Introduction
Cancer is a broad group of diseases that can affect any part of the organism. It is promoted by abnormal cell proliferation generated by DNA changes associated with mutations1. The main objective of cancer treatment is to improve the patients’ quality of life, to significantly lengthen life expectancy, or completely cure the disease. Therefore, early diagnosis is fundamental. The orthodox mainstays of cancer treatment are surgery, chemotherapy, and radiotherapy; alternative or complementary therapies include hormone therapy, bone marrow transplantation, non-cytotoxic therapeutic targets, and immunotherapy2.
Chemotherapy is one of the most widely used treatments, whose mechanism of action is to induce cell death by inhibiting the cell cycle3. Radiotherapy is another primary cancer treatment; it is used in at least 60% of patients with solid tumors who receive curative or palliative radiotherapy as part of their treatment4. Radiation causes cell death by inducing stress. In addition, the administered dose may promote senescence or cell death due to the absorption of X-rays or gamma rays or the energy of charged particles that may be ionized or excited5. Another commonly used therapy is hormonal therapy, whose main objective is destroying tumor cells through altering DNA or microtubules6. Finally, immunotherapy promotes the body’s immune response mechanisms to attack and eliminate tumor cells, and it is currently the main area of clinical trial development worldwide7.
Immune response to cancer
The immune system can specifically eliminate tumors and generate long-term memory to prevent cancer recurrence without presenting toxicity to normal tissue. During cancer development, immunoediting occurs, which is composed of three main phases. First, the elimination or immunosurveillance phase, in which tumor cells release danger signals that can mediate their elimination by the immune system before they become clinically apparent. Second, the equilibrium phase, characterized by the dynamic equilibrium between tumor cells and the immune system; these cells may remain quiescent or continue to accumulate changes that modulate the expression of tumor-specific antigens or stress-induced antigens. Third, the escape phase, in which the continuous pressure exerted on tumor cells causes a decrease in immunogenicity and immunosuppressive mechanisms that attenuate the immune response and favor tumor growth and invasion of other organs (metastasis)8.
Innate and adaptive immune response mechanisms are involved in the antitumor immune response9. In the innate response, the main effector cells are the NK (natural killer) cells, whose recognition signals are based on a balance between activation and inhibition signals. The function of NKs is to produce perforins that perforate the membranes of tumor cells and subsequently release granzymes that exert cytotoxic activity. In the early stages of the immune response, neutrophils are among the first cells to infiltrate the inflamed tissue and are the main component of pus. It has been reported that N1 and N2 phenotypes can be encountered during the immune response in cancer. The former plays an antitumor role by phagocytizing tumor cells and secreting proinflammatory cytokines such as IL-6, IL-18, tumor necrosis factor-alpha (TNF-α), immunomodulatory cytokines, and reactive oxygen species (ROS). These molecules are able to eliminate tumor cells and stimulate the recruitment and effector functions of other cells, such as dendritic cells (DCs), B-cells, NK cells, and CD4+ and CD8+ T-cells. The N2 phenotype has been described as pro-tumoral and is characterized by the production of anti-inflammatory cytokines such as IL-1710. During a malignant process, a polarization of N1 neutrophils towards an N2 phenotype favors tumor progression, metastasis, and angiogenesis11.
Furthermore, macrophages play a fundamental role against solid tumors through their proinflammatory and anti-inflammatory functions, which depend on M1 or M2 cell phenotype. For example, M1 macrophages produce proinflammatory cytokines such as IL-1β, IL-6, IL-12, IL-18, IL-23, TNF-α, ROS, and reactive nitrogen species (RNS) and express major histocompatibility complex (MHC) classes I and II and the chemokine CXCL10. This type of macrophage is involved in antitumor and microbicidal responses. In contrast, M2 macrophages are involved in the anti-inflammatory immune response by producing IL-4, IL-5, IL-10, and IL-13, arginase 1, IL-1 receptor antagonist (IL-1Ra), CD163 (scavenger receptor), and CD206 (macrophage mannose receptor type C1), associated with humoral immunity and wound healing. Macrophages may have a dual role in cancer development, as they can either eliminate tumor cells or promote tumorigenesis by facilitating angiogenesis, immunosuppression, invasion, and metastasis through polarization to the M2 phenotype12.
