Introduction
Pharmaceutical products are organic chemical compounds used worldwide to treat disorders and illnesses in humans and animals. Approximately more than 631 different pharmaceutical products have been found at variable concentrations in treatment plants, surface, and groundwater worldwide [1]. Non-steroidal anti-inflammatory drugs (NSAIDs) are the most self-medicated product highly consumed globally to treat chronic diseases, inflammation, and pain, and these are the leading pharmaceutical products detected in water bodies, causing an emerging problem due to their deleterious effects on human health and organisms [2]. NSAIDs are stable and not eliminated by conventional wastewater treatment plants; thus, they can pollute surface drinking water sources and be a risk for humans and biota [3]. It has been estimated that 940 tons of diclofenac were consumed worldwide in 2008, according to the health database Intercontinental Marketing Services (IMS), while in 2014, naproxen ranked fifth among the most detected pharmaceuticals in 50 countries in surface, drinking and groundwater in the world [4].
In Mexico, 10 % of all discarded residues are pharmaceuticals of which naproxen, ibuprofen and diclofenac have been found in the highest concentrations in water [5]; diclofenac and naproxen were detected at 2000-6000 ng/L and 4000-9000 ng/L in surface waters bodies near Mexico City [6]. These pollutants and other NSAIDs are considered as emergent, and countries do not have regulations to control pharmaceuticals in water bodies. It has been reported that pharmaceutical products can have harmful effects on aquatic organisms [7]. Particularly, diclofenac caused 50 % mortality (EC50) of Daphnia magna exposed during 21 days to 2.00 mg/L in water; also, a significant decrease in egg production was observed when this organism was exposed to concentrations lower than 0.50 mg/L [1]. Naproxen can accumulate in the fish bile to a concentrations 1000 times higher than those detected in the water body where the organisms were obtained; also, naproxen caused gastrointestinal and renal effects in fishes [8]. A challenge has arisen for the elimination of NSAIDs because, in the environment, they are present in mixtures of chemicals with different modes of action and unknown side effects in non-target organisms. Conventional water treatment processes, including coagulation/flocculation, sedimentation, and sand filtration have shown low removal efficiencies (<30 %); advanced treatment processes have also been proposed, including those based on membrane filtration, granular activated carbon, and advanced oxidation processes [9].
Constructed wetlands are systems composed by a substrate (sand, gravel, soil, and others) giving support to plants like aquatic macrophytes able to remove different kind of pollutants. These systems have become an easy and inexpensive option to treat water contaminated with metals, pharmaceuticals, organic matter, nutrients, and other organic chemicals, taking advantage of biological and chemical mechanisms that result in processes including adsorption, precipitation, absorption, degradation, where the plants play an important role in the elimination of pollutants [10]. However, the description of the biological and physicochemical interactions that occur in wetlands is complex because the mechanisms of degradation of organic compounds, the interaction of pharmaceuticals with plant roots are still poorly understood, however, some efforts have been made to explain these processes [9,10]. Also, the identification of degradation byproducts needs expensive equipment limiting their study. Nonetheless, the processes involved in the elimination of pharmaceuticals in the constructed wetlands should consider the degradation processes of these substances in the treatment units, since some studies have reported degradation byproducts that could be more toxic than the pollutants originally discharged in the effluents, even at trace concentrations. Thus, in this effort, Domaradzka [11] indicated that 8-chlorocarbazole-1-acetic acid and 1-(6-methoxy-2-naphthyl) ethanol could be the main degradation byproducts of diclofenac and naproxen, respectively. Most of the time, degradation byproducts are present in low concentrations, making difficult to detect and monitor these substances during the removal process.
There is a growing interest in better understanding the removal mechanisms of pharmaceuticals in the treatment processes to improve their removal efficiency. For the constructed wetlands, the laboratory and pilot scale units studies have provided information about the total removal efficiencies of pharmaceuticals from wastewaters, however there is still lacking information about the contribution to the removal of each component of the constructed wetland like the type of substrate, there are limited information on the degradation and fate of these compounds during the treatment and few studies have dealt with the interactions among the substances in the solutions when two or more pharmaceuticals are present, as well as the interactions with the plants used [12].
