1. Introduction
The notion of a tardy, low-diversity recovery from the Permian-Triassic mass extinction event has to be reconsidered following the paper by Brayard et al. (2017). Outline in that paper was an Early Triassic marine ecosystem with a phylogenetically diverse, functionally complex and trophically multi-level marine ecosystem, from primary producers to top predators and potential scavengers. Thus, ecosystem balance and optimisation were reached not long after the Palaeozoic Era. However, this was merely the first part of the transition from the Palaeozoic to the modern marine evolutionary fauna. A second, essential phase (undeveloped during the Palaeozoic) still had to take place, as demonstrated below. To encompass patterns of widespread evolutionary changes among benthic invertebrates in the marine realm during the Mesozoic (ca. 252 - 66 Ma), Vermeij (1987) coined the term ‘the Mesozoic Marine Revolution’. He focused mainly on extinct molluscs and observed a Mesozoic-Cenozoic radiation of durophagous predators. Another, coeval, revolution has been discussed by Knoll and Follows (2016), with reference to marine primary producers, inclusive of radiations of photosynthetic dinoflagellates, coccolithophorids and diatoms.
Mesozoic evolutionary and adaptive radiations in invertebrate groups such as echinoids (e.g., Kier, 1982; Smith, 1984; David et al., 2009; Kroh and Smith, 2010), heteromorph ammonoids (Mikhailova and Baraboshkin, 2009; Lehmann, 2015) and brachyuran decapod crustaceans (e.g., Karasawa et al., 2014; Schweitzer et al., 2016) have also been recorded in considerable detail. However, there was no link or overview in these palaeontological studies between the adaptive radiations observed and a coherent marine food chain revolution that filled an important portion of the modern marine ecosystem. The present paper demonstrates, for the first time, a bottom-up Mesozoic marine revolution model from phytoplankton to diverse nektonic and benthic invertebrate groups (Figure 1).
1.1. PHYTOPLANKTON
In order to gauge why several invertebrate groups experienced blooms and proceeded to occupy new ecological niches in Early Cretaceous marine assemblages, the food web at the time needs to be studied from the bottom up. In their bottom-up perspective on ecosystem changes in Mesozoic oceans, Knoll and Follows (2016) provided a mechanism for the observed evolutionary shifts in Mesozoic marine biota on the basis of a phytoplankton radiation and diversification (Figure 2).
Stratigraphically and taxonomically, planktonic microfossils provide the most complete record of biodiversity of any group of extinct marine organisms. The phytoplankton record is of particular significance as it tracked global changes in the marine system and, in turn, had an impact on biodiversity and productivity of higher trophic levels of the biosphere. Coccolithophores and other calcareous nannoplankton first appeared in strata of Late Triassic (c. 210 Ma) age. Rates of speciation at levels significantly above background were confined to the Late Triassic, Early Jurassic and Tithonian-Berriasian intervals (Bown et al., 2004). Another phytoplankton group, the dinoflagellates (single-celled protists at or near the base of the food chain), showed remarkable patterns in species diversity during the Mesozoic. First appearing in the Middle Triassic, they rapidly increased to a Late Jurassic maximum of 420 species in the Kimmeridgian (157 - 153 Ma). After a minor decline, diversity rose to an all-time peak of 584 taxa during the mid-Cretaceous (Albian; see MacRae et al., 1996). A third group of planktonic forms of importance comprises the enigmatic calpionellids. These marine organisms with calcitic, bellshaped tests ranged, particularly in more basinal environments, from the Tithonian to the Albian (Reháková and Michalík, 1997). Thus, from the Late Jurassic (Tithonian, 152 - 145 Ma) onwards a marked increase, both in numbers and overall diversity, of phytoplanktonic food items has been documented (Figure 3).

Figure 2. Radiations of species of photosynthetic coccolithophorids, dinoflagellates and diatoms (data from Knoll and Follows, 2016).

Figure 3. Radiation of zooplankton (planktonic foraminifera) related to the radiation of phytoplankton (modified after Bown et al., 2004, fig. 8).
