Introduction
Since the emergence of the new SARS-CoV-2 (severe acute respiratory syndrome type 2 coronavirus) in Wuhan, China, when it was still virtually unknown, one feature was evident: its high rate of infection among the population. This feature resulted in the spread of the virus, initially in China and Europe, and then in the rest of the world. In Mexico, an approximate mortality of 12% has been reported1. On January 30, 2020, the World Health Organization declared SARS-CoV-2 infection a public emergency, and on March 11, 2020, a global pandemic was declared2. At the time of writing this manuscript, about 7.5 million people have been infected, and about 500,000 have died3.
SARS-CoV-2 generates a clinical picture characterized by fever, dry cough, and fatigue; some patients experience dyspnea, headache, muscle pain, and diarrhea4. Moreover, COVID-19 has been reported to be characterized by an inflammatory response that may contribute to airway damage5. Severe cases may progress to acute respiratory distress syndrome (ARDS) 8-9 days after the onset of symptoms, which can lead to respiratory failure, multiple organ failure, and death6,7.
It has been shown that the SARS-CoV-2 cell receptor is the angiotensin-2 converting enzyme (ACE-2) and that this virus can infect respiratory tract epithelial cells, alveolar cells, endothelial cells, and macrophages in the lungs8-10. The virus expresses on its surface the so-called S-protein (spike), which gives it the appearance of having a crown. This protein binds to ACE-2 and allows viral infection11,12.
Data from patients with SARS-CoV-2 indicate that high levels of neutralizing antibodies are produced after infection; however, the type, level, and timing of the immunity that protects against reinfection remain unknown13. A person who has been infected with SARS-CoV-2 will generate a sufficient immune response to avoid reinfection; however, there are reports of recovered persons relapsing into the disease. These cases are rare and appear to be related to the presence of viral debris rather than infecting virus14.
Previous coronaviruses: SARS-CoV and MERS-CoV
SARS-CoV-2 is part of the Coronaviridae family, of which seven viruses can infect humans: four affect the upper respiratory tract (229E, NL63, OC43, and HKU1), and the other three can damage the lower respiratory tract (SARS-CoV, MERS-CoV (Middle East coronavirus respiratory syndrome), and SARS-CoV-2)12.
The closest relative to SARS-CoV-2 is SARS-CoV, with a genetic similarity of 79%15. SARS-CoV triggered an epidemic in 2002, leaving about 8000 infected people and approximately 800 deaths. Another coronavirus of epidemiological importance that emerged in 2012 was MERS-CoV, which has left approximately 2500 infected people and 858 deaths. The development of animal models was encouraged to study the pathogenesis and replication of SARS-CoV and MERS-CoV viruses. The knowledge generated from the study of these models has led to a better understanding of SARS-CoV-216,17.
MERS-CoV has been shown to infect activated T-cells18 and induce late production of cytokines after infection of myeloid cells such as dendritic cells and macrophages19-21 in vitro, which may contribute to the failure to eliminate the virus and to develop an effective immune response22.
In patients with severe or moderate MERS-CoV infection, virus-specific CD8+ T-cells were detected during the acute phase, while antibodies and CD4+ T-cells appeared later23. In some patients, the T-cell response and antibody generation were detectable 2-3 weeks after diagnosis23,24. In patients with a mild infection, antibody levels tend to be low and transitory compared with severe cases, in which specific antibody levels have been detected for at least 2 years25, while the T-cell response has been detected in all surviving cases, 2 years after infection26.
Furthermore, SARS-CoV infection can trigger a range of humoral and cellular immune responses. Specific antibodies against SARS-CoV (immunoglobulin (Ig)G and IgM) were detectable approximately 2 weeks after infection, reaching a peak at 60 days and remain at high levels for up to 180 days27. Moreover, high neutralizing antibody titers and cytotoxic T-cell responses specific to SARS-CoV were detected in patients recovered from SARS. These findings suggest that immune responses, both humoral and cellular, are crucial for the elimination of SARS-CoV infection28,29. With these results, it has been proposed that both antibody induction and T-cell response are needed to generate an optimal long-term protective response22.
