1. Introduction
Recent small climatic fluctuations on a 100 - 1000 year time scale can be correlated at least throughout the temperate climatic belt of the Northern Hemisphere, e.g. the Little Ice Age with a decline of the mean annual temperature of < 1° C from 1850 to 1950 (Maisch, 1995). This suggests that major climatic changes on a 100000 year scale (glacial- interglacial cycles) and also on a 10000 year scale (the approximate length of an interglacial), must be of similar ages throughout the temperate climatic belt of the Northern Hemisphere. This concept allows continental pedostratigraphical correlations.
The second necessity for a reliable pedostratigraphy is a time frame of the formations of the loess-paleosol sequences (LPS). This is given first by the knowledge of the position of the Brunhes/ Matuyama (B/M) boundary in the LPS in Luochuan/China, Karamaydan and Chashmanigar/ Tadjikistan and various LPS in Central Europe. Second, the chronostatigraphical position of the last interglacial soil in all LPS is known by thermoluminescence dates (see chapter 3). Third, appropriate methods are necessary for a paleoclimatic interpretation of paleosols. These issues are still controversial and intensively discussed until recently, therefore a methodological chapter is needed.
2. Methodology
For paleoclimatic deductions from LPS it is necessary to understand the processes involved in the genesis of paleosols. This is, however, often difficult because most of the buried paleosols are truncated and more or less recalcified from the overlying loess. Micromorphology allows primary and secondary carbonates to be distinguished; examples are given in Figures 1a and 1c (see also photos 1, 8-12, 17-19 and 23 in Bronger, 1976). This genetic distinction is especially important, for example it allows the distinction between Degraded Chernozems, now referred to as Phaeozems in the World Reference Base (IUSS, 2014) and Chernozems still containing primary carbonates in the lower part of the profile. The development of Phaeozems needs a moister climate (udic soil moisture regime) than Chernozems sensu stricto, which develop in an ustic soil moisture regime (Bronger, 1991, 2003b; for definitions see esp. diagrams 3, 6, 7, 9, 11, 13, and 14 in Soil Survey Staff, 1999).

Figure 1 Micromorphology of paleosols in the loess sections of Tadjikistan and Carpathian Basin; a. Primary calcites, irregularly distributed in the matrix, in typical loess above the PK I in Karamaydan; b. Illuviation argillans as a sign of clay illuviation, which took place with pH-values < 6.2, next to later deposited calcite accumulation of the overlying loess as a sign of a polygenetic soil formation. Bt horizon of PK III in Karamaydan; c. Same as 1b, under +N; d. Fine spongy fabric rich in aggregates and pores in the Ah horizon of the PK III in Karamaydan. Most top soils of the pedocomplexes were removed during the solifluction period of the following cold period; e. Strongly aged illuviation argillans in the PK XV (Matuyama epoch) in Chashmanigar; f. Mid pleistocene rubefied earthy Braunlehm (-Lessivé) F6 in Stari Slankamen / Serbia, upper B(t) horizon; g. Same paleosol as 1f, lower part. Small parts of earthened (aged) illuviation argillans, often granulated and inactive in the fabric.
Micromorphology especially provides a complete view of soil development through different stages, even in polygenetic pedocomplexes (e.g. Kubiena, 1959, 1964; Smolíková, 1967, 1971, 1990; Bronger, 1970, 1976; Catt, 1990; Smolíková et al., 2010) and often reveals the effects of diagenetic processes after burial. Micromorphology also provides an unequivocal indication of clay illuviation. This process occurs in the pH range 4.5 - 6 (in 0.1 M KCl or CaCl2) in soils on plateau sites well above the ground water table. Unless Na+ ions are present, it is associated in temperate climates with a forest vegetation and udic soil moisture regime, but not with a steppe vegetation and ustic soil moisture regime.
This relationship has been found in many Holocene loess soils in East and Central Europe and in the Central Lowlands and Great Plains of the USA (Bronger, 1976: 35-106; Bronger, 1991, 2003b). Even where illuvation argillans1(Brewer, 1964; Bullock et al., 1985) as unequivocal signs of clay illuviation (Figures 1b and 1c) are transformed by changing environmental conditions in buried or relict paleosols, or are aged (Figures 1e and 1g) because of post-pedogenetic or diagenetic processes (which are still poorely understood), they still remain visible as a stable element of soil microfabric. They are even found in the excrements of earthworms (e.g. Zachariae, 1964; photo 3 in Bronger, 1976; photo IA in Catt, 1989) or in “Fließerde” (geliflucted soil) in a buried Mid-Pleistocene Udalf (figures 2A and 2B in Bronger, 1970; photos 1 B-D in Catt, 1989). To summarize, the distinction of primary and secondary calcites and the evidence of clay illuviation enables the recognition of typical loess and the recognition of different genetic soil horizons, such as CB (still with primary calcites), BC, Ah, Bw and Bt horizons (Figure 2).
Figures 3-8 partly summarize our results based on field studies and micromorphological investigations of about 300 thin sections in East and Central Asia, of about 400 thin sections of modern soils and LPS in the Carpathian Basin and several hundred thin sections of LPS in former Czechoslovakia and Lower Austria. Records of magnetic susceptibility have been used for further paleoclimatic interpretation until recently (e.g. Buggleet al., 2008). Markovic et al. (2011) regarded the “record of magnetic susceptibility (MS) variations as one of the most sensitive loess proxies for paleoclimatic change”. However, Maher (1998) already summarized the literature in stating that maxima in magnetic susceptibility values correspond with the paleosols in the Chinese Loess Plateau, in Tadjikistan and in Czechia and minima with the loess layers. In LPS e.g. in Siberia, Alaska and Argentina, however, the complete opposite pattern is found with susceptibility minima corresponding to the most developed pedogenic horizons. Therefore, a paleoclimatic interpretation of magnetic susceptibility records seems at least questionable.