1.2. ZOOPLANKTON
In modern seas and oceans distinct zooplankton responses to phytoplankton blooms have been recorded (e.g., Atkinson et al., 1996). In the fossil record there is a striking link between zooplankton (i.e., planktonic foraminifera and radiolarians) abundance and diversity and a phytoplankton increase from the Tithonian to the Late Cretaceous (e.g., Erbacher and Thurow, 1997; Premoli Silva and Sliter, 1999; Bown et al., 2004).
During the Late Palaeozoic and into the Mesozoic, Radiolaria experienced a gradual decline until the end of the Jurassic, at which time there was a rapid diversification. This coincided with a radiation amongst dinoflagellates which may have represented an increased source of food for the Radiolaria (Olney, 2002).
As a result of the increased, continuous ‘snowfall’ of planktonic remains to the sea floor in offshore settings, from the Tithonian onwards (e.g., Rückheim, 2005), large amounts of food particles were available to allow a radiation of small benthic organisms, collectively known as meiofauna, to take place.
1.3. HETEROMORPH AMMONITES
While heteromorph ammonites play almost no role during the earliest Cretaceous, they predominate in numerous faunal assemblages from the early late Hauterivian (c. 130 Ma) onwards, until the end of the late Maastrichtian (69 - 66 Ma) or the start of the early Paleocene. Although the link between the proliferation of planktonic groups appears quite obvious, many ammonite workers are still debating the diverse ecological constraints involved (Lehmann et al., 2015). Larger-sized invertebrates that benefited from the total plankton radiation were, first and foremost, nektonic ancyloceratine ammonites that fed directly on and within the planktonic ‘clouds’ in the water column (e.g., Mikhailova and Baraboshkin, 2009) (Figure 4).

Figure 4. Successive radiation in time of numbers of planktonic foraminiferal genera and of ancyloceratine ammonite families (data from Rückheim, 2005; Mikhailova and Baraboshkin, 2009).
During the Albian (mid-Cretaceous, 113 - 100 Ma), a group of ammonites that comprised the superfamilies Scaphitoidea and Turrilitoidea, diversified and replaced the Ancyloceratina which previously had been the predominant heteromorphs (Mikhailova and Baraboshkin, 2009). These two groups remained important elements of Middle and Late Cretaceous ammonite assemblages right up to the latest Maastrichtian and into the earliest Paleocene (Landman et al., 2014, 2015).
In recent years, well-preserved buccal masses of scaphitoid and turrilitoid ammonites have been described in detail (e.g., Kruta et al., 2011, 2013). Three-dimensional reconstructions of the buccal apparatus in these ammonites have revealed a similarity that suggests all of them had adopted similar feeding habits. The morphology of the jaws and radula and the presence of possible prey remains inside the buccal mass makes it likely that these ammonites fed on small organisms in the water column, rather than capturing and eating large prey on the ocean floor, as exemplified by living NautilusLinnaeus, 1758 (Kruta et al., 2011). The appearance of this plankton-feeding strategy led to the radiation of the so-called aptychophoran ammonites during the Jurassic and Cretaceous, in conjunction with the radiation of new groups of plankton as discussed herein. Understanding the role of these ammonites in the new Mesozoic food web provides insights into their radiation in the Jurassic, as well as their extinction at the end of the Cretaceous or during the earliest Paleogene (Kruta et al., 2011; Landman et al., 2014, 2015). Phylogenetic studies of the ontogeny and sutural morphology have established that Turrilitoidea and Scaphitoidea together comprise a monophyletic group, with the former derived from Ancyloceratoidea and the latter from the Turrilitoidea (Monks, 1999).
