Highlights:
Hot water treatment induced tolerance to chilling injury in “Tommy Atkins” mango.
Sequential hot water treatment improved cell membrane stability.
Sequential hot water treatment markedly influenced the activity of SOD, CAT and APX enzymes during chilling injury.
Introduction
Mango (Mangifera indica L.) is a tropical fruit, highly accepted by consumers due to its unique taste, aroma and attractive appearance. This fruit is produced and marketed throughout the world. Mexico is the world’s sixth largest producer but is the leading exporter, especially to Canada, the United States of America, and Japan (Food and Agriculture Organization [FAO], 2021). For marketing, mango is stored at critical temperatures (10-13 °C) because in its pre-climacteric stage it is highly susceptible to chilling injury (CI) (Chongchatuporn, Ketsa, & van Doorn, 2013; Zhang et al., 2017), a physiological disorder that is observed when fruit is stored below the critical temperature.
The susceptibility to CI is related to the storage time and physicochemical quality of the fruit (López-López et al., 2018; de Mello-Vasconcelos et al., 2019). Changes in the components of the membrane and its permeability are the first responses to CI at the cellular level, while oxidative stress is the second response (Zhang, Shen, Li, Meng, & Sheng, 2013; Shadmani, Ahmad, Saari, Ding, & Tajidin, 2015). The combination of these responses in “Tommy Atkins” mango results in symptoms like pitting or sunken lesions, peel browning, uneven ripening, internal discoloration, water soaking of the tissue, and fungal decay (Wang et al., 2008; Kim, Lounds-Singleton, & Talcott, 2009), and the symptoms can become more intense when fruit is stored at higher temperatures (Zhang et al., 2012).
Previous studies have reported that the use of oxalic acid (“Zill”; Li, Zheng, Liu, & Zhu, 2014), nitric oxide (“Kensington Pride”, Zaharah & Singh, 2011; Barman, Asrey, Pal, Jha, & Bhatia, 2014), gum arabic and calcium chloride (“Choke Anan”; Khaliq, Muda-Mohamed, Ghazali, Ding, & Ali, 2016), and hot water treatment (quarantine) alone (“Tommy Atkins”, Kim et al., 2009) or in combination with calcium chloride (“Keitt”, López-López et al., 2018; Díaz-Corona et al., 2020) reduce susceptibility to chilling temperatures in mango.
The use of moderate stress (heat or cold temperature) prior to storage at low temperature is a technology that helps fruit to reduce oxidative stress, activating the antioxidant system which includes enzymes like superoxide dismutase (SOD, EC 1.15.1.1), catalase (CAT, EC 1.11.1.6), ascorbate peroxidase (APX, EC 1.11.1.11), glutathione reductase (GR, EC 1.8.1.7), and peroxidase (POD, EC 1.11.1.7). It also increases heat shock proteins and antioxidant compounds such as polyphenols, carotenoids and ascorbic acid (López-López et al., 2018; Zhang et al., 2019). This system allows the defense mechanism to be prepared for an enhanced stress like cold storage and has positive effects on fruit quality by reducing respiration rate and increasing shelf life (Dea, Brecht, Nunes, & Baldwin, 2010; Nasef, 2018).
The mango export protocol requires the fruit to be subjected to a quarantine treatment to eliminate invasive pests (USDA-APHIS, 2014). The use of hot water as a quarantine treatment (46.1 °C/75 to 110 min; USDA-APHIS, 2014) allows fruit fly disinfestation and reduces lenticel darkening and anthracnose. Also, a quarantine treatment increases the activity of antioxidant enzymes (CAT, APX and POD) and reduces CI susceptibility in mango (López-López et al., 2018; Díaz-Corona et al., 2020). However, quarantine treatment can also accelerate ripening, increasing softening, water loss, sinking pulp in the peduncle area and poor color development, compromising the mango quality (Dessalegn, Ayalew, & Woldetsadik, 2013; Nasef, 2018).
On the other hand, the use of short-term hot water treatments (HWT) allows a delay in fruit ripening and softening, maintaining postharvest quality. Some of these beneficial effects have been reported in several fruits, such as “lisbo” lemon (Citrus limon [L.] Brum) (Safizadeh, Rahemi, & Aminlari, 2007), “Satsuma” mandarin (Citrus unshiu Marc) (Ghasemnezhad, Marsh, Shilton, Babalar, & Woolf, 2008), “Italica" broccoli (Brassica oleracea) (Zhang, Nakano, & Maezawa, 2009), “Baifeng” peach (Prunus persica Batsch) (Jin, Zheng, Tang, Rui, & Wang, 2009) and loquat (Eriobotrya japonica) (Shao & Tu, 2012). In mango (“Tainong 1” and “Tommy Atkins”), short-term heat treatment (55 °C, 10 min; 55 °C, 5 min) also increases CI tolerance and reduces quality loss, but does not control the fruit fly (Zhang et al., 2012; Almeida-Miguel, Durigan, Marques, Ascari Morgado, & Ferraudo, 2016).
