Introduction
Changes in land use as a result of human activities are the leading cause of the loss of forests and biodiversity associated with them (Sebbenn, 2011; Toledo-Aceves, Meave, González-Espinosa, & Ramírez-Marcial, 2011; Agramont, Maas & Nava, 2012; Food and Agriculture Organization [FAO], 2016). The montane cloud forest is one of the natural systems recording the highest loss rate in Mexico (Toledo-Aceves et al., 2011). This forest system generates unique environmental conditions for the coexistence of temperate and Neotropical plant species, where endemic species grow (Challenger, 1998; Williams-Linera, 2007; Toledo-Aceves et al., 2011).
The estimated cover of these forests in Mexico ranges between 0.5% and 1% (Rzedowski, 1996; Jiménez, 2012). Chiapas, and particularly Los Altos region, has recorded an annual deforestation rate of 18.9%, caused mainly by illegal logging, grazing, and agriculture (González-Espinosa, Quintana-Ascencio, Ramírez-Marcial, & Gaytan-Guzman, 1991; González-Espinosa, Ochoa-Gaona, Ramírez-Marcial, & Quintana-Ascencio, 1995; Cayuela, 2006; González-Espinosa et al., 2007).
A conservation strategy for forested areas is the restoration and floristic enrichment of forest remnants, as well as the introduction of new genetic variants to increase genetic diversity (Frankham, Ballou, & Briscoe, 2002). Unfortunately, urban forests are reforested primarily with exotic species; however, based on the ecological knowledge of local forests, native species could also be incorporated. The introduction of new organisms to previously settled communities brings ecological consequences (e.g., competition, diseases) (Begon, Townsend, & Harper, 2006). Also, genetic factors may compromise the long-term viability of introduced populations, e.g., through inbreeding, deleterious alleles, and founder effect, all of which should be factored in during the introduction of organisms (Frankham et al., 2002; Freeland, 2005).
El Cerrito de San Cristóbal de Las Casas, Chiapas (hereafter called El Cerrito) is an urban forest developed by the afforestation of a volcanic mound some 60 years ago, by planting three species: Cupressus lusitanica, Pinus pseudostrobus, and Eucalyptus camaldulensis, the latter two being exotic species. Although C. lusitanica is considered to be native to Mexico, its biological properties restrain the growth of other plant species (Fernández-Pérez, Ramírez-Marcial, & González-Espinosa, 2013). This study determines the current diversity and propose floristic enrichment, and the genetic richness associated with it, taking this urban forest as an example of a conservation strategy for native cloud forest species.
Objectives
The specific objectives are: (1) describing the composition and floristic richness of El Cerrito de San Cristobal urban forest, (2) increasing tree diversity in El Cerrito with MCF native species from Los Altos de Chiapas (Chiapas Highlands) as a conservation strategy for these species, and (3) estimating the introduced genetic diversity in the cluster of species used for floristic enrichment.
Materials and methodos
Study area
El Cerrito is a promontory located in the central part of the valley of San Cristóbal de las Casas, Chiapas, seeming like an "island" of tree vegetation surrounded by human settlements and roads (Ruiz-Montoya, 2014). Its altitude ranges from 2128 m to 2190 m above sea level, stretching across 80 hectares approximately. It is located between 16°43'54.72"-16°44'08.38" N and 92°38'52.59"-92°38'24.52" E (Fig. 1).

FIGURE 1 Study area in El Cerrito, San Cristóbal de Las Casas, Chiapas, for the analysis of tree composition, floristic enrichment, and genetic diversity of 14 introduced tree species.
The first afforestation effort took place in the late 1960s to embellish the chapel dedicated to the patron saint San Cristóbal. These efforts hardly prospered, given the scarcity of soil and water. A new afforestation attempt was conducted in 1973 as an initiative of municipal and state government authorities, through the then Socio-Economic Development Program of the Chiapas Highlands (Prodech), with the participation of the local society. In that year, El Cerrito became public property; it was then that some 5000 plants of cypress (Cupressus lusitanica), eucalyptus(Eucalyptus camaldulensis), and Japanese privet (Ligustrum japonicum) were planted. Planting and maintenance activities were carried out mostly by citizens.