Many therapeutic targets for vaccines have been proposed to stop the spread of these coronaviruses. It is important to remark that most have considered the S protein in its complete structure or its subunits (S1 and S2) because it is the main antigenic component that induces an immune response. Furthermore, it is the protein that the virus uses to enter the host cell17,30. One strategy in vaccine development has been the use of an inactivated virus, which has shown a significant production of neutralizing antibodies. For example, in animal models, the activation of Th2 cells (producers of interleukin (IL)-4, IL-5, and IL-13) can promote the production of antibodies; however, Th2 response resulted in the development of eosinophilia and hypersensitivity in the presence of other pro-inflammatory cytokines16,31-33.
Similarly, vaccines developed with viral DNA produced high neutralizing antibody titers. However, in some cases, Th2 response was exacerbated, probably leading to hypersensitivity. Interestingly, this problem was solved when using combinations of inactivated vaccines and DNA, as this directed the immune response toward a Th1 cell profile, which could reduce the exacerbation of the Th2 phenotype. While DNA vaccines for SARS-CoV have not been promising, it is essential to note that better results have been found for MERS-CoV. In murine models and macaques, neutralizing antibodies were demonstrated, as well as the response of CD4+ and CD8+ T-cells that released interferon-gamma (IFN-γ) and tumor necrosis factor-alpha (TNF-α), providing humoral and cellular immunity16,17,32,33.
Another strategy for the development of coronavirus vaccines has been the use of viral vectors, as these showed to efficiently induce both innate and T- and B-cell-mediated immune responses. However, this type of vaccine produces variable results depending on the preparation and route of administration. When comparing intramuscular and intranasal vaccination, the latter induced a higher neutralizing antibody titer, preventing more effectively the replication of the virus34. The production of antibodies was significantly lower than that obtained with the attenuated virus; however, the vaccine was protective against the virus. It is essential to mention that there is pre-existing immunity to some viral vectors, such as human adenovirus serotype 5, which may decrease the efficiency of CD8+ T-cell response16,34,35.