Some authors report that sequential heat treatments in “Tommy Atkins” (hot water at 36.5 °C for 75 min + 46.5 °C for 61 min) and “Tuu Shien” (hot water for 55 °C for 3 min + vapor heat at 46.5 °C for 40 min) mangoes previous to storage at low temperatures increases CI tolerance and reduces membrane damage and water loss, compared to the same treatments applied individually (Nyanjage, Wainwright, & Bishop, 1999; Le, Shiesh, & Lin, 2010), but these combinations do not control the fruit fly. In this sense, sequential heat treatments where quarantine treatment is included can have great benefits, taking advantage of the fact that quarantine hot water treatment ensures pest control and that it is a mandatory treatment for mangoes exported to the United States. The objective of this study was to determine the effect of sequential hot water treatments on CI incidence and antioxidant enzyme activity in “Tommy Atkins” mango during storage at 5 °C (85-90 % relative humidity) and ripening.
Materials and methods
Plant material, treatments and experimental design
‘Tommy Atkins’ mangoes were obtained from an orchard located in Culiacan, Sinaloa, Mexico (24° 46’ 23’’ N and 107° 32’ 56’’ W, at 26 m a. s. l.). The fruits were harvested from trees 15 and 20 years old located in sandy or clay soils, warm weather (30-34 °C). The plants were maintained with drip irrigation before the rainy season. The fruits were selected in stage 2 (8-11 °Brix) (Crisosto et al., 2009) with absence of physical damage and a weight range of approximately 350 to 420 g, and transferred to the Physiology and Technology Postharvest laboratory at the Autonomous University of Sinaloa, Mexico.
Fruits were cleaned by dipping in a solution of 300 (L·L-1 sodium hypochlorite for 3 min, air dried and randomly divided into four groups. Each group received one of the following treatments: control (immersed in water at room temperature), HWT1 (dipping in water at 46.1 °C for 75 [USDA-APHIS, 2014]), HWT2 (dipping in water at 55 °C for 5 min [Ghasemnezhad et al., 2008]), and HWT1 + HWT2 (sequential treatment under the same aforementioned conditions, applied one after the other).
The HWT were carried out using a water bath (1266-02, Cole-Parmer®, Thermo Scientific, USA) with continuous hot water recirculation. Untreated mangoes (immersed in water at room temperature) were used as the control. Fruits of each treatment were stored at 5 °C (85-90 % relative humidity) for 15 and 30 days. After storage for 30 days at 5 °C, the fruits were exposed to ripening for 4 and 8 days at 21 °C ± 1 °C.
Parameters evaluated
Chilling injury index
The chilling injury index (CII) was determined according to López-López et al. (2018). The evaluations were made once fruits were removed from each storage. Symptoms of CI were visually assessed and included lenticel darkening (L), pitting (P), uneven color development (U), and decay (D). The severity (S) of CI symptoms was evaluated with a five-point visual scale (0 = no tissue injured, 1 = 1 to 25 % of tissue injured, 2 = 26 to 50 % of tissue injured, 3 = 51 to 75 % of tissue injured, and 4 = more than 76 % of tissue injured). Five fruits were used per day and treatment. The CII was calculated by the following formula:
where SL is the severity of lenticel darkening, SP is the severity of pitting, SU is the severity of uneven color development, and SD is the severity of decay.
Electrolyte leakage
For electrolyte leakage (EL), ten mesocarp discs (7 mm in diameter and 5 mm in height) were obtained from each fruit with a cork borer (Malacrida, Valle, & Boggio, 2006). The discs were washed three times with deionized water, then placed in 25 mL of 0.4 M mannitol solution and incubated for 2 h at 25 °C with continuous shaking (150 rpm). Electrical conductivity (S·m-1) readings of the solution were taken with a conductivity meter (Hanna Instruments®, HI98311, South Africa) after incubation (initial electrical conductivity) and after the discs were autoclaved at 121 °C for 20 min and cooled at 25 °C (total electrical conductivity). Five fruits were used per day and treatment. The percentage of EL was calculated with the following formula:
Determination of malondialdehyde
The final product of lipid peroxidation is malondialdehyde (MDA) and was determined by the thiobarbituric acid (TBA) technique according to Hodges, de Long, Forney, and Prange (1999). Briefly, 1 g of fresh tissue and 30 mL of ethanol:water (80:20 v/v) were homogenized and centrifuged at 3,000 g (4 °C, 10 min). Two solutions were prepared: 1 mL of supernatant plus 1 mL of solution A (-TBA) and 1 mL of supernatant plus solution B (+TBA). Both solutions contained 20 % trichloroacetic acid (TCA) + 0.01 % butylated hydroxytoluene (BHT), and solution B also contained 0.65 % TBA. All samples were shaken, heated at 95 °C for 25 min, cooled and centrifuged (3,000 g) at 25 °C for 10 min. Absorbance readings were taken at 440, 532 and 600 nm using an UV-Vis spectrophotometer (SQ2800, Unico, USA). The concentration of MDA equivalents was calculated using the following formula:
Weight loss
The weight loss (WL) was determined individually in nine fruits (Concellón, Zaro, Chaves, & Vicente, 2012). The weight was recorded on the initial day and then every 3 days during storage at 5 °C. The percentage of WL was calculated as follows:
where Wi represents the initial weight and Wf represents the final weight.