Dendritic cells have numerous functions that depend on cell type, maturation stage, location, or surrounding microenvironment. Their main functions are the induction and polarization of specific immune responses towards Th1 or Th2 and central tolerance by selecting T-cells and peripheral cells. Another function of dendritic cells is the direct interaction and presentation of antigens to CD8+ T-cells or NK and NKT cells through other molecules of the MHC system called CD1 (CD1 a, b, c, d, e)13.
Finally, the adaptive immune system generates a specific response against tumor antigens recognized by the different groups of T lymphocytes (CD4+, CD8+, and Treg). CD4+ or helper T-cells produce cytokines depending on the subgroup to which they belong (Th1, Th2, and Th17). The Th1 subtype cells activate death receptors on cancer cells through the production of interferon-gamma (IFN-γ), while the Th2 subtype is responsible for the activation of the humoral response through the release of the cytokines IL-4, IL-5, IL-10, and IL-13; finally, the Th17 subtype is characterized by the production of IL-17. In contrast, CD8+ (cytotoxic) T cells promote an MHC-l-mediated cytotoxic response by producing perforins, granzymes, IFN-γ, and TNF-α14.
The functions of T lymphocytes can be summarized as recognition, elimination, and memory of antigens. They contribute to the immune response in two main ways: by modulatory signals to promote the release of cytokines or by recognizing and eliminating cells through direct contact. However, the subgroup of regulatory T cells (Treg) is involved in evading the immune response by producing immunosuppressive cytokines such as IL-10, IL-35, and transforming growth factor β (TGF-β), which prevents the proper function of antigen-presenting cells15. Finally, B cells differentiate into antibody-producing plasma cells that can promote tumor destruction by interacting with NK cells, macrophages, and CD4+ and CD8+ T cells16 (Figure 1).

Figure 1 Mechanisms of immune response in cancer. Role of the various cells of the immune system in the development of cancer. CXCL10: C-X-C motif chemokine ligand 10; GM-CSF: granulocyte-macrophage colony-stimulating factor; IFN-y: interferon gamma; IL-1: interleukin-1; IL-12, interleukin-12; iNOS: nitric oxide synthase; M1: macrophages type 1; MIP-1 α: macrophage inflammatory protein-1 alpha; N1: neutrophils type 1; NK: natural killer cells; RNI: reactive nitrogen intermediates; ROI: reactive oxygen intermediates; TNF-α: tumor necrosis factor alpha. Figure made at https://biorender.com.
Immunotherapy in cancer
Immunotherapy, also known as "biological therapy" for its use of strategies that stimulate the body’s natural defenses to fight cancer, is based on the concept that immune cells or antibodies can recognize and destroy transformed cells in the body17. This therapy is usually administered as monotherapy or in combination with chemotherapy or other treatments. The origins of immunotherapy date back to 1891, but it was not until 1980 that the field of immunotherapy finally re-emerged, and the foundations were laid to understand its biological impact. Since then, a large number of immunological studies and clinical trials have been developed18.
There are different types of immunotherapy. One of them is the use of monoclonal antibodies capable of recognizing molecules expressed exclusively in tumor cells (tumor-associated antigens), on which they exert different mechanisms of action that allow their eradication. Among these mechanisms are the distribution of antitumor drugs directly to tumor cells, the blocking of receptors or molecules necessary for the activation of signaling pathways involved in tumor development, and antibody-dependent cellular cytotoxicity19.