1.4. IRREGULAR ECHINOIDS
Spatangoids (‘heart urchins’) originated during the early Berriasian, c. 145 Ma (Masrour et al., 2004) and experienced diversification during the entire Cretaceous Period. Studies have shown a continuous developmental trend of the group during that interval (e.g., Villier and Eble, 2004). The first representatives (i.e., species of the genus Toxaster) were characteristic of offshore environments. Subsequently, various derived forms colonised warm and shallow waters of shoreface environments, thus augmenting the range of ecological niches occupied by spatangoids. During the Hauterivian-Aptian interval, the genus Heteraster was the commonest heart urchin along the margins of the Tethys Ocean, inhabiting upper offshore and lower shoreface settings that are represented mainly by orbitoline-rich marls and limestones (Masse and Humbert, 1976). Heteraster showed several phases of diversification that can be correlated to the colonisation of new palaeogeographical realms and the development of carbonate platforms. From the Hauterivian to Barremian, this genus diversified in the western Tethys. Its adaptive success resulted from morphological specialisation for more efficient gas exchange and better burrowing ability. This was coeval to the colonisation of new ecological niches in shallow-water platforms along the margins of the Urgonian facies. Heteraster became much more diverse and widespread during the late Barremian and early Aptian (c. 126 - 123 Ma) and remained the commonest and most diverse spatangoid throughout the Aptian (e.g., Yavari et al., 2016). However, new lineages differentiated in shallow waters by the end of the early Aptian, each with adaptive strategies to improve gas exchange - the earliest members of the Macraster/Douvillaster clade (i.e., Douvillaster convexus) and of the family Micrasteridae (Epiaster polygonus). Heteraster, Douvillaster and Macraster remained the most widely distributed forms, but the Albian was marked by a clear differentiation of primitive representatives of the families Micrasteridae, Hemiasteridae and Schizasteridae - i.e., the earliest members of all modern groups of spatangoid echinoids.
Hemiasterids (Hemiaster) became specialised to muddy substrates in offshore settings and to cool, shallow-water carbonates, whereas schizasterids (Mecaster, Periaster and Polydesmaster) adapted to shallow and warmer waters. In the early Cenomanian, the Schizasteridae rapidly diversified and became the major spatangoid group. At the same time, diversity of Douvillaster dropped and the genera Heteraster and Macraster went extinct.