Color
This parameter was measured using a colorimeter (CR200, Minolta, Japan) based on the CIELAB color system and calibrated with a white plate. The measurements were made in three equidistant points on the equatorial axis of each fruit peel, and the values of luminosity (L*) and hue angle (°Hue) were recorded.
Firmness
Firmness was evaluated with a digital penetrometer (Chatillon DFE 100, AMETEK®, USA) fitted with an 11 mm diameter stainless steel cylinder probe. The fruit peel was removed and the mesocarp was penetrated (5 mm depth) at six different points with a constant speed of 50 mm·min-1. The results are expressed in Newtons (N).
Oxidative metabolism (enzymatic activity)
Superoxide dismutase (SOD, EC 1.15.1.1). The enzymatic activity of SOD was determined according to López-López et al. (2018). A freeze-dried tissue sample of 0.5 g was homogenized with 5 mL of 0.05 M potassium phosphate buffer (pH 7.5) + 1 mM ethylenediaminetetraacetic acid (EDTA) + 5 % polyvinylpyrrolidone (PVP). The mixture was centrifuged for 15 min (17,200 g at 4 °C) and the supernatant was recovered as enzymatic extract. The reaction mixture included 1 (L of enzymatic extract plus 199 (L of 0.1 M potassium phosphate buffer (pH 7.8) which contained 0.025 % Triton X-100, 0.01 M methionine, 0.11 M EDTA, and 57 (M nitro blue tetrazolium chloride (NBT), plus 50 (L of 20 (M riboflavin (final volume 250 (L). The absorbance at 550 nm was recorded before and after the light exposition (25 W) for 10 min, using a microplate reader (The Synergy™ HT Multi-Detection, Bio-Tek®, USA).
SOD activity is expressed in units of enzymatic activity per milligram of protein (U·mg-1 protein). One unit of SOD activity was defined as the amount of enzyme that would inhibit 50 % of the NBT photoreduction. Proteins were quantified using the Bradford (1976) assay.
Catalase (CAT, EC 1.11.1.6). To analyze CAT activity, 0.5 g of freeze-dried tissue was homogenized with 5 mL of extraction buffer (0.045 M potassium phosphate [pH 7.0] + 5 mM dithiothreitol + 5 mM EDTA + 0.125 g of polyvinylpolypyrrolidone). The mixture was centrifuged at 17,200 g and 4 °C for 30 min. The supernatant was recovered as enzymatic extract. CAT activity was determined by measuring the disappearance of hydrogen peroxide (H2O2) (López-López et al., 2018). The reaction mixture contained 1 mL of 0.04 M potassium phosphate buffer (pH 7.0) plus 0.04 M H2O2 and 25 (L of enzymatic extract. The changes in H2O2 concentration during 5 min were calculated based on its extinction coefficient (43 M-1·cm-1) at 240 nm. The results are expressed in U·mg-1 protein.
Ascorbate peroxidase (APX, EC 1.11.1.11). The APX activity was determined using the same enzymatic extract obtained for CAT. The reaction mixture contained 969 (L of 40 mM potassium phosphate buffer (pH 7.0) plus 5 (L of 0.1 M ascorbic acid and 1 (L of 0.1 M H2O2 and 25 (L enzymatic extract (López-López et al., 2018). The activity is expressed as U·mg-1 protein. The changes in ascorbic acid concentration were calculated based on its extinction coefficient (2.8 mM-1·cm-1) at 290 nm.
Statistical analysis
Data were subjected to a two-way analysis of variance (ANOVA) using Stat-graphics Plus 5.1 software (Statistical Graphics, Rockville, MD). The effect of treatments (control, HWT1, HWT2 and HWT1+HWT2) and days of storage (0, 15, 30, 30+4 and 30+8 days) were the factors analyzed. Significant differences (P < 0.05) between means were established using Fisher’s least significant difference (LSD) test. Unless another number is indicated, three replicates with five repetitions were used for the analysis.