Radioimmunotherapy combines a monoclonal antibody linked to a radioactive isotope to deliver radiotherapy directly to tumor cells20. Meanwhile, chimeric antigen receptor T-cell therapy (CAR-T) allows the modification of T-cell receptors to recognize and eliminate tumor cells specifically21. In addition, treatments based on antibodies function as immune checkpoint inhibitors and whose primary purpose is to prevent T cells from attacking healthy cells in the body. Another type of immunotherapy includes the use of cytokines that stimulate immune cells to attack tumor cells22. One of the most conventional immunotherapy treatments is the use of immunomodulators, which is based on the administration of drugs that modulate or intensify the activity of the immune system to fight tumor cells23. Moreover, cancer vaccines introduce inactivated tumor cells, parts of tumor cells, or only antigens that enable T-cell activation or antibody production. Finally, treatments based on oncolytic viruses include introducing laboratory-modified viruses to specifically infect and eliminate tumor cells (Table 1)24-30.
Table 1 Types of immunotherapies
Therapy | Description | Mechanism of action |
---|---|---|
Monoclonal antibodies | Laboratory-generated proteins capable of recognizing molecules expressed exclusively in tumor cells. | They target and ’mark’ tumor cells for destruction through immune system components. They can also act by blocking a ’receptor’ on tumor cells that is important for tumor growth24. |
Radioimmunotherapy | Combined therapy of a monoclonal antibody linked to a radioactive isotope. | The monoclonal antibody binds to tumor cells, and the radioactive substance is released specifically to these cells to induce senescence or apoptosis25. |
Chimeric antigen receptor (CAR) T cells | Treatment in which the patient’s T cells are modified in the laboratory to specifically recognize and attack tumor cells. | CAR T cells target specific antigens on tumor cells by stimulating the activation of the cellular immune response26. |
Cytokines | Treatment with substances produced by immune cells or in the laboratory to promote the elimination of tumor cells. | They induce cell recruitment and promote the death or inhibit the proliferation of tumor cells27. |
Immunomodulators | Drugs that target the initiation of pathways that promote the activation of the immune response. | They modulate or intensify the activity of the immune system to fight tumor cells28. |
Treatment vaccines | Preparation intended to generate active immunity against cancer. | They introduce inactivated tumor cells or antigens that allow the activation of lymphocytes and the production of antibodies29. |
Oncolytic viruses | A type of therapy that uses viruses to deliberately infect tumor cells. | It triggers an immune response against the virus and virus-infected tumor cells30. |
Monoclonal antibodies
Antibodies are glycoproteins secreted by B lymphocytes that belong to the immunoglobulin (Ig) superfamily and are responsible for humoral immunity24. Their main function is to recognize foreign molecules called antigens, on which they exert effector mechanisms. Structurally, they are heterodimers composed of four chains: two heavy chains (CH) and two light chains (CL) connected by disulfide bonds. Functionally, antibodies are divided into Fab (Fragment antigen-binding) regions, involved in antigen recognition, and a crystallizable (Fc) region, which mediates the different effector functions through interaction with cell surface receptors or immune system proteins (Figure 2A)25.

Figure 2 Structure and types of therapeutic antibodies. A: general structure of antibodies. CH: constant region of the heavy chain; CL: constant domain of the light chain, VH: variable domain of the heavy chain; VL: variable domain of the light chain. Fab: antigen recognition fraction, Fc: crystallizable fraction. B: available formats of therapeutic antibodies. Figure made at https://biorender.com.
Monoclonal antibodies (mAbs) are derived from a single cell clone and can recognize a single specific region of the antigen (epitope). The technology required for their development was proposed by Köhler and Milstein in 1975 based on the generation of hybridomas, for which they were awarded the Nobel Prize in Physiology and Medicine in 199826. In the early 1980s, mAbs began to be used as empirical therapy in humans due to their ability to recognize proteins expressed by tumors, which causes an inhibition in the interrelations between receptor and ligand27.