The demise of Macraster, Douvillaster and Heteraster might have been initiated by competition with the diversifying schizasterids that evolved more efficient structures for gas exchange and burrowing in shallow-water environments. Late Cenomanian and younger Cretaceous spatangoid communities were structured as follows: schizasterids and some hemiasterids in upper offshore and shoreface environments, and micrasterids in the mid-shelf zone (Saucède and Villier, 2005).
During this time, irregular echinoids switched to deposit feeding for the first time in the history of the clade. This ecological shift to graze feeding required the development of denser tube feet and, hence, pore pair crowding, necessitating the adoption of plate compounding. In time, the radiation of spatangoid echinoids directly followed the bloom of planktonic foraminifera and ancyloceratine ammonites (Figure 5).
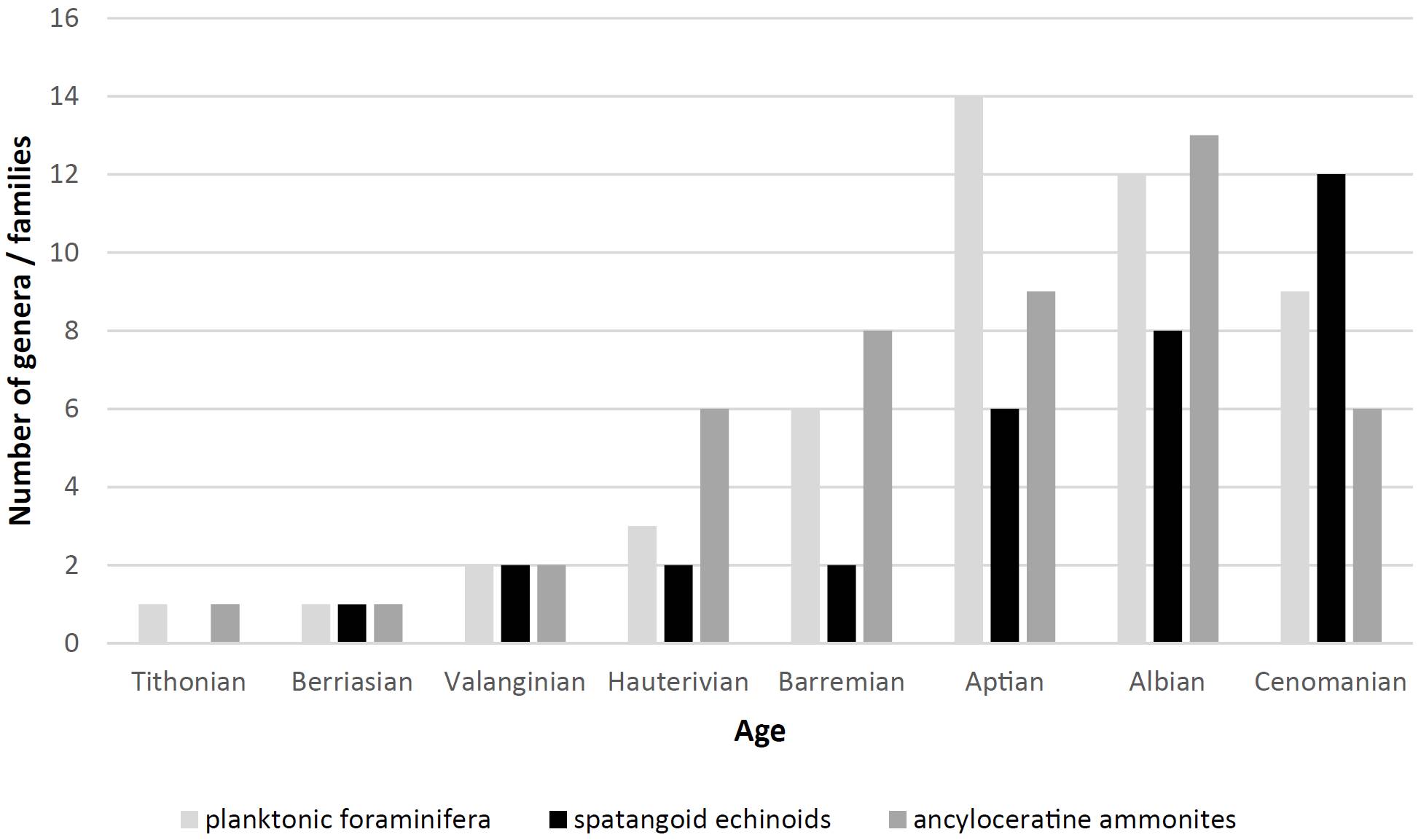
Figure 5. Successive radiation and peaks in time of numbers of planktonic foraminiferal genera, of ancyloceratine ammonite families and of spatangoid echinoid genera (data from Villier et al., 2004; Rückheim, 2005; Mikhailova and Baraboshkin, 2009).
1.5. MEIOFAUNA
The term ‘meiofauna’ refers to a range of metazoan components of the benthos, defined by body size (42 - 1000 μm). These are the most diversified element amongst marine biota - in present-day settings as many as 23 of 33 animal phyla have meiobenthic representatives, either during their entire lifespan or just temporarily (Vincx, 1996). Today, meiofauna constitutes the most abundant benthic group in the marine realm, and is thought to be intimately linked to other faunal components of the benthic system. Amongst meiobenthic protozoans, foraminifera are the commonest and most diverse of shell-bearing micro-organisms in the oceans. The phylum Nematoda, meanwhile, is the most plentiful (often > 50 per cent of total meiofauna, up to > 90 per cent in deep-sea sediments) and diverse metazoan meiofauna taxon (Watzin, 1983; Wägele, 1989; Kalogeropoulou et al., 2010; Balsamo et al., 2012). The rise in abundance and diversity of meiofauna, triggered by the Late Jurassic-Cretaceous plankton bloom, in turn led to a radiation and increased areal distribution amongst a range of benthic groups that had previously been unable to inhabit offshore environments because of a general lack of food. In short, the phytoplankton radiation generated a bloom of zooplankton. The uninterrupted ‘snowfall’ of these two components offered new food sources and niches to occupy, first in shelf settings and later also in more basinal environments.