Results and discussion
Chilling injury index
The application of HWT and storage time had a significant effect on the presence of CI symptoms like pitting, uneven color development and decay in “Tommy Atkins” mango (Figure 1). The application of HWT1, HWT2 or HWT1 + HWT2 significantly reduced the susceptibility to CI compared with the control after 30 days at 5 °C (Figure 1a). When fruits were transferred at ripening, the presence of symptoms increased as the storage period progressed (30+8 days) (Figure 1b). Mangoes treated with HWT2 and HWT1 + HWT2 resulted in a lower CII (1.72). When doing a linear regression analysis, HWT2 showed the lowest rate in the appearance of symptoms during storage at 5 °C (linear term coefficient of 0.053, coefficient of determination of R2 0.999), while HWT1 + HWT2 presented this behavior during storage at 21 °C (linear term coefficient of 0.080, coefficient of determination of R2 0.955). This result is related to postharvest heat treatment, since it generates physiological and biochemical changes in the fruit. These changes are related to factors such as the type of heat treatment, the exposure time and the fruit genotype (Ummarat, Matsumoto, Wall, & Seraypheap, 2011).

Figure 1 a) Chilling injury index (CII) of “Tommy Atkins” mango during storage at 5 °C plus 4 and 8 days at 21 °C, and b) representative images of mango from each treatment after 30 days at 5 °C plus 8 days at 21 °C. HWT = hot water treatments. Data are the mean of five replicates with Fisher’s least significant difference (LSD = 0.17) as vertical bars. Different letters show significant differences among treatments (Fisher, P < 0.05).
The increase in CI tolerance favored by an individual short or long heat treatment has been previously reported in “Tuu Shien” and ‘Keitt’ mango (Le et al., 2010; Díaz-Corona et al., 2020), “Hom Thong” banana (Ummarat et al., 2011) and “Hongyang” kiwifruit (Ma et al., 2014). Also, this tolerance was observed when sequential heat treatment was applied in “Frangi” papaya (Shadmani et al., 2015). The ability of HWT2 and sequential treatment to reduce CII can be attributed to the maintenance of cell membrane integrity (less MDA content) and a reduction in membrane permeability (less EL; Figure 2a), as well as an increase in the enzymatic antioxidant system that protects cells from oxidative stress in fruit subjected to HWT1 + HWT2.
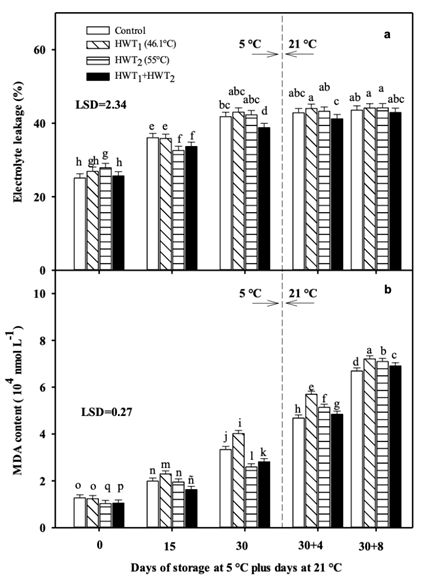
Figure 2 a) Electrolyte leakage and b) malondialdehyde (MDA) content in “Tommy Atkins” mango during storage at 5 °C plus 4 and 8 days of ripening at 21 °C. HWT = hot water treatments. Data are the mean of five replicates with Fisher’s least significant difference (LSD = 0.17) as vertical bars. Different letters show significant differences among treatments (Fisher, P < 0.05).
Also, the reduction in CI susceptibility in HWT1 fruit with respect to the control was related to higher enzymatic activity, but not to a reduction in cell membrane damage, since HWT1 presented higher MDA and EL values than the control. Thus, the use of sequential heat stress allows a greater activation of the antioxidant system in the fruit, priming it for greater stress such as long-term cold stress (30 days at 5 °C).
Electrolyte leakage and malondialdehyde
The EL increased in different proportions during storage at 5 °C and room temperature for all treatments. At day 15, mango treated with HWT2 and HWT1 + HWT2 presented lower EL than HWT1 and the control, while after 30 days of cold storage, only the HWT1 + HWT2 treatment maintained the lowest EL. However, during storage at 21 °C, all treatments presented similar EL (Figure 2a). In regression analysis at 5 °C, HWT1 + HWT2 showed the lowest rate of electrolyte release, obtaining a linear term coefficient of 0.437 (coefficient of determination of 0.983), while, contrary to what was observed in the analysis of variance at 21 °C, HWT1 presented the lowest rate in EL (linear term coefficient of 0.141, coefficient of determination of R2 0.826).