Antibody production methods
Hybridoma generation technology
The procedure for producing a mAb starts with immunizing a mouse with the antigen of interest, followed by the extraction of B lymphocytes from the animal’s spleen, which will be fused with myeloma cells. In this way, hybridomas, cells that combine the antibody-producing capacity of B lymphocytes and the immortality of myelomas, are obtained. Subsequently, the hybridoma is expanded and frozen to finally purify the monoclonal antibody obtained. This hybridoma will be an inexhaustible source of antibodies that will be identical and specific to recognize a single epitope26.
Recombinant DNA techniques
These techniques involve the genetic modification of the original sequences that give rise to conventional mAbs to produce different types of recombinant antibodies containing modifications in the initial structure of the mAb and increase its affinity and decrease its immunogenicity. In general, these techniques consist of extracting genetic material from hybridomas, human B cells, or immunized animals to amplify the sequences encoding the variable regions of the heavy and light chains of the antibodies. The use of eukaryotic vectors and expression systems allows the antibodies generated to correctly carry out the folding and post-translational modifications that allow them to perform their biological function. Among the most commonly used cell lines for the production of recombinant antibodies are hamster (CHO, BHK), human (HEK293, HT-1080, PER.C6, CAP, HKB-11, Huh-7), and mouse (NS0, Sp2/0) cells28. CHO cells are the most widely used cells for producing therapeutic antibodies for cancer; however, one of their major limitations is the high production costs associated with using cell cultures to generate antibodies on a large scale and the low production of antibodies29.
Among the strategies implemented to improve the production of recombinant antibodies is the modification of post-translational changes (glycosylation) in the Fc region30. Strategies focused on maintaining only the antigenic recognition site for subsequent expression in bacteria, yeast, viruses, and plants have also been implemented. Bacterial production systems are the most widely used due to low culture costs, with Escherichia coli being the most used bacterium. The procedure involves amplifying the gene encoding of the desired antibodies, which will later be cloned and used to transform the bacteria. Through inducers, gene expression will be promoted in the bacteria to allow antibody production31. However, despite the advantages offered by this expression system, it usually has some drawbacks, such as the low stability and aggregation of the antibodies produced, as well as the lack of a post-translational modification system, which limits its use when developing antibodies lacking the Fc region32. Furthermore, the production of antibodies in yeast offers advantages associated with low production costs, post-translational modifications similar to those in mammals, and adequate folding in the endoplasmic reticulum. However, one of its main drawbacks is the low success of cell transformation compared to other expression systems and the low affinity of the antibodies produced33.
The most widely used technology for generating antibodies in viruses is the generation of phage display, or phage expression library, which allows to produce repertoires of antibodies against a wide variety of antigens. This technology is based on collecting genes encoding antibodies and their subcloning and insertion into a filamentous phage. Subsequently, single-stranded antibodies (scFv) will be expressed on the surface of the phage, and strategies will be used to select the fragment capable of recognizing the antigen of interest34. Another system for recombinant antibody production is transgenic plants that allow large-scale, low-cost production. Among the most commonly used plants are tobacco, potato, soybean, alfalfa, rice, and corn, which allow the production of complete antibodies, Fab fragments, scFv, bispecific scFv, membrane-anchored scFv, or chimeric antibodies35. The development of antibody-producing transgenic plants is achieved by transfecting them with viral vectors containing genes encoding the antibodies of interest or by using Agrobacterium tumefaciens, a bacterium that infects plants and generates tumors in the stem36. Plants have post-translational modification systems that, when genetically manipulated, allow the production of human-like glycosylated complete antibodies37. Other expression systems in development involve the use of transgenic eggs by inserting genes coding for the antibody heavy and light chains into the chicken ovalbumin gene locus38.
Use of monoclonal antibodies in cancer therapy
mAbs are commonly used in cancer therapy because of their antitumor activity by specifically interacting with tumor antigens39. The first antibody format used for cancer treatment was murine antibodies; however, it was observed that this type of antibody favored the production of human anti-mouse antibodies (HAMA), which reduced the efficacy of the treatment40. Therefore, various alternatives, such as chimerization and humanization, were explored to eradicate these adverse effects associated with treatment. Chimeric antibodies are immunoglobulins containing the variable region of murine origin, while the heavy and light chains are of human origin. Conversely, humanized antibodies retain only the hypervariable regions (CDRˈs) of murine origin, and the rest of the immunoglobulin is of human origin41. Thus, therapeutic monoclonal antibodies can be classified into four major groups according to their origin and composition; murine, chimeric, humanized, and fully human mAbs42 (Figure 2B).