1.6. RANINOIDIAN CRABS
Prosopids and allied crabs, the most primitive among the brachyurans, flourished in sponge-microbial and coral-reef environments along the northern margin of the Tethys Ocean during the Late Jurassic, making good use of the numerous cryptic habitats within these environments (Jagt et al., 2015). The clade Gymnopleura (that is, Raninoidia), also known as frog crabs, form a group of brachyurans of which extant members are characterised by a fusiform carapace (palaeocorystid-lyreidid-raninid type), a narrow thoracic sternum, a pleon that is partially exposed dorsally and paddle-like limbs, all of which are well suited to their cryptic specialised burying (back-burrowing) lifestyle. Nevertheless, the most basal gymnopleurans were morphologically different, with ornamented carapaces that were wider than long (necrocarcinid-type) and had a broader thoracic sternum and a pleon that fit between the legs, assisted by pleonal locking mechanisms (Luque et al., 2012).
Luque et al. (2012) noted that the Gymnopleura was one of the major brachyuran groups to have adapted to inhabit soft and sandy sediments across a wide bathymetric range, from tropical to low-latitude regions across the globe. The first raninoidian known to date is from the uppermost Jurassic reefal limestones of Štramberk, Czech Republic (pers. obs.). From a basic hexagonal necrocarcinid morphotype (shallow-marine reef inhabitants) arose the fusiform palaeocorystid morphotype (burying within fine-grained, soft sediments).
Extant Raninoides lamarcki still shows a scavenger mode of food acquisition of invertebrates (meiofauna) (Soto and Escobar-Briones, 1995). Rosa and Bemvenuti (2005) showed that meiofauna community structure was negatively affected by the burrowing crab Chasmagnathus granulata (Dana, 1851).
Until recently, the oldest record of a gymnopleuran was that of Paranecrocarcinus hexagonalisVan Straelen, 1936 from the Hauterivian of Migraine, near Auxerre (Yonne, France). During the Aptian, necrocarcinids were already diverse and widely distributed worldwide (e.g., Van Bakel et al., 2012; Schweitzer et al., 2016), whereas the first palaeocorystoid appeared in Colombia (Luque et al., 2012; Luque, 2014). During the Albian both types occurred globally and in large numbers, mostly in shale-rich deposits. Although numerous studies on the earliest gymnopleurans have recognised the order of appearance of the various morphotypes, the successive diversity peaks (Figure 6), and an increase of gymnopleurity over time, a model to explain these changes has not yet been proposed. The phylogenetic relationships of palaeocorystoids have recently been revised by Van Bakel et al. (2012), who concluded that the Palaeocorystidae Lőrenthey in Lőrenthey and Beurlen, 1929 was the most specialised family that was characterised by a back-burrowing mode of life. In view of the fact that the new niche was in relatively deeper-marine environments with predominantly shale-like deposits that lacked crevices and other places for crabs to hide in (such as those in reefal settings), a burying lifestyle was a prerequisite for survival. A major step in developing a shape that was advantageous to such a mode of life involved a series of coherent adaptations (a narrowing of the body, minimisation of the posterior thoracic sternal surface, development of arthrodial cavities in a more axial position and exposure of the pleurites) (Figure 7).

Figure 6. Successive radiation in time of necrocarcinid- and palaeocorystid-type crab genera (numbers) (data from Luque et al., 2012; Van Bakel et al., 2012; Karasawa et al., 2014, Schweitzer et al., 2016; pers. obs.).

Figure 7. From palaeocorystoid (no external gymnopleurity; A, B), via lyreidid (increased gymnopleurity; C) to raninid morphotype (most conspicuous gymnopleurity; D), documenting nichereplacement events over time.
After having been successfully adapted to a burying lifestyle and filling this new marine niche worldwide, the predator-pressure by, for example, fishes and ammonites increased. Driven by this predator-pressure, the mode of hiding/burying had to be improved. Apparently, the increase of gymnopleurity (reduced branchiostegites and exposed pleurae of the cephalothorax) and loss of conspicuous abdominal holding (a double peg on thoracic sternite 5) was most successful in that respect.