Tree Diversity.
Twenty 1000 m2 circular plots were established across the entire polygon of El Cerrito (Ramírez-Marcial et al., 2010). Within each plot, the diameter at breast height (DBH) was measured for all individual trees, both planted and grown naturally, which had a trunk ≥ 5 cm in diameter. The presence of shrub and herbaceous species was recorded in the entire area in July and August 2012 and 2013 through walks along tracks. Absolute and relative data on density, frequency, and basal area of each species were recorded.
Floristic enrichment
All introduced plants were grown in nurseries after thorough seed tree selection and seed collection processes, along with feasibility and planting analyses (Guillén, Luna & Ruiz-Montoya, 2014). Introduced plants were between two and four years old. Planting was conducted in 20 plots of 400 m2, distributed across El Cerrito slopes. Individuals of each species were assigned to plots according to local site conditions (microrelief, accumulated soil, radiation) and shade tolerance (Ramírez-Marcial, Camacho-Cruz & González-Espinosa, 2005; Ramirez-Marcial, Camacho-Cruz, González-Espinosa, & Lopez-Barrera, 2006; Ramirez-Marcial et al., 2008). Trees were planted at two time points during the rainy season: late June 2012 and early June 2013. Given the high ecological relevance of oaks (Quercus) in forests of Chiapas Highlands (González-Espinosa et al., 2012), we used a larger number of individuals of oak species.
The survival of the plantation was assessed in January 2014 by quantifying the number of live plants versus the total number of plants introduced in the two consecutive years. In addition, growth rate was determined by comparing the height and diameter at two time points - September 2012, one month after the end of the plantation, and January 2013 - in a random sample of individuals of the 2012 plantation. Plant height was measured from the plant base up to the highest part of the main stem; stem diameter was measured at 10 cm from the ground. The relative growth rate was calculated for the two variables (height and diameter) using the formula TCR = ln (M2-M1)/4, where TCR is the relative growth rate, M1 and M2 are the measurements recorded at times 1 and 2, respectively, and the denominator refers to the time elapsed (4 months) between times 1 and 2 (Wilson & Tilman, 1991).
Introduced Genetic Diversity
The genetic diversity introduced into the cluster of species managed was estimated by assessing genetic variation through Restriction Fragment Length Polymorphism (RFLP) of a universal chloroplast region; this allowed reducing time and costs associated with obtaining specific markers. We used the spacer region of the chloroplast genome (cpDNA) corresponding to exon 5’ of tRNL (UAA) and to exon 3' of tRNL (UAA; Taberlet, Gielly, Pautou, & Bouver, 1991), with genbank access number: B49317 (5’ CGAAATCGGTAGACGCTAGGG 3’) and A49855 (5’ GGGGATAGAGGGACTTGAAC 3’).
Two to three leaves were collected from 29 to 30 individuals, according to availability, of each of the 14 introduced species prior to planting, and were stored at -70 ºC in the Genetics Laboratory at El Colegio de la Frontera Sur (Ecosur). DNA was extracted with a modification of the CTAB 2X method described by Doyle and Doyle (1987). DNA amplification was performed using the Polymerase Chain Reaction (PCR). The amplification conditions used were initial denaturation at 94 ºC for 2 min, followed by 30 cycles at 94 ºC for 1 min (denaturation), 52 °C for 1 min (alignment), 72 °C for 1 min (extension), and 72 ºC for 7 min (final extension). The amplification products were visualized by electrophoresis in 2% agarose gels.
Once the DNA region of the target chloroplast was obtained, it was digested with eight restriction enzymes (Fermentas): HinfI, HaeIII, MspI, HhaI, TaqI, MboI, AluI, and DraI. The restriction reaction was conducted with 10 µl of nuclease-free water, 2 µl of buffer (Green Buffer), 0.7 µl of 10X enzyme, and 5 µl of the amplified product. Each reaction was incubated in a water bath at 37 °C for 90 min. The restriction fragments were separated by electrophoresis in 8% acrylamide gels. The patterns of bands obtained by electrophoresis were interpreted as suggested by Dumolin-Lapegue et al. (1997), where each polymorphic restriction fragment is considered a character and each state is encoded according to fragment size.