Murine mAbs
The nomenclature used to differentiate murine antibodies from other types of antibodies is the -omab termination. A major disadvantage of using murine mAbs is their shorter half-life when administered in humans due to relatively weak binding to human FcRn. In addition, they have other limitations; for example, they have a low effector function of antibody-dependent cytotoxicity and complement-dependent cytotoxicity, essential functions necessary for tumor destruction43. Over time, a series of modifications have been made through genetic engineering and biotechnology techniques to try to overcome these limitations, allowing total or partial modification of the murine Fc region to improve compatibility with humans or increase its effector function44.
Despite the disadvantages of this type of antibody, some murine antibodies are currently approved in cancer therapy, including ibritumomab tiuxetan (Zevalin) used in the treatment of follicular B-cell non-Hodgkin’s lymphoma. This anti-CD20 antibody is bound to the radioisotope 90-yttrium and has been shown to be effective without adverse effects in patients, even if they have produced HAMA45.
Chimeric mAbs
The generation of chimeric antibodies is achieved through genetic engineering processes, through which it is possible to obtain antibodies of approximately 65% of human regions and 35% of murine origin. The names of mAb of chimeric origin are characterized by the suffix -ximab. Unlike fully murine antibodies, this antibody format usually has a prolonged half-life in humans and shows reduced immunogenicity; however, the tendency of chimeric mAbs to induce HAMA remains considerable46. The first FDA-approved antibody of this type was used in patients with non-Hodgkin’s lymphoma and is recommended as a single agent after relapse; however, options to increase its efficacy and decrease the failure rate are still being explored47.
Humanized mAbs
Humanized mAbs are developed by inserting hypervariable regions (areas showing the six CDR sites of highest variability within the variable region) of murine heavy and light chains into a human antibody. These molecules have about 95% of human regions and are identified by having the suffix -umab. The main objective of humanization techniques is to increase the tolerance of the immune system for the therapeutic antibody while maintaining stability, specificity, and affinity towards the targeted antigen48. The humanization process can be carried out using some of the previously described recombinant DNA techniques to create constructs capable of expressing the antibody in mammalian cell cultures. For this, it is first necessary to isolate the genes that generate the protein, and once cloned, they are grown in a bioreactor so that the antibodies can be obtained in mass. These mAb are less antigenic, better tolerated, and have a longer half-life than other antibody formats49. An example of a successful FDA-approved humanized antibody for cancer treatment is Trastuzumab, an IgG1 antibody against HER2, first approved for therapeutic use in 1998 for breast cancer. Over time, its use has been expanded to other cancers, such as gastric and colorectal cancer50.
Fully human mAbs
With the development of new technologies, producing fully human monoclonal antibodies is now possible. These antibodies are created by inactivating an animal’s endogenous genes for antibody production and replacing them with human genes. These transgenes include the variable region and allow the production of recombinant human antibodies. In this way, high-affinity human monoclonal antibodies can be obtained from transgenic rodents. In addition, large quantities of these antibodies can be obtained from serum of large animals, such as transchromosomic cattle51,52.
Ofatumumab is one of the first antibodies in this format to be approved by the FDA for therapeutic use in cancer. It is an IgG1 class antibody directed to CD20. Although it has proven efficient in treating chronic lymphocytic leukemia, its use in other chronic degenerative diseases, such as multiple sclerosis and rheumatoid arthritis, is still under research. This antibody was developed from transgenic mice immunized with cells transfected with human CD20 and the generation of anti-CD20 antibody-secreting hybridomas53.