New alternatives to avoid these complications have been sought, such as chimpanzee adenoviruses, which have entered clinical trials as viral vectors. As an example, a vaccine encoding the MERS-CoV S protein was developed based on a chimpanzee adenoviral vector (ChAdOx1), which induced high levels of neutralizing antibodies and CD8+ T-cell mediated responses in both mouse and human models (Figure 1)35,36.
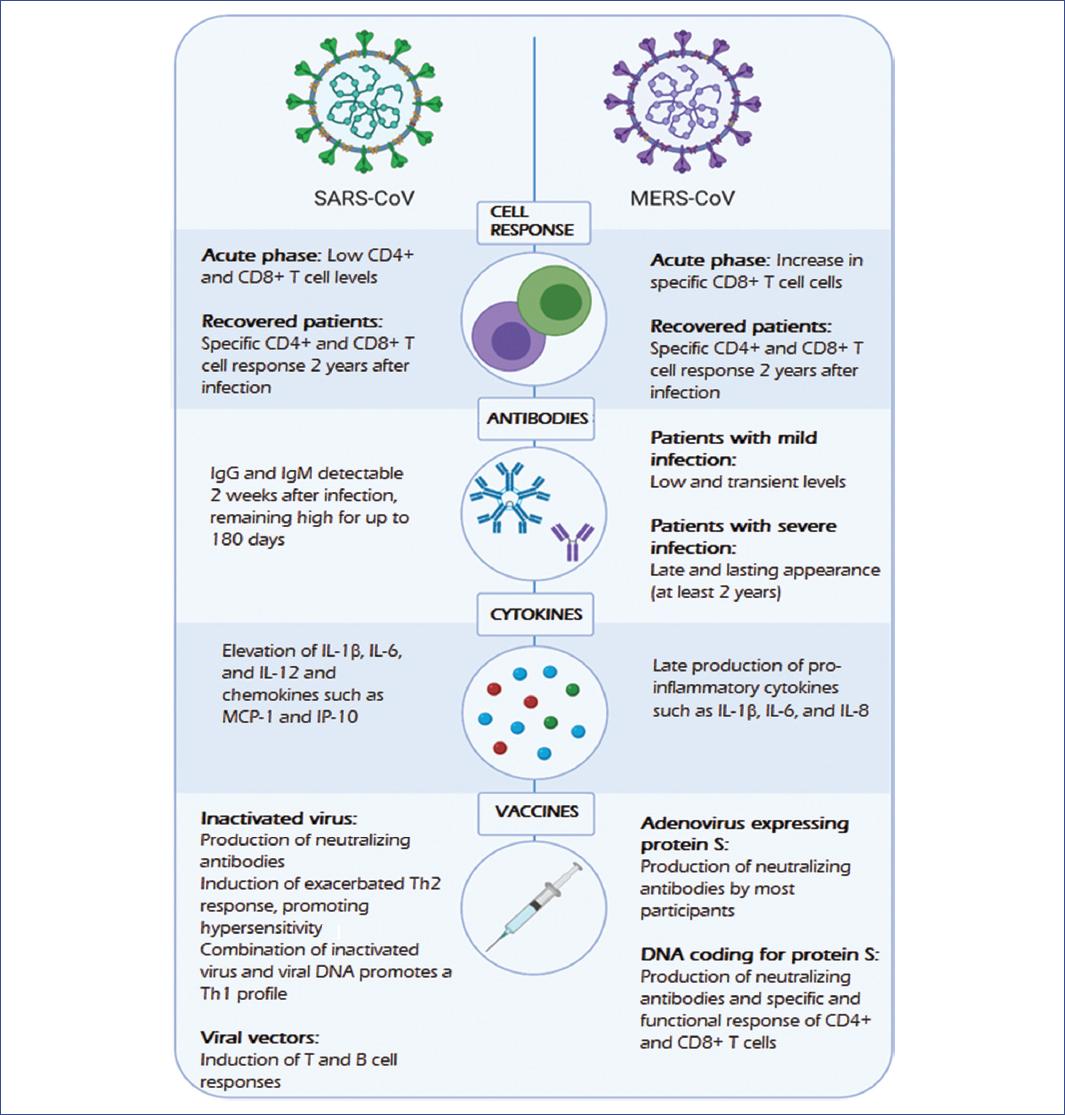
Figure 1 Immune response and vaccines against SARS-CoV and MERS-CoV. SARS-CoV and MERS-CoV are the closest known viruses to SARS-CoV-2; they induce cellular and humoral responses with the production of antibodies and cytokines. Proposed vaccines against these coronaviruses have shown variable results; however, they have served as the basis for the development of SARS-CoV-2 vaccines.MERS-CoV, Middle East respiratory syndrome coronavirus; SARS-CoV, severe acute respiratory syndrome coronavirus; SARS-CoV-2, severe acute respiratory syndrome type 2 coronavirus.
However, only a small number of SARS-CoV or MERS-CoV vaccines made it into Phase I clinical trials, and none of these reached full development, as an abrupt decline in the incidence of these viruses, and the discovery of adverse effects that could exacerbate the disease, cut the funding for vaccine development. Although the opportunity to advance in the development of vaccines against viruses preceding SARS-CoV-2 was lost, the study of the immune response in these coronaviruses shows the importance of inducing both long-lasting neutralizing antibodies and cellular mechanisms (CD4+ Th1 and CD8+ cytotoxic T-cells). Furthermore, the need for platforms to generate protective immunity should be highlighted, as it is not possible to predict when another health emergency might arise from these or other genetically related pathogens.
Present health emergency: SARS-CoV-2 and the development of a vaccine
Regarding the emergence of the new SARS-CoV-2, it has been observed that most patients generate high neutralizing antibody titers 10-14 days after the onset of symptoms; however, some patients had undetectable antibody titers in their blood13. In addition, antibody levels decrease by 11.7% in more than half of the patients after 2 months of recovery37, which suggests that they are not long-lasting and are not the only ones that play an important role in the resolution of the disease. Therefore, a vaccine should not focus solely on generating humoral immunity.