1.7. ETYIOID CRABS
Förster (1968, p. 190) postulated that etyioids were derived from a necrocarcinid ancestor. In subsequent studies it has been inferred that etyioid crab taxa were able to swim (e.g., Bishop, 1991; Fraaye, 1996a, b). Bishop (1991) even transferred the genus XanthosiaBell, 1863 to the Portunidae on the basis of carapace features. In view of the morphological similarity of Xanthosia to extant swimming crabs (portunids), an ancestral relationship seems quite possible (compare Wright and Collins, 1972). The most striking morphological features are the following: a thin, dorsally flattened carapace that is wider than long, with multiple spines on subrounded anterolateral margins and with large, widely spaced orbits. There is a general trend in time amongst etyioids from more ornamented carapaces (e.g., Steorrosia (Rathbun, 1935); Albian-Cenomanian) to smoother carapaces (e.g., the late Maastrichtian Cretachlorodius Fraaye, 1996a and early Paleocene FaksecarcinusSchweitzer, Feldmann, Franţescu and Klompmaker, 2012; see Fraaye, 1996b, p. 275) (Figure 8).
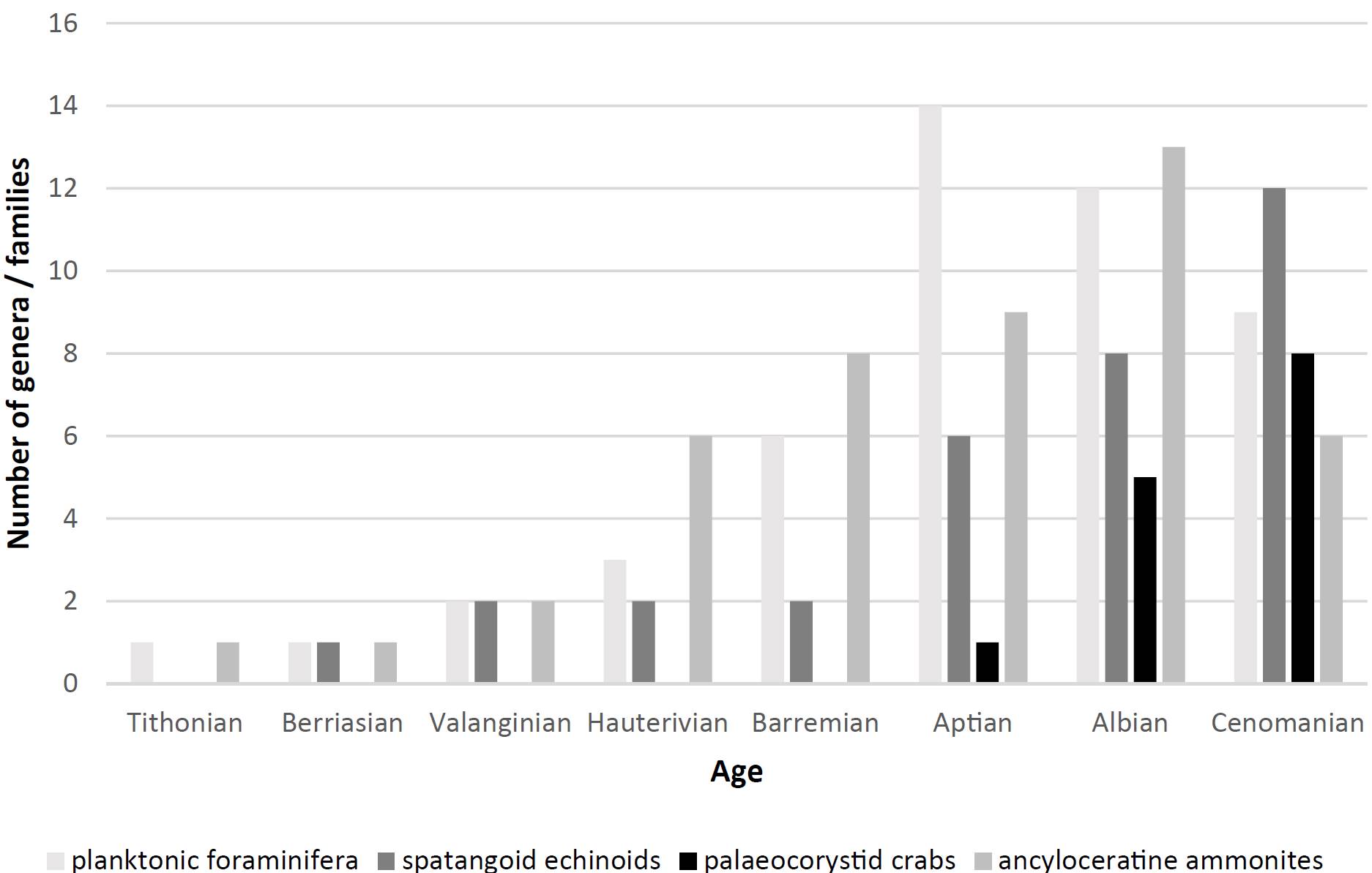
Figure 8. Successive radiation in time of numbers of planktonic foraminiferal genera, of ancyloceratine ammonite families, of spatangoid echinoid genera and of palaeocorystid crab genera (data from Villier et al., 2004; Rückheim, 2005; Mikhailova and Baraboshkin, 2009; Luque et al., 2012, Van Bakel et al., 2012; Karasawa et al., 2014; Franţescu et al., 2016).
It is most likely that the first stage in the swimming mode amongst etyioids was connected to escape reactions in avoidance of predators. In time, the natatory capabilities improved to periods of feeding (capture of prey) and finally to the maintenance of a continued nektonic lifestyle.
Radwański (1996) documented paired punctures that pierced the shells of latest Maastrichtian scaphitid ammonites and interpreted them as having been produced by predatory crabs. Similar traces of damage on scaphitids are not uncommon in the Upper Cretaceous of North America (Takeda et al., 2016). Externally inflicted injuries, indicated by repaired damage on mature shells, account for an average incidence of 10 per cent in Maastrichtian scaphitids from North America, with the exception of the stratigraphically