The following parameters were used as genetic diversity estimators: haplotype diversity (h), percent polymorphism (%P), expected heterozygosity (uh), and number of polymorphic sites (restriction fragments) (s); all were obtained using the program GenAlEx v. 6.51 (Peakall & Smouse, 2006). The genetic differentiation between species was estimated through an Analysis of Molecular Variance (AMOVA) and the fixation index (Fst), determined using the software Arlequin v. 3.5.1.2 (Excoffier, 2010). In addition, we obtained the Shannon’s genetic diversity index (SI) using the program MVSP v. 3.1 (Kovach, 2007).
The genetic similarity between species was examined through the analysis of Nei’s genetic distances (Nei, 1978) with the program GenAlEx v. 6.51 (Peakall & Smouse, 2006), depicted graphically applying the Neighbor-Joining method including 1000 replicates, with the program PAUP v. 4.0a147 (Swofford, 2002). In addition to the genetic diversity parameters, clade width (defined by genetic distances) was used as an indirect estimator of genetic diversity: a broader width is related to a lower genetic relationship, i.e., a greater genetic diversity due to phylogenetic remoteness (distance).
Results
Species Diversity
We identified six shrub, 11 tree, and 107 herb species. The most abundant species was C. lusitanica, representing nearly one-third of all trees (Appendix 1). Herbaceous species showed a high species richness (107), 68 being dicotyledons (63.55%), 24 monocotyledons (22.43%), and 15 ferns and lycophytes (14.02%). The most species-rich family was Asteraceae with 16 species, followed by Poaceae and Lamiacea with eight species, and Polypodiaceae with six species. The genus Polypodium (ferns) showed the largest number of species (Appendix 1). Species richness, density, and basal area were highly variable in all parcels evaluated, indicating a high heterogeneity in the composition and structure of the tree vegetation.
Floristic enrichment
The number of individuals planted in years 2012 and 2013 amounted to 2762 and 590, respectively, for a total of 3352 plantlets (186 ind/ha) across 8000 m2, with a mean survival of 45%. The species showing the highest percent survival one year after planting were Olmediella betschleriana (88%) and Myrsine juerguensenii (84%). The species with the lowest survival were Quercus sebifera (0%), Oreopanax xalapensis (10%), and Symplocos breedlovei (20%).
A low average growth was recorded both in height (0.001 cm/month) and in stem diameter (0.018 cm/month). Quercus rugosa displayed the highest growth rate (0.22 cm/month in height and 0.030 cm/month in stem diameter), while other species showed even a decrease in size (e.g., Styrax magnus, -0.079 cm/month in height; Q. laurina, -0.013 cm/month in stem diameter; Table 1).
TABLE 1 Growth rate (cm/month) and percent survival (%) of 14 native tree species introduced to El Cerrito de San Cristóbal de Las Casas, Chiapas, Mexico. - = no data obtained, N = Sample, S = survival.