On the other hand, MDA content increased with storage time at both storage temperatures (Figure 2b). Fruit treated with HWT2 showed the lowest MDA content at the end of storage at 5 °C, followed by fruit of the sequential treatment, while the application of HWT1 promoted a higher MDA content, even over the control. During ripening, fruit treated with different HWTs presented higher MDA content than the control, but the use of HWT1 + HWT2 allowed lower lipid peroxidation. Similar behavior was observed in linear regression analysis, as HWT2 presented the lowest MDA production rate with a regression coefficient of 0.052 and coefficient of determination of R2 0.989 at 5 °C. On the other hand, at 21 °C, this analysis showed that HWT1 presented the lowest MDA production rate with a regression coefficient of 0.398 and coefficient of determination of R2 0.999, which differs from the analysis of variance possibly due to variability in MDA production.
The abiotic stress produced by low temperatures promotes oxidative damage in fruit and fast senescence. Therefore, accumulation of reactive oxygen species (ROS) in cells due to stress can result in membrane deterioration and an increase in EL and MDA content (Nasef, 2018). The use of HWT2 and HWT1 + HWT2 protected the cell membrane, reducing the levels of EL and MDA during cold storage. However, after removing from 5 °C, HWT fruit showed significant increases in these parameters. Similar results were previously reported in different fruits (pomegranate, mandarin, banana, and cucumber) treated with HW for a short time (40 to 55 °C, 4 to 10 min), where heat treatment reduced membrane damage (Mirdehghan et al., 2007; Ghasemnezhad et al., 2008; Ummarat et al., 2011; Nasef, 2018).
Nasef (2018) reported that heat treatment (55 °C for 5 min) alleviated CI in cucumbers due to a reduction in EL and retention of membrane integrity. Likewise, the behavior of the sequential treatment of the present study was similar to that of the combination of two HWTs (36.5 °C for 75 min plus 46.5 °C for 61 min) in “Tommy Atkins” mango stored at 4 and 9 °C, because the sequential heat treatment may delay ripening and softening, and thus reduce EL (Nyanjage et al., 1999). The individual application of heat treatments presented similar EL to the control. This increase in cell membrane permeability is attributed to the effects of heat stress, as it induces the activation of heat shock proteins, the arginine pathway, and glucose metabolism. Furthermore, it increases antioxidant enzymes and antioxidant compounds such as ascorbic acid and glutathione, which inhibit peroxidative damage (Zhang et al., 2019).
Weight loss
All treatments presented an increase in WL during storage at 5 °C, reaching values ranging from 2.98 % for HWT1 + HWT2 up to 3.92 % for HWT1 at the end of storage (Figure 3). Mango treated with a sequential treatment resulted in the lowest WL (2.98 %), showing significant differences (P < 0.05) with the fruit of the other treatments after 36 days at 5 °C. Interestingly, HWT1 and control fruit had similar values and resulted in the highest WL percentages. Likewise, this study revealed that HWT1 + HWT2 was more effective in reducing WL than the individual application of each heat treatment, which suggests that sequential heat treatment could reduce alterations in fruit peel, transpiration and respiration during cold storage, without inhibiting ripening when transferred to room temperatures (Shadmani et al., 2015).

Figure 3 Weight loss of Tommy Atkins mango during storage at 5 °C. Data are the mean of nine replicates. Vertical bars represent Fisher’s least significant difference (LSD) = 0.33.
The application of sequential heat treatment in mango could increase the barrier properties of cuticle and epicuticular wax, maintaining the integrity of the tissue (Shadmani et al., 2015). Contrary to these results, “Kensington” mango subjected to sequential treatment for a long time (vapor heat, 40 °C for 8 h plus HWT, 47 °C for 15 min) and stored at ripening temperature showed increased WL (2.29 %), a higher respiration rate, accelerated ripening (11.43 N in firmness, 13.92 °Brix, 0.96 titratable acidity), and increased transpiration and dehydration, compared to control fruit (Jacobi, Macrae, & Hetherington, 2000). On the other hand, Nasef (2018) reported that WL of cuccumbers was affected by treatment temperature (45 or 55 °C for 5 min), storage period (7, 14, 21 days at 5 °C plus 2 and 4 days at 20 °C), and their interaction. The use of 55 °C for 5 min could increase the barrier functions of the cell membrane and cell wall, including during ripening. The use of quarantine treatment plus short-term heat treatment has not been previously reported.