youngest latest Cretaceous levels where scaphitid injuries approach 40 per cent (Landman and Waage, 1986). Fraaye (1996b) subsequently postulated that the predation pressure on decapod crustaceans by, for instance, ammonites, might have led to an ‘evolutionary’ escape of natant etyioids at the end of the Cretaceous. In turn, these etyioids met with a new and abundant food source - scaphitid ammonites. Etyioids were ousted by better-adapted portunids during a faunal replacement event in the Paleogene (Fraaye, 1996b).
Quantitative patterns of decapod biodiversity through time were virtually unknown until the recent study by Klompmaker et al. (2013), who presented genusand species-level diversity curves for different decapod groups in the Mesozoic. They concluded that the diversification of decapod crustaceans coincided with reef expansion and was followed by a severe diversity loss as reefs contracted, suggesting that reefs may have acted as ecosystem engineers over time. The evolution of raninoids and etyioids (Figure 9) are the first two documented examples of radiation in non-reefal settings.

Figure 9. Successive radiation in time of numbers of necrocarcinid, palaeocorystid and etyid crab genera (data from Luque et al., 2012; Schweitzer et al., 2012; Van Bakel et al., 2012; Karasawa et al., 2014; Schweitzer et al., 2016).
2. Conclusions
Major Mesozoic evolutionary and radiation events among marine phytoand zooplanktonic microbiota triggered, both directly and indirectly, the rise of new ecological niches and, concomitantly, evolutionary radiation of various marine benthic and nektonic groups. From the Late Jurassic onwards, the impact on marine invertebrate detritus and plankton feeders was direct. More complex scavenger/detritivore patterns (e.g., brachyuran decapod crustaceans) were intimately linked to the bloom of (part of) their food source (i.e., marine meiofauna). During the Cretaceous Period new innovations and adaptations brought about additional distinctive faunal turnovers in such groups as brachyuran decapod crustaceans, irregular echinoids and heteromorph ammonites. For the first time, a coherent bottom-up model for the Mesozoic marine revolution, from phytoplankton to diverse nektonic and benthic invertebrate groups, is presented here.