Species | Growth | Survival | |||
N | Height | Diameter | No. of Individuals Planted | S | |
Alnus acuminata | - | - | - | 61 | 0.33 |
Arbutus xalapensis | - | - | - | 62 | 0.48 |
Myrsine juergensenii | 5 | 0.010 | 0.007 | 101 | 0.84 |
Olmediella betschleriana | 8 | -0.029 | 0.010 | 102 | 0.88 |
Oreopanax xalapensis | - | - | - | 50 | 0.10 |
Prunus barbata | 5 | -0.040 | 0.009 | 85 | 0.47 |
Prunus rhamnoides | 7 | 0.008 | 0.010 | 85 | 0.53 |
Symplocos breedlovei | - | - | - | 50 | 0.20 |
Styrax magnus | 4 | -0.079 | 0.011 | 102 | 0.54 |
Quercus crassifolia | 17 | 0.006 | 0.039 | 412 | 0.48 |
Quercus crispipilis | 18 | -0.001 | 0.022 | 907 | 0.44 |
Quercus laurina | 18 | -0.005 | -0.013 | 733 | 0.48 |
Quercus rugosa | 25 | 0.22 | 0.030 | 571 | 0.54 |
Quercus sebifera | 5 | 0.005 | 0.013 | 31 | 0.00 |
Average/total* | 0.001 | 0.018 | 3352* | 0.45 |
Introduced Genetic Diversity
The amplification fragment of the chloroplast spacer region included 600 base pairs (bp). After the digestion of fragments with restriction enzymes, we identified 20 restriction sites (loci) with HinfI, 23 with HaeIII, 11 with MspI, 20 with HhaI, 27 with TaqI, 21 with MboI, 14 with AluI, and 13 with DraI, for a total of 149 loci.
Styrax magnus showed the highest genetic diversity (s = 57, h = 0.13, %P = 46.31), followed by Prunus rhamnoides (s = 33, h = 0.08, %P = 30.87), M. juergensenii (s = 41, h = 0.08, %P = 27.52), and Olmediella betschelriana (s = 38, h = 0.09, %P = 25.50). Symplocos breedlovei was the species with the lowest genetic diversity (s = 17, h = 0.03, %P = 9.72) (Table 2). In general, mean genetic diversity was low (s = 30.5, h = 0.06, %P = 22.67; Table 2).
TABLE 2 Genetic diversity of 14 native tree species introduced to El Cerrito de San Cristóbal, Chiapas, Mexico, obtained with eight restriction enzymes of the tRNL region of chloroplast DNA (cpDNA).
Species | N | s | h | %P | SI | uh |
Alnus acuminata | 27 | 30 | 0.04 | 20.13 | 0.06 | 0.04 |
Arbutus xalapensis | 30 | 17 | 0.04 | 18.12 | 0.06 | 0.04 |
Myrsine juergensenii | 30 | 41 | 0.08 | 27.52 | 0.13 | 0.09 |
Olmediella betschleriana | 28 | 38 | 0.09 | 25.50 | 0.13 | 0.09 |
Oreopanax xalapensis | 30 | 23 | 0.04 | 22.82 | 0.07 | 0.04 |
Prunus barbata | 30 | 23 | 0.05 | 15.44 | 0.08 | 0.05 |
Prunus rhamnoides | 30 | 33 | 0.08 | 30.87 | 0.12 | 0.08 |
Symplocos breedlovei | 29 | 17 | 0.03 | 11.41 | 0.04 | 0.03 |
Styrax magnus | 30 | 57 | 0.13 | 46.31 | 0.20 | 0.13 |
Quercus crassifolia | 30 | 35 | 0.06 | 23.49 | 0.10 | 0.07 |
Quercus crispipilis | 30 | 18 | 0.03 | 12.08 | 0.05 | 0.03 |
Quercus laurina | 30 | 37 | 0.07 | 24.83 | 0.10 | 0.07 |
Quercus rugosa | 30 | 30 | 0.08 | 20.13 | 0.11 | 0.08 |
Quercus sebifera | 30 | 28 | 0.08 | 18.79 | 0.11 | 0.08 |
Average | 29.57 | 30.5 | 0.06 | 22.67 | 0.10 | 0.07 |
N = number of sequences analyzed, s = number of polymorphic sites, h = haplotype diversity, %P = percentage of polymorphism, SI = Shannon’s genetic diversity index, uh = expected heterozygosity [uh = 1 - (p 2 + q 2 )].
The analysis of molecular variance (AMOVA) showed that 67.21% of variation was found between species and 32.79% within species, with a fixation index Fst = 0.67, P = 0.001. The tree of genetic distances showed a clear grouping only for Quercus species, with Q. crassifolia and Q. crispipilis as the closest species. A genetic relationship was also found between Oreopanax xalapensis and Olmediella betschleriana, as well as between Symplocos breedlovei and Alnus acuminata. Prunus barbata was identified as the basal species (Fig. 2).
