (FD), and frequency of inundation (FI). The biological variables are seedling density (DS), height (H), and growth rate (GR). S1, S2, S3, and S4 refer to sites 1-4, respectively.
Discussion
Ecological restoration through hydrologic rehabilitation (desilting of tidal channels) and modification of the topography restored the environmental conditions at the sampling sites. The input of water to the restored sites led to changes in hydroperiod and soil conditions. Hydroperiod (water level, inundation frequency, and flooding duration) was important as it influenced the transport and settlement of propagules and the survival of mangrove seedlings.
The effect of the hydrological regime on mangrove regeneration in the restoration area indicated a differential trend. The establishment of A. germinans showed a patchy distribution, occurring only at the sites where the conditions were appropriate (Lewis 2005, Monroy-Torres et al. 2014, Spier et al. 2016). Not all the restoration area responded to the restoration actions at the same speed because the appropriate environmental conditions did not occur throughout the area. At the lower elevation site (-0.040 masl), where water remained for a longer period of time (680 ± 2.74 h) and the level was higher (0.28 ± 0.1 m), propagules were unable to settle and develop because of the reduced oxygen conditions that affect physiological functions, such as obtaining water and nutrients through the roots (Krauss et al. 2008). Neither did natural regeneration occur at the higher elevation site (0.24 masl) because the propagules transported by the tide were unable to settle due to the high soil bulk density (1.81 g cm-3) (Naidoo 2006).
The decrease in porewater salinity at all the sites was a determining factor for mangrove growth (Fig. 4). Porewater salinity decreased as a result of the increased frequency of flooding that “cleaned” the soil (inflowing water had a salinity of 38 and outflowing water a salinity of 46) and improved water exchange (Krauss et al. 2006). At the restored sites, we observed short (<70 cm), flowering mangroves in juvenile stages, with lateral shrublike branch growth. In contrast, plant heights of 1 m were observed at site 4 (reference). The shorter plant height is associated with high salinity and bulk density (Bashan et al. 2013, Monroy-Torres et al. 2014, Flores-Verdugo et al. 2015).

Figure 4. Interannual comparison of mean porewater salinity at the restored sites (1, 2, and 3), the reference site (4), and the higher and lower elevation sites.
Seedling density was higher at the reference site because of the presence of adult trees that produced propagules. Density at the restored sites, however, depended on the tide for propagule input, dispersion, and recruitment. This latter colonization process has been studied in other restoration areas where transport through tidal channels allowed seedling establishment (Lewis 2005).
Plant survival was greater at the restored sites (74%) than at the reference sites (29%). This difference can be attributed to 2 biological interactions of black mangrove seedlings. The plants at the reference sites showed a negative interaction associated with density-dependent mortality (Chu et al. 2010). Conversely, the plants at the restored sites showed a positive or facilitative interaction because seedlings benefit from their patchy distribution (Vogt et al. 2014), which improves nutrient availability (Corbin and Holl 2012), oxygenation, and soil elevation via root production (Milbrandt and Tinsley 2006).
For the natural regeneration of A. germinans, the optimum topographic elevation was 0.087 masl. In the case of hydroperiod, the optimum values were 8 floods per month, a flooding duration of 529 h m-1, and a water level of 0.14 m.
Our findings show that the topographic gradients and hydroperiod characteristics in the restoration area were the most important environmental controllers of the natural regeneration of A. germinans. The restoration activities (modification of the microtopography and reestablishment of the hydroperiod) helped to reduce salinity, reestablish water flow and propagule dispersion, and contributed to the development of mangrove seedlings and juveniles. This study contributes to the knowledge of environmental regulators and the dynamics of mangrove forest development in restoration projects.