Mechanisms of action of therapeutic antibodies
Monoclonal antibodies exert their biological activity through a wide variety of mechanisms, depending on the location of the target antigen (tumor cell, infected cells, cytokines, or growth factors). Understanding these mechanisms of action is vital to revolutionize the methods used for cancer treatment, leading to a more tolerable toxicity profile than conventional treatments54. There are several mechanisms of action by which mAbs can kill tumor cells, including blocking signaling pathways necessary for tumor cell survival, circumventing programmed cell death, evading immune checkpoints, and mechanisms mediated by effector functions associated with the Fc region of antibodies (Table 2)55-63.
Table 2 Therapeutic antibodies
Antibody | Target | Class | Type | Mechanism of action | Development stage |
---|---|---|---|---|---|
Penpulimab | PD-1/tumor | IgG1 | Humanized | It blocks PD-1 to PD- binding, eliminates FcγR binding and antibody-dependent cell-mediated cytotoxicity (ADCC)/antibody-dependent cell phagocytosis (ADCP) completely55,56. | Approved |
Zinberelimab | PD-1/tumor | IgG4 | Humanized | It binds to and inhibits PD-1 and its downstream signaling pathways; this can restore immune function by activating T-cell and T-cell-mediated immune responses against tumor cells57,58. | Approved |
Camrelizumab | PD-1/tumor | IgG4-k | Humanized | PD-1 receptors expressed by T cells bind to their ligands PD-L1 and PD-L2, which can inhibit T cell proliferation and cytokine production59. | Approved |
Tislelizumab | PD-1/tumor | IgG4 | Humanized | The interaction between PD-1 and its ligands, PD-L1 and PD-L2, causes T-cell exhaustion, thereby attenuating the immune response against cancer60. | Approved |
Sintilimab | PD-1/tumor | IgG4 | Humanized | It blocks the interaction of PD-1 with its ligands (PD-L1 and PL-L2) and, consequently, helps to restore the endogenous T-cell antitumor response61. | Approved |
Toripalimab | PD-1/tumor | IgG4 | Humanized | It binds to PD-1 and prevents PD-1 binding to programmed death ligands 1 (PD-L1) and PD-L262. | Approved |
Prolgolimab (Forteca) | PD-1/tumor | IgG1 | Chimeric | It has significant antitumor activity and a manageable safety profile in patients with advanced melanoma63. | Approved |
Receptor inhibition-mediated tumor cell death
This effect is stimulated by receptor agonist activity, such as antibody binding to tumor cell surface receptors to induce cell death64. Another mechanism of direct death occurs when antibodies bind to membrane receptors leading to cell death through the blockade of dimerization or kinase activation, altering transduction signals, which decreases proliferation and promotes apoptosis. Panitumumab is a pure antagonist and induces internalization of the epidermal growth factor receptor (EGFR), which prevents dimerization, autophosphorylation, and signal transduction leading to increased apoptosis and reduced proliferation and angiogenesis (Figure 3A)65.
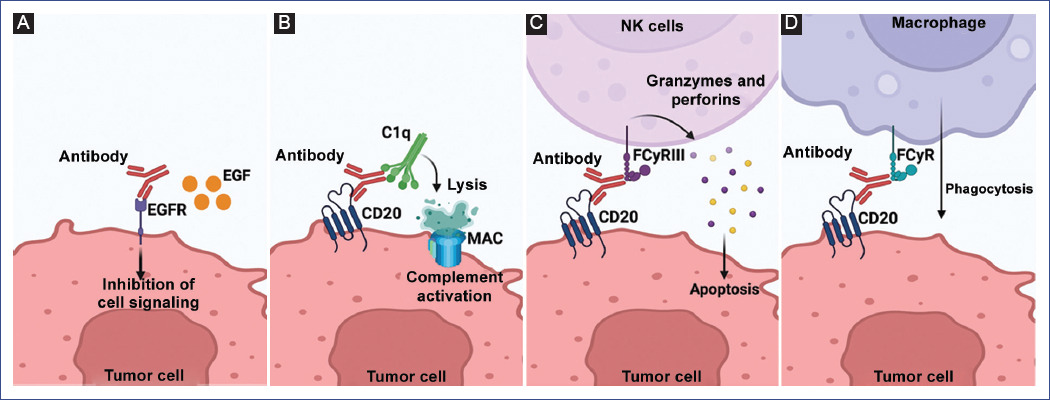
Figure 3 Mechanism of action of therapeutic antibodies. A: direct death. Direct tumor cell death by blockade of receptors and signaling pathways. EGFR: epidermal growth factor receptor; EGF: epidermal growth factor. B: complement-dependent cytotoxicity. MAC - membrane attack complex; CD20 - CD antigen (Cluster of differentiation 20); C1q: C1q complex (complement component 1q). C: antibody-dependent cytotoxicity. FcγRIII: Receptor for IgG. D: antibody-mediated phagocytosis. FcγRIII: Fc gamma receptor (FcγR). Figure made at https://biorender.com.