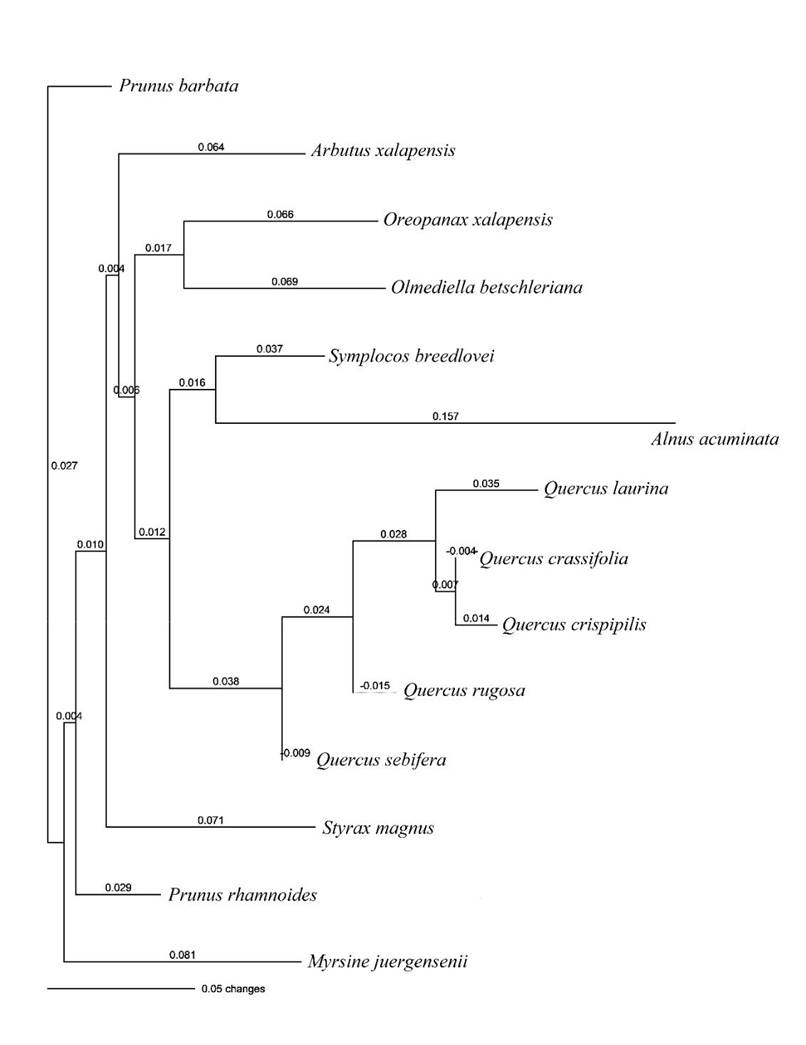
FIGURE 2 Tree of Nei’s genetic distances (Nei 1978) with 1 000 permutations for 14 tree species introduced to El Cerrito de San Cristóbal de Las Casas, Chiapas, based on eight restriction enzymes of the tRNL region of chloroplast DNA.
Discussion
Tree Diversity
As expected, the floristic composition analysis revealed that the forest is dominated by those species that were initially selected to be planted in a mound devoid of tree vegetation. This choice was likely based on the availability of plants produced at that time to supply the timber industry. The area was forested aiming to embellish a site with religious significance and, unintentionally, it is currently a landscape element that certainly serves to regulate temperature and probably captures atmospheric pollutants; at the same time, it is used as a leisure area for the local population and it also contributes to the conservation of native plant and animal species, especially birds (Mena & Silva, 2014), and insects (León-Cortés, Caballero, Miss-Barrera, & Giron-Intzin, 2019).