Figure 3 Genesis of paleosols and pedostratigraphic correlation between the sections of Karamaydan, Tadjikistan, Luochuan, China, Stari Slankamen and Nestin, Serbia and Paks, Hungary. PK I - PK III with S1 - S3 (Luochuan) and F2 - F5a (SE Central Europe). For legend see Figure 2.
Another approach towards a paleoclimatic interpretation of LPS is the “rubefication intensity (RI) as proxy of the hematite content” (Buggleet al., 2008). Their conclusion that the F6 soil in Stari Slankamen, Serbia (their S5) because of a high RI value is associated with a “mediterranian/ subtropical climate” (their figure 2, p. 18) - which later was even extended to “mid-latitudinal Eurasia” (Buggle et al., 2014: 18) - is no longer valid (see chapter 3.2). Their misinterpretation may have been also caused by an insufficient consideration of the earlier improved chronopedostratigraphy (figure 11 in Brongeret al., 1998a; Bronger 2003a). Also the hematite increase, determined by 5° K Mössbauer spectra of the 0.2 µm fraction (Bronger et al., 1983) - of Terrae rossae in Slovakia showed that their rubefication is independent of the intensity of weathering (Bronger et al., 1984).
In dated 10-30 ka (Zöller, 2000) “Red Soils” in southern Nepal (at the border to India) surprisingly little pedogenetic clay mineral formation could be identified. The hematites, however, are mostly of pedogenic origin. Therefore, the rubefication is a recent autochtonous process and by itself is not a reliable indicator of strong pedogenic weathering (Bronger et al., 2000). For further paleoclimatic deductions the comparison of the paleosols with the modern soils of the studied region is necessary. Besides the changed lime content (see above) and therefore misleading pH-values in paleosols several other important diagnostic features which can be recognized in modern soils have disappeared or have been changed because of post-pedogenic or diagenetic alteration of the paleosols.