Immune-mediated tumor cell elimination
The main objective of this mechanism is targeting and eliminating tumor cells. This type of cell death can occur by triggering one of several mechanisms, including complement-dependent cytotoxicity (CDC), antibody-dependent cell cytotoxicity (ADCC), and antibody-dependent phagocytosis (ADP)66.
CDC is a mechanism that involves the activation and recruitment of complement factors, a set of plasma proteins whose purpose is to form a complex on the membrane of target cells and pores that facilitate the exit of intracellular components, leading to death by lysis67. This pathway begins with binding the antibody to its target antigen and the subsequent recruitment of C1q, which will recruit the rest of the complement components to form the membrane attack complex (MAC). One of the FDA-approved therapeutic antibodies that activate CDC is rituximab, which binds to C1q, initiating the typical pathway that ends in cell lysis. This antibody is often used in biotherapy for chronic B-lineage lymphoproliferative syndromes and autoimmune diseases (Figure 3B)68.
ADCC is an effector function derived from antibody binding to a tumor cell and an immune effector (NK) cell. While the variable regions of the antibody (Fab) bind to the antigen present on the tumor cell, the Fc region of the antibody can bind to Fcγ receptors (FcγR) expressed on leukocytes, including FcγRIIIA expressed by NK cells. Activation of NK cells will lead to a signaling cascade to cell death by releasing lytic factors (perforins and granzymes). Rituximab (anti-CD20) induces this effector mechanism by interacting with FcγRIII to kill tumor cells (Figure 3C)69.
Finally, another effector mechanism of therapeutic anticancer antibodies is the activation of macrophages by ADCP, resulting in the encapsulation and digestion of opsonized target cells by macrophages. This function is mediated by binding the antibody’s Fc region to the FcγRI receptor expressed on macrophages, neutrophils, and eosinophils. In general, it has been observed that, like rituximab, several of the antibodies that induce ADCC also activate ADCP because interferon-gamma produced and released by the NK cell induces expression of the FcγRI receptor on polymorphonuclear cells and macrophages, initiating signaling pathways leading to tumor cell phagocytosis (Figure 3D)70.
Use of therapeutic antibodies in childhood cancer
Childhood cancer represent one of the leading causes of death in pediatric patients worldwide. Acute lymphoblastic leukemia (ALL) is the most common childhood cancer, and more than 80% of ALL cases are classified as B-lineage ALL71. Although the survival of this type of cancer has increased in recent decades thanks to advances in diagnosis and treatment, there is still a continuous search for new treatments that help improve the quality of life of pediatric patients and overcome challenges such as relapse72.