Despite the small number of tree species selected for the afforestation of El Cerrito 60 years ago, the current forest is characterized by heterogeneous vegetation composition and structure, albeit with a low diversity of native tree species. A null recruitment of seedlings and juveniles of the species that dominate the canopy was also observed. The low diversity of native species may be due to the fact that C. lusitanica prevents the establishment of other tree species (Fernández-Pérez, Ramírez-Marcial, & González-Espinosa, 2013). In addition, it is known that monospecific plantations lead to a low diversity of plants and animals, as well as to alterations of the properties of soil (e.g., acidification) (Cavelier & Tobler, 1998). In El Cerrito, soil pH is near-neutral (6.7-7.9) (Ruiz-Montoya, 2014), with a high cation-exchange capacity, suggesting suitable conditions for the establishment of plantlets of the 14 species introduced.
Floristic Enrichment
The introduction of native species is widely recommended for reforestation, ecological restoration, or forest enrichment, as this preserves the functionality and resilience of communities, which become better adapted to the current changing conditions (Thomas et al., 2014). All the species introduced to the El Cerrito urban forest are native to montane cloud forests of Los Altos de Chiapas, and, given their tolerance to high irradiation, are easily established in disturbed areas (Alcantara, Luna, & Velázquez, 2002; Ramírez-Marcial et al., 2012).
Enrichment increased the diversity of tree species in the early development stages (between two- and four-years post-planting) (Guarigata, Rheingans, & Montagnini, 1995; Jiménez 2012); an effective establishment is expected, so that subsequent growth should maintain the forest cover in the area in decades to come. However, a decline may occur because the local coexistence of introduced species depends on their specific tolerance to the prevailing environmental conditions in the site, as well as on those that may emerge as the forest develops (e.g., shade) (Quintana-Ascencio & González-Espinosa, 1993; Ramírez-Marcial, 2003; Quintana-Ascencio, Ramírez-Marcial, González-Espinosa, & Martinez-Icó, 2004).
In the short follow-up of the plantation, Q. rugosa showed the highest growth rate, likely because of its tolerance to moderate shade (Quintana-Ascencio, González-Espinosa, & Ramírez-Marcial, 1992; Bonfil & Soberón, 1999). On the other hand, five species (Olmediella betschleriana, Prunus barbata, Styrax magnus, Q. crispipilis, & Q. laurina) showed a decrease in height and stem diameter, maybe associated with water stress and damage caused by passers-by that cut off growing tips, thus negatively affecting their development and, ultimately, their establishment.
According to Galindo-Jaimes et al. (2002), Pinus-dominated forests in Los Altos de Chiapas are less fertile because of low organic matter and nitrogen in the soil; in addition, according to these authors, the establishment of many species and forest regeneration depend on a canopy dominated by Quercus. In this sense, our study recorded a 39% survival of Quercus spp. two years after planting, which supports a good prognosis for short- and long-term survival. However, it is suggested to continue planting native trees aiming to increase population density and species richness, as well as to monitor their establishment and growth.
Introduced Genetic Diversity
Planting native species for enrichment purposes increases species richness and, from a genetics perspective, enhances genetic flow and the founder effect (Glaubitz, Wu, & Moran, 2003; Li et al., 2005). The enrichment planting conducted at El Cerrito introduced previously absent genotypes and alleles, with populations that may show a low genetic diversity due to the founder effect, depending on the degree of diversity managed for the cluster of introduced species, and on the number of individuals with genetic variants that reached the reproductive stage.
To achieve a long-term development of introduced populations, it is recommended to manage high genetic diversity levels (Frankham et al., 2002; Glaubitz, Wu, & Moran, 2003; Stockwell, Hendry, & Kinnison, 2003). This study estimated genetic diversity with a molecular marker selected based on its universality, but the genetic variation recorded was low (%P = < 46.31, h = 0.13, s = < 41). These low genetic diversity levels are probably related to the collection of seeds from just a few parent trees, and to the chloroplast being a region of DNA of exclusively maternal inheritance with no recombination and a low evolutionary rate (Palmer and Stein, 1986), particularly as regards the tRNL region used in our study (7% variation in Symplocos; Fritsch, Cruz, Almeda, Wang, & Shi 2006).