The main objective of computational chemistry is to predict all kinds of molecular properties of chemical systems using physical chemistry, molecular physics, and quantum physics, it uses a wide variety of theoretical techniques in constant development with which it can predict through mathematical models the interaction between compounds. Currently, computational chemistry studies have focused on the prediction of the behavior of complex molecular systems, the design of pharmaceuticals, and more recently on the simulation of adsorbents for the removal of emerging contaminants [13]. This tool could be used to understand the interactions in wetland systems due to the characteristics mentioned above.
Cellulose is an important component of plant cell walls and is the most abundant biopolymer in the world, contains seemingly simple linear chains of glucose residues, but these chains aggregate to form immensely strong microfibrils that give plants stability. The physical properties of these microfibrils, which keep the plant organized, are responsible for both the elongation of oriented cells during plant growth as well as the force required to maintain an upright growth habit [14], due to these characteristics it would be important and interesting to understand how these molecules interact with pharmaceuticals. The objective of this work was to evaluate the removal efficiency of diclofenac and naproxen in a mixture in a horizontal subsurface flow constructed wetland (HSSF-CW) planted with Typha latifolia, as well as to predict the possible interactions between diclofenac and naproxen in the solution, and with cellulose, that allow us to know and somehow explain and understand about the elimination process of naproxen and diclofenac in the wetland.
Experimental
Installation and operating conditions of the horizontal subsurface flow constructed wetlands (HSSF-CW)
Inside a greenhouse, three HSSF-CW units with a capacity of 135 L (1.5 m long x 0.30 m wide x 0.30 m high; 0.45 m2) were installed. Each unit have one inlet port at a height of 20 cm to feed the solutions (affluent) and one outlet port at a height of 2 cm (effluent) for samples collection. The design follows the criteria proposed for HSSF-CW where the relation length multiplied by width should at least 4:1 due to the removal efficiency of the wetland is a function of the surface area [15]. The HSSF-CW units were packed with gravel to obtain a bed depth of 0.27 m to support the plants and favor the development of roots [15]; the synthetic solution column depth was maintained to 0.24 m during the experiments. Typha latifolia plants of 30 cm tall were planted in the HSSF-CW units and placed at intervals of 20 to 22 cm to allow the root development; the plants were grown for 90 days to achieve a vegetation coverage before experimentation according to that indicated by the U.S. Environmental Protection Agency [15]. During this period, the wetlands received a nutrient solution (1 mM NH4 ++ 1.5 mM K++ 0.125 mM PO4 3-+ 0.25 mM Ca2+); in previous studies, this combination of nutrients favored the plant propagation and growth in greenhouse conditions, and in presence of diclofenac and naproxen, the plants did not show effects of damage such as chlorosis [16,17]. Two HSSF-CW units were planted with Typha latifolia, and the third one was unplanted.
For the diclofenac and naproxen removal experiments, the hydraulic residence time was established to 3 days with an influent flow (affluent) of 10±4 mL/min. During the removal experiments (100 days), one HSSF- CW unit was fed with a synthetic solution with 3 mg/L of diclofenac, 5 mg/L of naproxen, and the nutrients indicated above, a second HSSF-CW was fed only with the nutrient solution (control unit); an unplanted HSSF- CW unit was fed with the solution containing diclofenac (3 mg/L), naproxen (5 mg/L) and nutrients. In this way, the control unit was used to follow the plants growth under non polluted conditions. The non-planted HSSF-CW unit was tested to estimate the contribution of the gravel bed to the total removal efficiency of diclofenac and naproxen. The experiment without plants was follow for 30 days, until the removal percentage remained constant. Inside the greenhouse, during the experimentation, the average room temperature remained around 27 °C.