Among the antibodies most widely implemented to date and approved by the FDA for treating ALL are blinatumomab and inotuzumab ozogamicin, directed at targets such as CD19 and CD22, respectively. These antibodies are recommended for therapy against relapsed B-cell precursor ALL, although their usefulness in other cancers is also being investigated73. Blinatumomab was the first antibody approved by the FDA to treat ALL. It is a bi-specific antibody comprised of two scFv. This antibody activates T cells and promotes their interaction with malignant B cells because it can recognize the CD3 molecule present on T cells and CD19 expressed on malignant B cells simultaneously, which promotes a synapse and activates the mechanisms of action of T cells against tumor cells. Although this antibody has been approved for treating the main type of childhood cancer, data from these patients are still being analyzed because ALL in pediatric and adult patients is biologically different, with the latter group having a worse prognosis. However, current clinical trials in infants indicate a significant improvement in the survival rate74.
Furthermore, inotuzumab ozogamicin is a humanized antibody conjugated to the cytotoxin calicheamicin. This antibody is directed against CD22 expressed on B-cell blasts. Once the antibody binds to CD22, it is internalized into the malignant cell, and calicheamicin promotes DNA cleavage, leading to death by apoptosis75. This antibody in pediatric patients has been tested in clinical trials, and shown to be effective and safe for administration in infants with relapsed/refractory ALL76,77. Although blinatumomab and inotuzumab ozogamicin were the first two therapeutic antibodies approved by the FDA for treating ALL, other FDA-approved antibodies for treating childhood cancer are currently in different phases of clinical trials. For example, rituximab for treating mature B-cell lymphoma78; epratuzumab combined with standard therapy in relapsed ALL79; anti-GD2 antibodies for treating neuroblastoma80; and antibodies that block tumor necrosis factor-related apoptosis-inducing ligand receptor for the treatment of pediatric sarcomas81.
Limitations of the use of therapeutic antibodies in cancer and perspectives
Despite the great advantages of using monoclonal antibodies as immunotherapy, some disadvantages limit their use. Among them are the elevated cost and low cost-effectiveness that prevent low-income patients from accessing this type of therapy. Costs would have to drop by approximately 57.7-93% to be considered cost-effective82-86. Another disadvantage of complete antibodies is their inability to penetrate solid tumors due to the complexity of the tumor microenvironment and the possible development of immunogenicity reactions in the host. Therefore, a variety of small-sized recombinant antibodies lacking the Fc region have been proposed to facilitate penetration into tumor tissue and decrease the risk of immunogenicity87. However, this modification affects their half-life and elimination, which could lead to elevated costs and excessive accumulation in the kidneys most likely causing long-term kidney damage88.
Antibody-based immunotherapy against cancer represents a promising approach because it overcomes the side effects caused by conventional therapies. As a result, the production and development of therapeutic antibodies have grown exponentially in recent years. It is estimated that by the end of 2023, at least 23 applications will be filed for the commercialization of antibodies currently in late-stage clinical development. Bispecific and drug-conjugated antibodies are of most interest due to their high efficacy in activating the immune system and delivering drugs to specific sites on tumor cells. As of March 2022, more than 100 drug-coupled antibody molecules capable of functioning as "biological missiles"89 were found in various stages of development, and more than 800 antibody therapies in clinical development for cancer as well, representing more than 60% of commercial cancer products90.
In conclusion, antibody-based immunotherapy represents a novel and complementary approach to the use of conventional therapies such as radiotherapy and chemotherapy, which offers patients the possibility of being treated in a personalized manner according to the type of cancer, stage, and antigens expressed by the tumor cells. Since they are a targeted therapy, their main advantage is that they have fewer side effects than conventional therapies. The relevance of mAbs as a therapy lies in their high specificity towards tumor antigens and their different mechanisms of action on this type of cells to eradicate them.
mAbs are not only capable of activating the immune response against tumor cells but are also widely used in conjunction with radiotherapy and chemotherapy to directly deliver the drug to the tumor site. However, the high production costs make them unaffordable for the general population, so it is necessary to optimize lower-cost expression systems that allow large-scale production, such as some of those described in this review. Finally, mAbs have the potential to revolutionize cancer therapy through the development of molecules capable of recognizing a wide diversity of tumor antigens, which will address important unmet medical needs. In turn, this treatment will lead to viewing cancer as a manageable chronic condition rather than a fatal disease.