Figure 4 Genesis of paleosols and pedostratigraphic correlation between the sections of Karamaydan, Tadjikistan, Luochuan, China, Stari Slankamen and Nestin, Serbia and Paks, Hungary. PK IV - PK IX (Karamaydan) with S4 - S7 (Luochuan) and F5b - F8 (SE Central Europe). For legend see Figure 2.
For instance, progressive decomposition of organic material with increasing age during the upper Pleistocene in Central Europe (Bronger, 1966, 1974) and even in the Early Holocene in the loess plateau in China (Bronger and Heinkele, 1989; Heinkele, 1990; He Xiubinet al., 2004) prevents the designation of most buried Chernozems (Ustolls) and Phaeozems (Udolls) as Mollisols in the Soil Taxonomy (Soil Survey Staff, 1999). For further paleoclimatic interpretation other soil-chemical methods of investigation applied to unburied (“modern”) soils cannot be used because of post-pedogenic changes in the paleosols.
For instance, the Fed-values are regarded as “useful tools for the characterization of chemical weathering” (Rohdenburg and Meyer, 1966: 90). So the values of the “active” (Feo) and “free” (Fed) iron oxides of four selected Holocene loess soils in the Carpathian Basin (figures 9 and 10) correspond well with the rates of their clay mineral formation. However, these relations are less valid in paleosols with increasing age and sometimes are inverse proportional. As an example the mid-Pleistocene multistory F6 paleosol in Stari Slankamen shows a much higher rate of pedochemical weathering and clay mineral formation than the selected Holocene soils (chapter 3.2; figures 9-11), but the increases of the Feo- and Fed-values are even smaller than in the two Holocene Udalfs (for more details see Bronger, 1974, 1976: 13-22).
On the other hand, geochemistry offers a possibility via weathering indices to quantify mean annual precipitation (MAP) and mean annual temperature (MAT) from paleosol horizons via specifically calibrated empirical transfer equations (e.g. Sheldon and Tabor, 2009; Schatzet al., 2015). So for instance Kühnet al.(2013) calculated the MAP during the formation of the last interglacial Bwt horizon of the LPS in Alsheim/ Mainz Basin, Germany on the basis of equation 30, however, with a standard error (SE) of ±235 mm/yr (Sheldon and Tabor, 2009: 16) being 836 mm (today 613 mm), which seems a bit too high.
Their calculated MAT of the early Würm Humuszones, loesses and sandy loesses in Alsheim using equation 25 from Sheldon and Tabor (2009) with SE of ±0.6° are 9.6° and 8.7°C respectively which are considered as too high by Kühn et al.(2013). Perhaps the unsatisfactory results could be improved by including the Holocene climaphytomorphic soils on loess of the area under investigation in order to get more reliable values of MAP and MAT. In a similar approach Schatz et al.(2015) studied a loess-paleosol sequence near Tokaj (North East Hungary), especially a paleosol, dated 45-27 ka BP. Based on a variety of weathering indices and different transfer functions for major and trace element concentrations (XRF), mass-specific magnetic susceptibility (MS) and stable carbon isotopic (δ13C) data MAT and MAP were calculated. Results converge to a MAT range of 8.5-10°C and a MAP range of 700-900 mm for the paleosol, MS-calculated MAT are 8.4°C and MAP 300-500 mm respectively. These data would have yielded in a distinct weathering of primary minerals and formation of clay minerals. The mineral composition of the silt and clay subfractions of (also partly synsedimentarily formed) loess syrosem “F1” in the loess exposure of Erdut/ Danube, Croatia (Bronger, 1976: 133ff; figure 2 in Bronger, 2003a), dated between 45 ka and 29 ka (Singhviet al., 1989) show, however, (almost) no mineral weathering and clay mineral formation (Bronger, 1976: 185; esp. tab. V in Bronger et al., 1976: 38-39; figures 5A and 5B in Bronger, 2003a). Therefore, the calculated MAT and MAP during the formation of the paleosol near Tokaj with the same age seem much too high.
Therefore, mineralogical investigations are necessary to indicate the nature and intensity of weathering. To enable paleoclimates, the primary and secondary minerals of each fraction, especially that of the clay, must be determined separately. For clay mineralogical investigations it is necessary to separate the clay fraction (< 2 µm) into coarse (2-0.2 µm) and fine clay (< 0.2 µm), to make semi-quantitative estimations possible (for details see Bronger and Heinkele, 1990; Bronger, 2003a).
To evaluate the type and intensity of weathering it is necessary to establish the original petrographic homogeneity of the parent material from which a soil developed. One way of determining this is to use resistant minerals (Barshad, 1967). Quartz is found to be suitable because it is resistant to pedochemical weathering at least in temperate latitudes, and is always abundant in loess. Investigation details of the silt and clay subfractions are given in Brongeret al.(1998a). Figures 9 summarize the mineralogical investigations (see chapter 3.2, 3.6); the figures 9 and 11-19 contain also the particle size distribution.
3. Results and conclusions
3.1. Karamaydan / Tadjikistan - a key sequence of the Brunhes epoch
The pedostratographical correlation of the loess-paleosol sequences in Tadjikistan and Luochuan / China for the Brunhes epoch (figures 3 and 4) is mainly based on two control points. First, the B/M boundary was found in both profiles (Heller and Liu, 1982, 1984; Penkov and Gamov, 1980; Forster and Heller, 1994). Second, the lower soil of the pedocomplex S1 in Luochuan and the lower soil of the PK I in Karamaydan represent the last interglacial or stage 5.5 in the oxygen isotope record. This is supported by TL data of several authors (Nishimuraet al., 1984; Liu, 1985: 213-237; Wintle, 1987; Frechen and Dodonov, 1998).
A comparison of the loess-paleosol sequences of Karamaydan and Luochuan for the Brunhes epoch clearly indicates that the sequence in Karamaydan is the more detailed one. To summarize, the loess-paleosol sequence in Karamaydan should be regarded as a key sequence in the temperate climatic belt of the Northern Hemisphere for reconstructing the climate history of the Brunhes epoch.