The concentrations of diclofenac and naproxen were determined at time 0 (initial concentration) in the feeding solution and every three days in the planted and unplanted wetlands. In the horizontal direction of the wetland, from the feeding inlet, samples of the solution were taken at 10 cm (sampling point A) and 130 cm (sampling point B) (Fig. 1). The concentrations of diclofenac and naproxen determined in the samples allowed us to estimate the removal efficiencies of these substances by the HSSF-CW.
Preparation of the synthetic solution and determination of diclofenac and naproxen
Diclofenac and naproxen stock solutions were prepared from compressed tablets. Diclofenac and naproxen tablets (10 of each one) were separately crushed to obtain a power. Then, 100 mg of diclofenac or 550 mg of naproxen were weighed and each one was dissolved in 100 mL of deionized water to obtain stock solutions of 1000 mg/L of diclofenac or 5500 mg/L of naproxen. The synthetic solution was prepared by taking the appropriate volumes of the stock solution to reach 3 mg/L of diclofenac and 5 mg/L of naproxen; the solution was supplemented with nutrients at the concentrations specified above.
The concentrations of diclofenac and naproxen were determined by colorimetric methods specific for each pharmaceutical. The method to determine the diclofenac concentrations is based on its reaction with iron (III) which reduces to iron (II) with potassium ferrocyanide, forming a blue-colored complex that can be measured at 710 nm; the limit of detection (LOD) was 0.12 mg/L [17,18]. The method to determine naproxen is based on the reaction between naproxen and the sulfone phthalein acid dye bromothymol blue (BTB) to form a yellow complex of ion pairs. The resulting complex was measured at 422 nm; the LOD was 1.64 mg/L [19]. Determinations were performed by UV/Vis spectrophotometry (Genesys 10UV). Samples collected from the wetland’s solution (planted and unplanted units) were quantified using the appropriate calibration curve: 0-10 mg/L for diclofenac and 0-10 mg/L for naproxen. Calibration curves were performed with reagent grade diclofenac or naproxen (Merck with purity >98 %).
Three samples of the influent and three samples of the effluent from the HSSF-CW planted with Typha latifolia were selected to be analyzed by GC-MS Spectrometry (GC System Perkin Elmer model Clarus 580 coupled to a Perkin Elmer electron impact ionization mass spectrometer model 560S in laboratories of the University of Guanajuato (Guanajuato, Mexico) with the purpose to detect diclofenac, naproxen, and the presence of degradation metabolites during the treatment. The samples were collected after 40 days after starting the treatment. Measurements were made in SCAN mode with the m/z range set to 40-550. The ion source temperature was set at 200 °C and operated at 2.9 scans per second. GC-EIMS data were examined with the Turbo Mass software (Perkin Elmer, Inc.).
Monitoring parameters of plant growth
The plant growth during the experimentation was followed up through the determination of chlorophyll and the height of the plants [17]. The quantification of chlorophyll was done in samples of Typha leaves, by the method of Arnon [20]; nine plants were selected from the wetland exposed to the pharmaceuticals and nine plants from the control wetland, and leaves samples were taken every 15 days during the experimental time (100 days). The height of the plants was also determined in the elected plants for the chlorophyll determination; measurements were done every 15 days to follow the plant’s growth in both the HSSF-CW planted receiving the solution with the pharmaceuticals and in the control.
Molecular modeling design and optimization
To understand the nature of the naproxen and diclofenac removal in the wetland, the intermolecular interaction between diclofenac, naproxen, and cellulose was computationally evaluated. All calculations were performed at the semi-empirical level of theory using the PM6 method [21] implemented in Gaussian09W [22]. The semiempirical method PM6 was used because it provides accurate predictions of energies and structures, in comparison with its predecessor, at a low computational cost [23].
The molecular modeling methodology consists of the molecule design followed by geometry optimization and vibration frequencies calculation [23]. Geometry optimization is a procedure that attempts to find the lowest energy spatial configuration of the molecule. For a frequency calculation, positive values indicate that we found a stable configuration rather than a transition state characterized by one imaginary frequency [24].