Color
Color parameters (L* and Hue angle) are presented in Table 1. Fruit showed an initial bright red and green coloration with average values of 41.18 and 18.23 for L* and °Hue, respectively. Storage time had a significant effect on loss of luminosity (-9.67 % on average), regardless of the treatment, which was maintained when the fruits were transferred to ripening at 21 °C in HWT1 + HWT2 and the control. HWT2 showed the lowest reduction in L* values after 30 days of cold storage, and after 8 days of ripening with respect to the other treatments. The treatments HWT1 and HWT1 + HWT2 showed the highest loss in L* values during cold storage (-13.91 %) and ripening (-16.51 %), respectively.
Table 1 Effect of the individual and sequential application of two different hot water conditions on luminosity, hue angle and firmness during storage of “Tommy Atkins” mango.
Treatments | Days of storage at 5 °C + days at 21 °C | ||
---|---|---|---|
0 | 30+0 | 30+8 | |
Luminosity | |||
Control | 41.18 ± 0.46 aB | 36.84 ± 1.24 bB | 34.74 ± 0.97 cC |
HWT1 | 42.41 ± 1.02 aA | 36.51 ± 0.09 bB | 36.95 ± 0.86 bB |
HWT2 | 40.62 ± 0.54 aB | 39.22 ± 1.04 bA | 38.66 ± 1.01 bA |
HWT1 + HWT2 | 43.06 ± 1.00 aA | 38.40 ± 0.35 bA | 35.95 ± 0.26 cBC |
Hue angle | |||
Control | 18.23 ± 1.70 aB | 17.28 ± 0.82 aB | 14.96 ± 1.04 bC |
HWT1 | 18.81 ± 1.45 aAB | 17.33 ± 0.23 bB | 17.01 ± 0.80 bB |
HWT2 | 19.85 ± 0.71 aA | 18.98 ± 1.07 aA | 19.18 ± 0.96 aA |
HWT1 + HWT2 | 18.90 ± 0.89 aAB | 18.28 ± 1.01 aAB | 17.36 ± 1.69 aB |
Firmness (N) | |||
Control | 135.68 ± 3.75 aAB | 112.95 ± 3.24 bA | 29.70 ± 3.99 cC |
HWT1 | 137.08 ± 4.18 aA | 104.07 ± 4.66 bB | 29.33 ± 0.87 cC |
HWT2 | 133.60 ± 6.98 aB | 101.80 ± 4.78 bB | 47.52 ± 2.70 cA |
HWT1 + HWT2 | 123.53 ± 4.72 aC | 97.67 ± 3.17 bC | 37.27 ± 1.96 cB |
For each parameter, different letters (a, b, c) in the same row (days of storage for each treatment) and different letters in the same column (A, B, C) (treatments in the same day) represent statistical differences according to Fisher’s least significant difference (LSD) test (P < 0.05). Values are the mean ( standard deviation. HWT1 = hot water treatment (46.1 °C, 75 min); HWT2 = hot water treatment (55 °C, 5min); HWT1 + HWT2 (same conditions, sequentially applied). 30+0 = 30 days of storage at 5 °C + 0 days at 21 °C, 30+8= 30 days of storage at 5 °C + 8 days at 21 °C.
On the other hand, a significant reduction (P < 0.05) in °Hue values was observed during cold storage in HWT1 fruit, while the values in the other treatments remained nearly constant. After an additional 8 days at 21 °C, only the control presented a significant reduction in °Hue (-13.42 %). The HWT2 and HWT1 + HWT2 preserved mango quality and maintained good appearance and color (luminosity and °Hue). This behavior can be associated with a reduction in CI symptoms and WL, stabilization of the cell membrane and a delay in ripening that in combination reduced the changes in peel color (López-López et al., 2018).
Similar results were previously observed in “Dahshan” cucumber, because the use of HWT for 5 min maintained a good color and appearance which was attributed to a delay in chlorophyll degradation by reduction of chlorophyll oxidase and peroxidase activities, induction of heat shock protein, stabilization of cellular membrane, and reduction of WL (Nasef, 2018). Likewise, the peel color index did not change significantly in mango immersed in heat treatments for a long time (75 min, 20 min) (Dea et al., 2010; Alvindia & Acda, 2015) or sequential heat treatment (3 min, plus 40 min) (Le et al., 2010), but they do when exposed to irradiation (Cruz, Soares, Fabbri, Cordenunsi, & Sabato, 2012).