Another significant component of the immune system in the resolution of viral infections is the cellular immune response. Although it has not been studied in-depth for SARS-CoV-2, it could be instrumental in inducing protection against the virus. For example, studies of bronchoalveolar lavage fluid from patients with moderate COVID-19 indicate a high proportion of cells with a transcriptional signature corresponding to plasmacytoid and myeloid dendritic cells, as well as the presence of cell infiltrates with a T-cell signature with tissue-resident phenotype, compared to patients who developed a severe condition38. Therefore, the presence of these cells is crucial for generating an immune response and achieving a better outcome. Consequently, the vaccine that succeeds in obtaining these two components will probably achieves the desired succes. In this context, the use of adjuvants or the characterization of viral components capable of activating and inducing this type of response will be of vital importance.
Furthermore, in ex vivo experiments with COVID-19 convalescent patients, viral S, M (membrane), and N (nucleocapsid) proteins activate CD4+ and CD8+ T-cells. These findings indicate a relationship between the type of clinical picture patients develop and the proportion of CD8+/CD4+ cells specific to viral proteins. When a moderate form develops, the proportion of CD8+ T-cells that produce IL-2, IFN-γ, and TNF-α is higher than that observed in the severe form, with M and NP proteins promoting a more significant response of these cells. In addition to the S protein, M and NP proteins should be evaluated as targets for inducing a T-cell mediated protective response39,40 and consider their use in vaccine development (Fig. 2).

Figure 2 The protective immune response against SARS-CoV-2. Based on preliminary data from studies of COVID-19, the SARS-CoV-2 virus binds to the angiotensin-converting enzyme receptor (ACE-2) through the S (spike) protein, the primary inducer of the immune response. The uptake of this and other antigens such as M (membrane) and NP (nucleocapsid) proteins by dendritic cells (DC), as well as the presence of adjuvants, lead to the antigen presentation, activating CD4+ and TCD8+ effector and memory T-cells. In turn, the infection induces the release of cytokines such as IFN-γ, promoting the recruitment of T-cell infiltrates. CD4+ T-cells that produce IFN-γ, TNF-α, and IL-2 mount an efficient antiviral immune response, which is enhanced by interferon type I produced by plasmacytoid dendritic cells (pDC). Moreover, CD8+ effector T-cells produce cytotoxic molecules such as granzyme (GZM) and perforin, promoting the death of the infected cell. Furthermore, CD8+ TRM (memory-resident) cells may acquire effector functions for the elimination of infection.Regarding the humoral response, there is an initial production of IgM, which are short-term antibodies generated by activated plasma cells, usually through an independent T-cell mechanism. Furthermore, activation of a B-cell by a T-cell dependent mechanism is necessary to produce long-lasting antibodies. In this mechanism, TCR-MHC-II interaction and the release of cytokines (such as IL-4) promote the differentiation to plasma cells and the change of the IgM antibody isotype to IgG. The production of neutralizing antibodies specific for the S-protein inhibits the entry of the virus by preventing interaction with its receptor, which would provide protection. These mechanisms from activation of the humoral to the cellular immune response would lead to the elimination of and protection against SARS-CoV-2 infection. COVID-19, coronavirus disease 2019; IFN-γ, interferon-gamma; Ig, immunoglobulin; IL, interleukin; MHC, major histocompatibility complex; SARS-CoV-2, severe acute respiratory syndrome coronavirus 2; TCR, T-cell receptor; TNF-α, tumor necrosis factor-alpha.
At present, about 14 vaccines are in clinical phase trials (Table 1). Several of these vaccines have shown promising results in animal models, protecting against the virus. Some propose the use of virus-specific T-cells and autologous dendritic cells as an interesting strategy to achieve protective immunity based on cellular immunity; however, evidence demonstrating the effectiveness of these approaches is still lacking.