For this work, a fragment of cellulose was modeled by three β-glucose units, which is the right size to support one diclofenac or naproxen molecule. To obtain this fragment, a monolayer consisting of two cellulose (Iβ) chains was built using the cellulose-builder software [25], then we took only one chain from this monolayer and finally we reduced the chain to three β-glucose units (Figures 1 and 2, Supplementary Material). The cellulose fragment and the neutral molecules of diclofenac and naproxen were optimized without constraints, and their frequencies were calculated. In addition, the diclofenac anion and naproxen anion were optimized because it has been reported that naproxen [26] and diclofenac [27] can be found in the environment in its anionic form.
Diclofenac, Naproxen and Cellulose proposed interactions
To understand the interaction between cellulose and pharmaceuticals, one molecule of diclofenac or naproxen was superimposed on the cellulose, these systems were optimized, and their frequencies were calculated. The interactions of diclofenac with cellulose and naproxen with cellulose were analyzed through the energies obtained [28]. Since pharmaceuticals could interact with each other in wetlands, the interaction between diclofenac and naproxen molecules was also evaluated.
Results and disussion
Removal efficiencies of diclofenac and naproxen as a mixture by the HSSF-CW
Initially, the operating conditions of the wetland were established during the growth stage of T. latifolia to get the vegetation coverage in the wetland units as explained in Methodology (influent flow 10±4 mL/min; HRT 3 days).
At the beginning of the experimentation, the concentrations of the feeding solution were measured, which were 2.58±0.33 mg/L of diclofenac and 5.05±0.20 mg/L of naproxen. Figure 2 shows the total removal efficiency calculated from the difference between the influent and effluent concentrations of diclofenac and naproxen in the wetland throughout the experimental time. In the influent, the diclofenac concentration remained between 2.5 mg/L and 3.2 mg/L, while in the effluent, the concentration was 0.50±0.08 mg/L throughout the experimentation. In the influent, the concentration of naproxen varied from 5 to 6 mg/L, while in the effluent remained below 2.70±0.85 mg/L. The results showed that the wetland system planted with T. latifolia removed 82.0 % of diclofenac and 74.5 % of naproxen from the mixture. These results contrast with our previous work, where the removal efficiencies were 98.4 % for diclofenac and 97.7 % for naproxen in HSSF constructed wetlands planted with Typha latifolia and packed with gravel as support materials for plants; plants in the wetlands were exposed to single solutions of diclofenac (3 mg/L) or naproxen (5 mg/L) and the HRT was 5 days [17]. According to the results, diclofenac and naproxen were detected in the effluent, indicating that these substances were not completely removed as shown in Fig. 2. The HRT to which wetlands units were operated in this work was shorter than our previous work and possibly this caused that few metabolites were detected suggesting that diclofenac and naproxen were not degraded during the experimental time. These data were verified with the results of GC/MS, where diclofenac, naproxen and two degradation products have been detected so far in the effluent samples, however, the degradation products were not clearly identified.
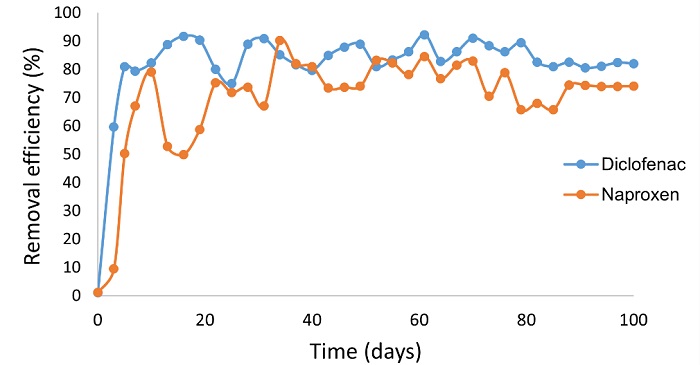
Fig. 2 Total removal efficiencies for diclofenac and naproxen determined in the experimental time from the HSSF-CW planted with T. latifolia.