Firmness
In relation to firmness, all treatments presented a significant reduction during storage at 5 and 21 °C (Table 1). Initial firmness values ranged from 123 to 137 N, and after 30 days of cold storage varied from 112.95 to 97.67 N. Interestingly, control fruit showed the lowest decrease in firmness (-16.75 %). The application of HWT1 in mango promoted the highest loss of firmness (-24.08 %), followed by HWT2 (-23.80 %) and the sequential treatment (-20.93 %). However, when the fruits were transferred to ripening, those of HWT2 showed the lowest loss of firmness, followed by HWT1 + HWT2, while the control and HWT1 fruits showed higher loss of firmness without significant differences (P > 0.05) between them.
The use of HWT1 + HWT2 caused a significant reduction in firmness compared with the treatments separately at the beginning of the study, which could be due to an increase in respiration rate and ethylene synthesis that cause degradation of starch into sugars, or to an activation of degradative enzymes that affect the cell wall (Hossain, Rana, Kimura, & Roslan, 2014). Although the HWT1 + HWT2 treatment had the lowest firmness values at the end of the storage period at 5 °C, it also presented the least change with respect to the initial day. Therefore, when sequential heat treatment is combined with storage at cold temperatures, cell wall degradation is reduced. According to Mirdehghan et al. (2007), a double stress could cause less depolymerization and solubilization of pectic substances (polyuronide) leading to reduce soluble pectin and ripening, as well as a decrease in the compression strength and enzymatic activity maintaining cell integrity and lower loss of firmness and cell decay (Le et al., 2010; Mahto & Das, 2013).
During ripening, the immersion of fruit in HWT2 reduced softening at day 30 at 5 °C plus 8 days at 21 °C, which could be associated with the short immersion time that had no effect on the activity of the enzymes polygalacturonase and (-galactosidase (Chávez-Sánchez, Carrillo-López, Vega-García, & Yahia, 2013). Similar results with sequential treatments were reported by Jacobi et al. (2000) in “Tuu Shein” mango treated with hot air for 8 h plus HW for 15 min, and Le et al. (2010) in mango treated with HW plus vapor heat for 40 min, where the authors observed reduced softening due to less cell wall degradation and changes in soluble pectin content.
Oxidative metabolism (enzymatic activity)
SOD activity increased for all treatments during storage at 5 °C. At day 30, the sequential treatment showed the highest activity followed by HWT1, while HWT2 and the control showed lower SOD activity without significant differences (P > 0.05) between them (Figure 4a). At day 30+8, all treatments presented a significant reduction in SOD activity; however, HWT1 + HWT2 maintained the highest activity with significant differences with respect to the other treatments. SOD enzyme contributes in the initial step of eliminating ROS and catalyzes the transformation of superoxide ion (O2 -) to H2O2 (Alscher, Erturk, & Heat, 2002; Soleimani-Aghdam, Jannatizadeh, Sheikh-Assadi, & Malekzadeh, 2016). In this study, the application of HW for a long time (HWT1 and HWT1 + HWT2) enhanced SOD activity, however, the sequential treatment presented higher activity at both storage temperatures (5 and 21 °C).

Figure 4 Antioxidant enzymatic activity in mango “Tommy Atkins” during storage at 5 °C (continuous lines) plus 4 and 8 days at 21 °C (dotted lines): a) superoxidase dismutase (SOD), b) catalase (CAT), and c) ascorbate peroxidase (APX). Data are the mean of five replicates with Fisher’s least significant difference (LSD) as vertical bars.
The HWT2 did not show any effect on SOD activity due to low membrane damage and reduced ROS content. These results suggest that the effect of HWT1 + HWT2 on the reduction of CI can be associated with an increase in SOD activity which prevented the accumulation of O2 - and therefore oxidative damage, decreasing EL and MDA content during cold storage. No significant differences were observed in SOD activity between HWT2 and control fruit. Contrary to this result, Fogelman, Kaplan, Tanami, and Ginzberg (2011) and Wang et al. (2016) reported that the increase in SOD activity in “Hami” melon was due to the effect of immersion in HW at 59 °C for 30 s plus 55 °C for 3 min; however, SOD activity did not have an effect on CI reduction, since it increased the content of O2 - and H2O2 which led to lipid peroxidation and membrane damages.
On the other hand, the results obtained in this study were similar to those reported by López-López et al. (2018) in “Keitt” mango immersed in quarantine treatment and stored at 5 °C, since an increase in SOD activity was related to CI tolerance and reduced membrane damage. Moreover, the use of sequential HWT (42 °C for 30 min plus 49 °C for 20 min) in “Frangi” papaya did not significantly increase SOD activity during storage at 6 °C; therefore, it did not correlate with CI tolerance (Shadmani et al., 2015). This is contrary to our results with sequential treatment, and can be associated with the differences in exposure time at both temperatures.