Table 1 Approved clinical trials for SARS-CoV-2 vaccines
Clinical trial | Clinical phase | Type of vaccine | Results | Developer | Registration |
---|---|---|---|---|---|
Ad5-nCov vaccine | I/II | Recombinant vaccine (adenovirus type 5 vector) | Humoral and T-cell response were induced rapidly in most participants. A peak of IFN-γ, TNF-α, and IL-2 producing CD4+ and CD8+ T-cell responses were observed on day 14 after vaccination, as well as a peak of antibodies specific for the S protein and the RBD, which were able to neutralize the SARS-CoV-2 virus in vitro on day 28 | Beijing Institute of Biotechnology and CanSino Biologics Inc. | NCT04313127 NCT04341389 |
mRNA-1273 vaccine | I/II | Lipid nanoparticle (LNP) dispersion containing an mRNA that encodes for the prefusion spike protein 2019-nCoV | The vaccine was safe and well-tolerated, inducing binding antibody titers similar to or higher than those from patients with the disease. Furthermore, eight patients produced neutralizing antibodies (the rest of the samples have yet to be analyzed). In a mouse challenge model, it provided complete protection against viral replication in the lungs | Moderna Therapeutics | NCT04283461 NCT04405076 |
AZD1222 vaccine (ChAdOx1 nCoV-19) | II/III | Attenuated chimpanzee adenovirus capable of producing protein S of SARS-CoV-2 | Unpublished | University of Oxford AstraZeneca | NCT04324606 NCT04400838 |
INO-4800 vaccine | I | DNA vaccine which encodes for the S protein. | Unpublished | Inovio Pharmaceuticals | NCT04336410 |
PRO-nCOV-1001 y 002 vaccine | I/II | Inactivated SARS-CoV-2 | Unpublished | Sinovac Research & Development | NCT04352608 NCT04383574 |
2019nCoV-101vaccine | I | SARS-CoV-2 Recombinant S Protein Nanoparticle Vaccine | Unpublished | Novavax | NCT04368988 |
BNT-162 vaccine | I/II | Lipid nanoparticle formulated mRNA vaccine encoding SARS-CoV-2 S protein | Unpublished | Pfizer/BioNTech SE | NCT04368728 |
COVID-19 tablet vaccine | I/II | Tablet with SARS-CoV-2 inactivated from the plasma of patients with COVID-19 | Unpublished | Immuitor Inc. | NCT04380532 |
GIMI-IRB-20002 vaccine | I | Lentiviral-based artificial APC vaccine | Unpublished | Shenzhen Geno-Immune Medical Institute | NCT04299724 |
LV-SMENP vaccine | I/II | Lentiviral-based dendritic cell and T-cell vaccine | Unpublished | Shenzhen Geno-Immune Medical Institute | NCT04276896 |
SCB-2019 vaccine | I | Recombinant SARS-CoV-2 trimeric S-protein subunit vaccine | Unpublished | Clover Biopharmaceuticals AUS Pty Ltd | NCT04405908 |
bacTRL-Spike 1 vaccine | I | Bifidobacterium longum CFUs, which contain plasmids that encode for the SARS-CoV-2 S protein | Unpublished | Symvivo Corporation | NCT04334980 |
Inactivated SARS-CoV-2 vaccine | I/II | Inactivated SARS-CoV-2 vaccine | Unpublished | West China Second University Hospital Yunnan Center for Disease Control and Prevention | NCT04412538 |
Autologous dendritic cell vaccine | I/II | Autologous dendritic cell vaccine loaded with SARS-CoV-2 antigens | Unpublished | Aivita Biomedical, Inc. | NCT04386252 |
Gam-COVID-Vac | I/II | Recombinant adenovirus containing the SARS-CoV-2 S protein | Unpublished | Gamaleya Research Institute of Epidemiology and Microbiology, Health Ministry of the Russian Federation | NCT04436471 NCT04437875 |
Information obtained from clinicaltrials.gov. APC, antigen-presenting cells; CFU, colony-forming units; COVID-19, coronavirus disease 2019; DNA, deoxyribonucleic acid; IFN-γ, interferon-gamma; IL-2, interleukin-2; mRNA, messenger ribonucleic acid; RBD, receptor-binding domain; S, spike protein; SARS-CoV-2, severe acute respiratory syndrome coronavirus 2; TNF-α: tumor necrosis factor-alpha.