Figure 5 Genesis of paleosols and pedostratigraphic correlation between the sections of Karamaydan / Tadjikistan and the composite section of Czechia in the Brunhes chron (for legend see Figure 2). PK I - PK III (Karamaydan) with PK II - PK V (Czechia).
This conclusion is supported by a correlation with the detailed deep-sea oxygen isotope record of Bassinot et al. (1994), tuned to an accurate astronomical time scale. It allows a detailed chronostratigraphical correlation with the loess-paleosol sequence of the key profile of Karamaydan for the Brunhes chron down to the substage level (figures 3 and 4). The more recent oxygen isotope record of Lisieko and Raymo (2005, 2007), which covers the whole Pleistocene time span down to the Pliocene, however, does not give more information regarding the Brunhes epoch.

Figure 6 Genesis of paleosols and pedostratigraphic correlation between the sections of Karamaydan / Tadjikistan and the composite section of Czechia in the Brunhes chron (for legend see Figure 2). PK IV - PK IX (Karamaydan) with PK VI - PK X (Czechia).
The conclusion that the sequence in Karamaydan should be regarded as a key sequence for the Brunhes epoch implies that the loess-paleosol sequences in the Carpathian Basin are less complete than earlier believed (figures 3 and 4). This correlation is again based on the two control points:the B/M boundary, found in Paks and Dunaföldvar below the F8 paleosol and recently below the F8 in Stari Slankamen (Pécsi and Pevzner, 1974; Pécsi and Richter, 1996: 222; Hambach in Markovicet al., 2011).

Figure 7 Loess profiles in the Kashmir Basin and their chronostratigraphic relationships. Arrows indicate location where samples for micromorphological study were collected.
The second control point is the F3 paleosol in the LPS of the Carpatian Basin (figure 2 in Bronger, 2003a, with luminescence dates of A.K. Singhvi) which represents the Riss/Würm interglacial or stage 5.5 in the oxygen isotope record, according to thermoluminescence dates (Wintle and Packman, 1988; Singhvi et al., 1989; Frechenet al., 1997).

Figure 8 Genesis of paleosols and comparison of the section of Luochuan, East Asia with the section of Chashmanigar, Central Asia for the Matuyama epoch; A) Central part of both sections; B) Lower part of both sections. For legend see Figure 2.
3.2. The f6 soil in Stari Slankamen: a welded or multistory paleosol
An example of the incompleteness of the loess-paleosol sequences in the Carpathian Basin is the very strongly developed F6 paleosol in Stari Slankamen, a rubefied Earthy Braunlehm- (Lessivé) in the sense of Kubiena (1956, 1959) and Smolíková (1967, 1971) (figures 1f and 1g) - with a distinct Ck partly Ckm (loess kindl) horizon. Mineralogical investigations of the silt and clay subfractions show much more pedochemical weathering and clay mineral formation than the Holocene soils in the same area (examples in figures 9 and 10): more than 40% of the feldspars and almost 80% of the micas are decomposed (Figure 11); in thin sections only muscovites are found and no biotites remained.

Figure 9 Mineralogical composition of four Holocene loess soils in the Carpathian Basin: two Pachic Haplustolls (1,2) and two Mollic Hapludalfs (3,4). For legend see Figure 12 except phyllosilicates > 2 µm: horizontal lines, vermiculites and mixed-layer clay minerals: crossed lines. Mineralogical composition of each particle size fraction.
This soil was previously believed (Bronger, 1976) to be a soil of the “subtropical soil province” according to Kubiena (1964). It is well preserved in Stari Slankamen but also occurs in Neštin. Later the F6 soil was correlated with the three soils of the S5 pedocomplex in Luochuan (Bronger and Heinkele, 1989) and therefore with the six soils of the two pedocomplexes PK VI and PK V at Karamaydan (Brongeret al., 1998b; see figures 3 and 4). According to the correlation with the OIS curve of Bassinotet al.(1994) the pedocomplexes of PK VI and PK V were formed during ∂18O-substages 15.5, 15.3, 15.1, 13.3, 13.13 and 13.11 in the time span of about 140000 years, although pedogenesis was interrupted several times by loess deposition.