The PCR-RFLP technique detects specific differences in DNA based on the presence or absence of fragments recognized by restriction enzymes (endonucleases); when the enzyme detects the specific unprotected (methylated) sequence, it cleaves the 4-6 nucleotide fragment. This produces fragments of variable length modified by DNA mutations, thus facilitating the comparison between genomes (Valadez & Kahl, 2000). Although this technique reveals the genetic variation based on the frequency of alleles (fragments), it involves only a relatively small DNA fraction; additionally, it does not identify heterozygous conditions, hence limiting the scope of the information obtained (Rentería-Alcántara, 2007). Therefore, future studies should include nuclear markers to obtain a complete estimate of genetic diversity (Rentería-Alcantára, 2007); also, a larger number of seed trees should be considered in future reforestation works.
Up to now, studies addressing the genetic diversity of wild populations of Quercus species are available for central and northern Mexico, yielding diversity estimates of 0.289 for Q. havardii based on the expected heterozygosity (He) with RFLP (Mayes, McGinley, & Werth, 1998). For the species covered in this study, it is known that genetic diversity levels of Q. crassifolia based on cpDNA ranges from 0.639 to 0.76 (Gorgonio-Ramírez, Clark, Campos, Monsalvo, & Alfonso, 2017), while the use of microsatellites yielded a He of 0.764; these studies suggest a high genetic diversity in oak species. We recommend exploring the genetic diversity of wild Quercus populations in montane cloud forests and contemplate such diversity in propagation and restoration programs of oak forests in Chiapas.
The genetic diversity of Oreopanax xalapensis in Los Altos de Chiapas has also been studied through enzymes, showing high genetic diversity and low genetic structuring at relatively small spatial scales (Ruiz-Montoya, Correa-Vera, Alfaro-González, Ramírez-Marcial, & Vallejo, 2011; Vera-Maloof, Ruiz-Montoya, & Ramírez-Marcial, 2019). Our results showed lower levels of genetic diversity than previously reported in Oreopanax xalapensis; possibly, the difference is due to the bias by sample size (low number of parent trees), so it is suggested to increase population genetics studies in native species of montane cloud forest trees.
As expected, the AMOVA showed the greatest percent variation between species, with a high and significant fixation index (Fst = 0.67), a finding that highlights the importance of each species in terms of the contribution to genetic variation introduced in El Cerrito. In addition, genetic distances identified Prunus barbata as the most evolutionarily conserved and differentiated species from all others (Weir, 1996; Week, Daly & Simpson, 2005). Since no previous information is available about the phylogenetic relationships of the species studied, the genetic distances between species reported in this document are valuable information for future phylogenetic analyses of tree species in montane cloud forests.
Conclusions
The results of this study support our proposal that urban forests are suitable for the conservation of native tree species. Planting native species improves forest richness and, in the case of El Cerrito, promotes the spatial continuity of montane cloud forests. The floristic enrichment of El Cerrito is a feasible strategy to boost the recovery of the forest community, i.e., improve habitat and microclimate conditions, thereby leading to the regeneration of a high diversity of tree species and local wildlife. The creation of forested areas including native species should be further promoted, in both rural and urban spaces, since it is one of the urgent actions needed to mitigate the effects of climate change.
The genetic diversity introduced by each individual species was relatively low, but nonetheless it was high at the community level, as assessed in terms of the distant phylogenetic relationships among species. If the plantation in El Cerrito urban forest progresses, it will likely translate into favorable environmental characteristics for the colonization of other species (Sebbenn, 2011).
This work shows that urban forests are a suitable approach for the conservation of native tree species, which in advanced stages may protect wild animal species and boost natural forest regeneration (De la Mora-Estrada et al., 2017; León‑Cortés et al., 2019; Lorenzo, Bolaños, García-Méndez, & Ruiz, 2014; Mena & Silva, 2014; Rao & Hodgkin, 2002; Ruiz-Montoya et al., 2014). Our results are relevant for ecological restoration and conservation of tree species of the montane cloud forest in urban forest systems, as well as for the knowledge of genetic diversity of tree species in Mexico.