In a similar mode, HWT1 + HWT2 and HWT1 presented higher CAT activities during cold storage, showing increases of 38.80 and 27.67 %, respectively, at day 30 (Figure 4b). HWT2 and the control showed lower activity (19.80 and 11.68 %, respectively). When the fruits were transferred at 21 °C, those treated with HWT1 had the maximum reduction in CAT activity at day 30+4, while the fruit treated with HWT1 + HWT2 maintained the highest activity with significant differences with respect to the other treatments. At the end of ripening storage, CAT activity was reduced in all treatments and no significant differences were observed among them. CAT reduces H2O2 to water and oxygen, protecting the cell membrane of oxidative damage (Nasef, 2018).
CAT activity showed similar behavior to SOD activity. HWT1 and HWT1 + HWT2 treatments presented a significant increase in CAT activity at the end of storage at 5 °C; the quarantine treatment can induce oxidative stress, but it also induces oxidative stress defense (López-López et al., 2018). HWT2 induced minimum CAT activity, similar to the control, but with the difference that HWT2 reduces membrane damage and ROS production. During ripening, the increase in CI symptoms was associated with reduced CAT activity and accumulation of H2O2, a molecule that easily passes through the cell membrane and reacts with intracellular metal ions, producing hydroxyl radicals that cause damage in protein and DNA structure and also loss of membrane integrity (Sala & Lafuente, 2000).
HWT1 + HWT2 maintained the highest activity during the first four days at ripening as observed for SOD activity. Both enzymes help to reduce H2O2 and O2 - production limiting hydroxyl radical intracellular and CI symptoms. In agreement with this, different authors indicate that heat treatment increases CAT activity and reduces the accumulation of H2O2 protecting the cell from damage and CI symptoms, with even CAT presenting higher antioxidant effect than APX, an enzyme that competes for H2O2 during cold storage (Fogelman et al., 2011; Nasef, 2018). On the other hand, Shadmani et al. (2015) report that sequential HW in papaya promoted less CAT activity than the control at 6 °C, especially after two weeks. They observed that H2O2 accumulation in control fruit was correlated with higher CAT activity, and hence, it was not observed that higher CAT activity correlated with CI tolerance in HWT fruit (Shadmani et al., 2015).
Regarding APX activity, this increased during cold storage in fruit with different HWTs, while control fruit did not show significant changes (Figure 4c). After 30 days at 5 °C, sequential treatment presented higher APX activity with significant differences with respect to HWT2 and the control. After 4 days at 21 °C, APX activity was reduced for all HWTs, but HWT1 + HWT2 maintained the highest values with respect to the other treatments. After 8 days at 21 °C, no significant differences in APX activity were observed between the control and all HWTs. In this work, the use of different HWTs increased APX activity, especially at the end of storage at 5 °C. During ripening, all treatments reduced APX activity in a similar way to the other enzymes (SOD and CAT), showing the highest activity with the sequential treatment.
Chongchatuporn et al. (2013) indicate that a reduction in CI susceptibility is related to a reduction in ROS and an increase in CAT and APX activities during cold storage. Similar results were previously observed in strawberry (Vicente, Martínez, Chaves, & Civello, 2006), broccoli (Zhang et al.,2009), banana (Ummarat et al., 2011), and mango (López-López et al., 2018), where a higher transcription and activity of APX were observed with respect to the control, reducing ROS accumulation and cell damage and increasing CI tolerance. A coordinated increase in the activities of antioxidant enzymes such as SOD, CAT, and APX promoted by sequential treatment is very important for scavenging ROS to protect the cell membrane, to induce CI tolerance and to maintain quality during cold storage.
Thus, the use of heat treatment for balance between antioxidant enzymes is critical for cell survival during cold stress (Zhang et al., 2013; López-López et al., 2018). It is important to highlight that the observed effect for sequential treatment (quarantine plus short-term) has not been previously reported for SOD, CAT and APX activities and CI tolerance in mango.
Conclusions
The application of hot water treatment previous to cold storage reduced chilling injury susceptibility in “Tommy Atkins” mango, especially in those treated with HWT2 (55 °C for 5 min) and in those with HWT1 + HWT2 (46.1 °C for 75 min plus 55 °C for 5 min) during ripening. HWT1 + HWT2 reduced EL, MDA and WL at 5 °C and activated the antioxidant system by increasing SOD, CAT and APX activities. HWT2 maintained color and firmness the best, followed by the sequential treatment. The combination of a quarantine treatment and a short-term heat treatment can be a useful alternative to maintain postharvest quality during storage and distribution of “Tommy Atkins” mango, complying with the pest control regulations required by USDA-APHIS (2014) and providing CI tolerance, which would facilitate its marketing to distant places. In addition, it would be interesting to try it on other cultivars susceptible to low temperatures and check its effectiveness.