Figure 10 Mineralogical composition of four Holocene loess soils in the Carpathian Basin: two Pachic Haplustolls (1,2) and two Mollic Hapludalfs (3,4). Cumulative curves of the primary minerals > 2 µm and the clay minerals.
This paleosol is therefore an example of a welded (Ruhe and Olson, 1980) or multistory paleosol - as e.g. the paleosol F5 formed during ∂18O-stages 11 and 9 in a time frame of 415 to 310 ka, the F4 and F2 in Stari Slankamen (figures 3 and 4; also chapter 3.4). Mineralogical investigations show only little differences in the type and amount of pedogenic clay mineral formation of selected paleosols in the pedocomplexes VI and V (Figure 15) in comparison with Holocene loess soils (Figure 12), indicating that the climates of the warm phases of PK VI and V were roughly similar to that of the Holocene (cf. chapters 2, 3.6).
3.3. Correlation of the sequence in Karamaydan with the composite section in Czechia
The correlation of the pedocomplexes in Karamaydan with the pedocomplexes of the composite section in Czechia (figures 5 and 6) according to Smolíková (1990) in comparison with the single profiles in the Carpathian Basin is straight forward. The two control points of the correlation are the B/M boundary found below the PK X in Czechia and the lower soil of the PK III in Czechia corresponding with the lower soil of the PK I in Karamaydan, equivalent to stage 5.5 in the OIS. The pedostratigraphical correlation allows an exact time frame of the formation of each pedocomplex in Czechia in the Brunhes chron. So, for instance, the PK IV in Czechia correlated with PK II in Karamaydan was formed in the δ18O stages 7.5 to 7.1 between 240000 and 190000 BC.

Figure 11 Mineralogical and clay mineralogical composition of the strongly rubefied mid-Pleistocene F6 soil in the loess profile of Stari Slankamen, Serbia. For legend see figures 9 and 12.
The PK VIII in Czechia correlated with PK VI in Karamaydan was formed in the δ18 O stages 15.5 to 15.1 between 615000 and 570000 BC.
3.4. Loess-paleosol sequences of the early würm period in Central Asia in comparison with Central Europe
Above the last interglacial F3 paleosol in the Carpathian Basin, there is only one strongly developed paleosol F2 (figures 3 and 4). At Karamaydan and Luochuan, the upper soils of the PK I- and S1- pedocomplex are the only strongly developed paleosols formed in the early Würm period, which is chronostratigraphically equivalent to δ18O substages 5.3-5.1. However, in several loess exposures in the Central Asian Kashmir Valley (India), which today has a xeric soil moisture regime like that of Tadjikistan, three distinct Ah or Aht horizons, each 30-50 cm thick and separated by loess or loess-like sediments, are preserved above the last interglacial Bt horizon (Bronger et al., 1987; see Figure 7). They were formed in the time interval between 80 and 50 ka (Singhvi et al., 1987).

Figure 12 Mineralogical composition of the silt and clay subfractions of two Holocene soils (Typic Haploxerolls or Haplic Phaeozems) in the Tadjik Depression (1700 m above sea level).
These Ah/Aht horizons correspond well with the three Ah horizons of the upper PK III and the PK II -all free of primary calcites- in Dolni Veštonice/Czechia (Bronger, 1976: 146-149). In western parts of Central Europe such as the Netherlands, Belgium and western parts of Germany, three distinct “Humuszonen” above the last interglacial soil are exposed in loess sections (e.g. Semmel, 1989, 1999; Bibus, 1999; Kühnet al., 2013) covering the time interval between 110 and 65 ka (Zöller, 1995; Frechen and Preusser, 1996). The lower and, on a minor scale the middle “Humuszone” in MainzWeisenau display characteristic molluscs of warm phases, among them several dedicated woodland species (Bibuset al., 2002). Therefore, the well-developed F2 soil exposed in many places in the Carpathian Basin (Bronger, 1976; figure 2 in Bronger, 2003a) and its chronostratigraphical equivalents at Karamaydan and Luochuan must be regarded as multistory paleosols.