One vaccine that has caught the attention is AZD1222 (ChAdOx1 nCoV-19), developed by the University of Oxford and AstraZeneca, which uses a platform that was first investigated in the MERS epidemic. This vaccine has shown encouraging results in murine and macaque models, protecting the development of pneumonia by inducing neutralizing antibodies and specific T-cell responses41. Moreover, human trials have begun, and the vaccine will soon move to Phase II/III (Table 1).
Furthermore, two research groups showed encouraging results in humans. Moderna Therapeutics, through its vaccine mRNA-1273, managed to induce neutralizing antibodies in all participants. They found at day 43 (2 weeks after the second dose) that the titers of these antibodies were higher than those observed in patients who have recovered from COVID-19. These results were obtained with the highest dose. Furthermore, in eight volunteers, it was possible to obtain neutralizing antibodies with titers comparable to those observed in a murine model, which were able to prevent viral replication in mice lungs. These results are still preliminary, and the analysis of a larger number of people has yet to be reported42. It has been announced that Phase III of this vaccine could start in July 202043.
Meanwhile, researchers from the Beijing Institute of Biotechnology and CanSino Biologics developed the Ad5-nCov vaccine, which achieved, with its highest dose, to produce binding antibodies in all participants and neutralizing antibodies in 75% of them after 28 days of vaccination. Furthermore, it induced responses in IFN-γ, TNF-α, and IL-2 producing CD4+ and CD8+ T-cells within 14 days of the vaccine application44; however, this vaccine has a disadvantage, since antibodies against the Ad5 vector exist in large part of the population, which could diminish its effectiveness.
A large number of clinical trials are being conducted, and many will follow in the future. However, it is estimated that only about 6% will be completed45. Given the demand that exists, a single vaccine cannot supply the global needs, as a large production and distribution will be required shortly. Therefore, it is likely that each country or region will develop its vaccine. Eventually, after administering each available vaccine, we will obtain results that will show which vaccine is the most efficient.
The full development of an effective vaccine against SARS-CoV-2 will still take several months; for this reason, alternatives have been sought to help in conferring protection with existing vaccines. Controlled trials have recently begun in several countries to assess whether BCG (Bacillus Calmette Guerin) vaccine can reduce the incidence and severity of COVID-19 in health care workers as this vaccine appears to have non-specific effects on the innate immune system, shown by the reduction in the severity of some viral infections. For example, in one experimental infection model, BCG vaccination reduced viremia caused by live attenuated yellow fever virus by 71%46, while in other studies in South Africa, BCG vaccine reduced respiratory tract infections by 73% in adolescents47.
Recently, the mechanisms of the non-specific immune effects produced by BCG were described. It has been observed that vaccination with this bacillus induces transcriptional, metabolic, and epigenetic reprogramming that improves the innate immune response to subsequent infections, a process that has been called immune training48. This response is associated with epigenetic changes, such as methylation and acetylation of histones49 at promoter sites of genes encoding pro-inflammatory cytokines in monocytes, and correlates with improved antiviral responses compared to monocytes from adults who did not receive the vaccine46.
Given the evidence of cross-immunity caused by BCG, its use for protection against COVID-19 could be considered. Some epidemiological studies indicate that countries without a BCG vaccination policy, such as Italy and the United States, have been more affected by the pandemic compared to countries with a standard BCG vaccination policy (Japan and South Korea), where fewer cases of COVID-19 were observed. However, these effects could also be related to health measures and the characteristics of the population of each country. It has been observed that protection lasts about 15 years50, suggesting that the prophylactic effect in the countries whose inhabitants received the vaccine would only occur in the young population. It is also possible that the BCG vaccine may be harmful in some instances due to the increased innate immune response because an exacerbated cytokine response has been associated with complications in patients with COVID-1949. With this evidence, it is clear that there is a lack of controlled clinical studies to verify whether the BCG vaccine could confer protection against COVID-19 and whether this would work as an alternative to reduce the number of severe cases and the number of positive cases. Furthermore, it is necessary to evaluate its impact as a tool in response to SARS-CoV-2 and for future pandemics, while waiting for the development of a specific vaccine that demonstrates safety and efficiency.
In recent days, the WHO has pointed out that the pandemic is not over. Although in some countries the situation is improving, the global picture is worsening. Moreover, a second outbreak of the virus is expected in the coming months. This review shows that there is still much research to be done to have a competent vaccine in the market. Data obtained are still preliminary; however, the first human studies suggest that it is possible to generate antibodies and cellular immunity against SARS-CoV-2. These observations are consistent with animal models that have been tested against the virus, where the immune response can inhibit viral replication and lung damage. We need to have definitive results in human volunteers, study the duration of the immunity developed, and evaluate whether the vulnerable population (such as the elderly) develops the same protective response. Furthermore, it is necessary to assess the cost-benefit ratio of the protection versus the adverse effects presented. It is also important not to be limited to S-protein-based vaccines but include M and NP proteins to induce balanced humoral and T-cell responses. Data presented in this review provide a broader perspective on the development of efficient vaccines for COVID-19, which will significantly benefit society in helping the global population to move out of confinement, to restore social and economic activities, and, above all, to save countless lives.
Addendum
SARS-CoV-2: previous coronaviruses, immune response, and development of vaccines. Update of July 18, 2020
In recent days, many laboratories have published their first Phase studies results. One vaccine that has made recent progress is the Ad5-nCoV developed by CanSino Biologics, which has not published its Phase II results but has managed to be approved by the Chinese government for its use in the military. This use would position it as the first vaccine to be approved51.
Moderna Therapeutics has published its Phase I results of the vaccine mRNA-1273, indicating that all the participants elicited robust neutralizing antibodies titers after the second dose, where their levels were very similar to convalescent patients. Furthermore, the vaccine produced CD4+ T-cell responses specific to the S protein on the group of 100 μg at day 43. These cells produced Th1 cytokines such as TNFα, IL-2, and IFNγ; also, CD8+ T-cell responses were deficient. In groups of 100 and 250 μg, all patients presented moderate adverse effects after the second dose52.
Oxford University has announced the start of Phase II/III. The Brazilian Health Regulatory Agency (ANVISA) approved the inclusion of Brazil in the clinical trials53. Recent reports from The Telegraph affirm that the vaccine is capable of inducing a humoral and cellular immune response. Furthermore, the Phase I results are expected to be published on July 20 in The Lancet54.
Pfizer/BioNTech published preliminary results of its BNT162b1 vaccine, whose 10 μg and 30 μg doses induced neutralizing antibody levels 1.8 and 2.8 times, respectively, higher than the observed in COVID-19 convalescent patients. Local reactions and systemic events were generally mild to moderate and transient. No serious adverse events were reported55.
INOVIO has announced that 94% of Phase I trial participants demonstrated overall immune responses at week 6 after two doses of the INO-4800 vaccine. Through week 8, the INO-4800 regime was deemed safe and well-tolerated with no serious adverse events. Meanwhile, in a preclinical animal study, INO-4800 provided full protection against SARS-CoV-2 replication in the lungs in mice challenged with the virus. INOVIO to begin Phase II/III efficacy study this summer56.
The vaccine PRO-nCOV-1 developed by Sinovac, and an inactivated-virus vaccine developed by Sinopharm launched their Phase III clinical trials in July57. The Russian vaccine developed by Gamaleya Research Institute, a combination of two adenovirus Ad5 and Ad26 and RNA vaccine developed by Imperial College London, started Phase I trial in June57-59.