Figure 13 Mineralogical composition of the silt and clay subfractions of pedocomplexes (PK) I and II in the loess profile of Karamaydan (Tadjk Depression). For legend see Figure 12.
3.5. Loess-paleosol sequences of the Matuyama epoch
For the Matuyama epoch, a paleomagnetic chronostratigraphical time frame of the LPS is provided by Heller and Liu (1982, 1984), but only for the Luochuan section. Figure 8 therefore can only compare the central and lower parts of the Luochuan and Chashmanigar sections. It shows that the central and lower LPS sequence at Chashmanigar, which represents most of the Matuyama epoch probably down to the Olduvai event (Dodonov, 1986, 1991), contains many more pronounced paleosols (about 20) than the equivalent parts at Luochuan. This is especially apparent in the Wusheng loess at Luochuan, which contains only synsedimentarily formed paleosols (‘WS1-WS 3’, see Figure 8).

Figure 14 Mineralogical composition of the silt and clay subfractions of pedocomplexes (PK) III and IV in the loess profile of Kara- maydan (Tadjik Depression). For legend see Figure 12.
It is astonishing that Dodonov and other authors, who worked in Tadjikistan (summarized in Brongeret al., 1995) did not even mention the very distinct Ck (loess kindl) horizons (e.g. Figure 20) in their figures (also Pécsi and Richter, 1996: 175). Most paleosols have lost at least their topsoils during the solifluction period of the following cold period. Sometimes the whole paleosol is eroded, only the Ck horizon, more resistant to soil erosion, remains from the former soil formation (examples in Figure 8B).

Figure 15 Mineralogical composition of the silt and clay subfractions of pedocomplexes (PK) V and VI in the loess profile of Karamaydan (Tadjik Depression). For legend see Figure 12.
In the Carpathian Basin only at Stari Slankamen are three strongly developed though truncated rubefied Braunlehms (in the sense of Kubiena) F9-F11 above Neogene sediments (Bronger, 1976, 2003a), in Lower Austria, however, several more paleosols mostly classified as Braunlehms, rubefied Braunlehms and Rotlehms are exposed, especially in Stranzendorf and Krems-shooting range (Fink and Kukla, 1977; Smolíkováet al., 2010; Sprafke, 2016).
3.6. Additional mineralogy: paleoclimatic deductions
The results of the mineralogical investigations of the three silt and two clay subfractions of selected paleosols in Karamaydan and Chashmanigar / Tadjikistan and for comparison with Holocene loess soils of this area are summarized in figures 12-19. Some points worth emphasizing are the following:
The percentage of quartz by weight in the silt fractions increases only slightly between the C or Ck and the overlying B or Bt horizons in almost all PKs and Ss, thus indicating original petrographic homogeneity. However, the change in the quartz content from 19.5 to 23.5% in S XVI and especially from 21% to 26% in S XII at Chashmanigar (Figure 8) shows a greater petrographic inhomogeneity in these paleosols.
The main sources of pedogenic clay minerals are phyllosilicates in the silt fractions: in most of the paleosols and the Holocene soils there is a considerable decrease in silt-size micas in the B or Bt horizons compared with the Ck horizons. Feldspars are a minor source of the pedogenetically formed clay minerals (figures 12-19).
There is little difference in the type and amount of pedogenic clay mineral formation between the Holocene soils and the buried paleosols (B or Bt horizons) of the Brunhes epoch at Karamaydan and of the Matuyama epoch at Chashmanigar. The amount of pedogenic clay mineral formation in the Quaternary soils is mostly in the range of 10 to 15%. Only in the lower Bt horizons of PK I and IV at Karamaydan it is as large as 20% (figures 13 and 14).
Together with the higher amount of illuviation argillans in these soils it can be concluded that the climates in these interglacials were moister than in the Holocene.
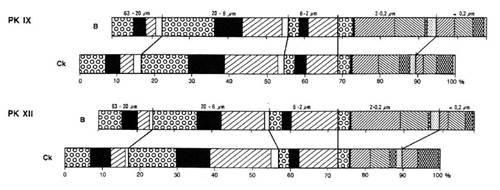
Figure 16 Mineralogical composition of the silt and clay subfractions of pedocomplexes (PK) IX and XII in the loess profile of Karamaydan (Tadjik Depression). For legend see Figure 12.

Figure 17 Mineralogical composition of the silt and clay subfractions of pedocomplex (PK) X and paleosols S XII and S XIV in the loess profile of Chashmanigar (Tadjik Depression). For legend see Figure 12.
This is in agreement with e.g. the area of Southern Moravia / NE Lower Austria: in Dolni Veštonice the last interglacial soil (OIS 5.5) is a Luvisol or Typic Udalf with distinct illuviation argillans (photo 21, 22 in Bronger, 1976), whereas modern loess soils in this area are Calcic Chernozems (Fink, 1964; Hraskoet al., 1973, Bronger, 1976: Stillfried soil; table 1 in Brongeret al., 1976). A limited amount of pedogenic clay mineral formation (10-15%) is also typical for the lower paleosols of the Matuyama epoch in the Chashmanigar section, although S XIX and S XXV, which are more rubefied soils (Figure 8), seem to be more strongly developed than the other paleosols. However, the clay mineralogical composition of the Bt horizons of S XIX and S XXV could only be compared with the (B)Ck horizons immediately beneath.
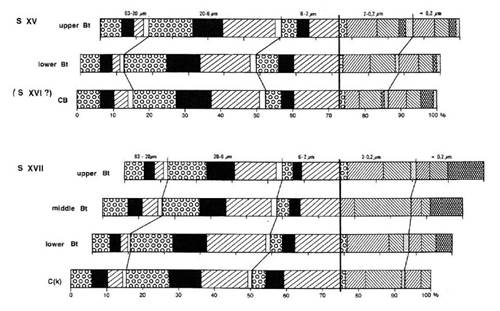
Figure 18 Mineralogical composition of the silt and clay subfractions of paleosols S XV (+ S XVI ?) and S XVII in the loess profile of Chashmanigar (Tadjik Depression). For legend see Figure 12.
These results, briefly summarized here, suggest that the interglacial climates represented by the B or Bt horizons of the buried paleosols of late, middle, and early Pleistocene age were roughly similar to that of the Holocene. Therefore, the partly rubefied Braunlehms, and Rotlehms of old Pleistocene age in the Carpathian Basin and in Lower Austria must be regarded as multistory paleosols.

Figure 19 Mineralogical composition of the silt and clay subfractions of paleosols S XVIII, S XIX and S XXV in the loess profile of Chashmanigar (Tadjik Depression). For legend see Figure 12.
4. Discussion remarks
The LPS of Chashmanigar described by Dodonov (1986, 1991) and Pécsi und Richter, (1996: 175) with paleomagnetic data probably down to the Olduvai event, was covered in 1992 by a big landslide. Therefore, paleomagnetic investigations for a reliable time frame of the detailed LPS of the Matuyama chron in Chashmanigar (Figure 8) would be very helpful.
Most loesses generally are connected with a cold climate especially in the glacial stages. In the central Great Plains of the U.S.A., however, Holocene loess formation (Bignell Loess) is reported (e.g. Olsonet al., 1997; Jacobs and Mason, 2004). It is underlain by the Brady Soil, which divided the late glacial Preoria Loess from Holocene Bignell Loess, which is up to 6 m thick e.g. in the Wauneta exposure in SW Nebraska. The Brady Soil developed between about 12.600 and 9.500 (cal. yr. BP; acc. to Johnson and Willey, 2000), at least during “a minimum of 2000 calendar years” (P. Jacobs, pers. comm.). Recently the age of the Brady Soil - regarded as a cumulative soil which grew upward in aggrading loess - was calculated between 15.040±900 (OSL) and 10.310±90 (14C) (Miaoet al., 2016).
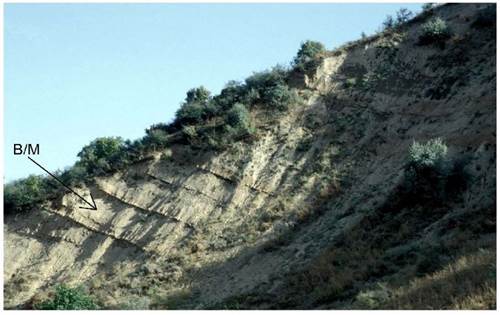
Figure 20 Lower part of Brunhes age loess-paleosol sequence of the section in Chashmanigar. - The autochtonous pedocomplexes XI to V are each underlain by Ck - loess kindl - horizons. The discordance between PK XI and PK X is located in both loess profiles in Chashmanigar and Karamaydan. Between PK X und PK IX the B/M boundary was located (Penkov and Gamov, 1980; Forster and Heller 1994).