The results obtained about naproxen removal efficiency are similar to those reported by Lancheros et al. [29] in a HSSF-CW wetland with Cyperus ligularis with a TRH of 2.5 days to treat a mixture of ibuprofen (50 µg/L) and naproxen (50 µg/L); the total removal efficiencies determined for ibuprofen and naproxen were 92 % and 83 %, respectively. Lancheros et al. [29] proposed that the removal efficiencies were due to three factors attributed to the plants, in strict importance order, microbial degradation, adsorption, and plant uptake. However, the role of each factor is still poorly understood, and the research faces the challenges of having access to methodologies with high detection limits to quantify the degradation byproducts of organic pollutants like the pharmaceuticals during the treatment processes.
The diclofenac and naproxen concentrations used in this work were in the order of mg/L, higher than the concentrations of diclofenac and naproxen reported in treated municipal wastewaters which are in the order of µg/L [6]. Therefore, the wetland planted with Typha latifolia has a great capacity to eliminate these compounds even in higher concentrations than those tested by Lancheros et al. [29] with C. ligularis. On the other hand, the removal efficiency for diclofenac is like that reported for a HSSF constructed wetland planted with Glyceria maxima, which removed 53-66 % of diclofenac and 74-87 % of ibuprofen from an aqueous mixture of ibuprofen (500 µg/L) and diclofenac (100 µg/L) [30].
Hijosa et al. [31] studied the behavior of a mixture of pharmaceutical and personal care products (ibuprofen, ketoprofen, naproxen, diclofenac, salicylic acid, caffeine, carbamazepine, methyl dihydrojasmonate, galaxolide and tonalide) in five wetland units of which three were planted with Typha angustifolia and two with Phragmites australis. By analyzing their results and comparing the wetland cells, Hijosa et al. [31] identified that the root of Typha angustifolia had a greater capacity to absorb pharmaceuticals than Phragmites australis. Although the correlation of greater absorption with greater biomass is not established, Hijosa et al. [31] indicated that Typha angustifolia had greater biomass than Phragmites australis attributing to this the differences observed in the removal of the substances studied. In their wetlands, plants of Typha angustifolia were exposed to concentrations of 0.41 mg/L for diclofenac and 1.74 mg/L for naproxen, and the elimination efficiencies were higher than 60 % for both substances which indicates the ability of this plant to eliminate pharmaceutical products.
Typha latifolia has shown to be tolerant to diclofenac and naproxen even at high concentrations such as 5 mg/L diclofenac and 10 mg/L naproxen; tests were done in bath systems without a plant substrate [17]. In this study, the plants height and the total chlorophyll were determined for nine plants from each wetland unit (fed with diclofenac and naproxen, and in the control wetland). The concentrations of chlorophyll and the height of the plants in the wetland that received the mixture of diclofenac and naproxen were not significantly different from the values for these same parameters in the plants of the wetland that only received a solution with nutrients; the results being compared were those determined at the end of the experimentation. At the beginning of the experimentation, the average height of the selected plants in the control wetland was 170±10 cm and at the end of the experiment, the plants were 230±14 cm height. The height of the plants in the wetland with diclofenac and naproxen was 171±12 cm at the beginning of the experiment and 228±2 cm at the end. According to these results, the plants growth in the control unit was similar to that the wetland with diclofenac and naproxen; data obtained from the plants in both constructed wetlands were not statistically different (P<0.05), which indicates that the plants were not affected by exposure to the pharmaceuticals. At the beginning of the experiment, the total chlorophyll content was 2.08±0.28 mg/g (n=9 plants) in the control wetland and 1.41±0.18 mg/g (n=9 plants) in the wetland with the pharmaceuticals, while at the end of the experiment, the total chlorophyll content was 2.51±0.07 mg/g and 3.0±0.12 mg/g, respectively. Thus, the mixture of diclofenac and naproxen did not affect the development of the plants compared to the control, rather it doubled the total chlorophyll, which reflects that the pharmaceuticals have not affected the growth of the plant. During this project, the removal efficiencies for diclofenac and naproxen were determined in an unplanted wetland unit to estimate the contribution of the support material (gravel) for the plants to the total removal efficiencies. The experimentation was carried out for 30 days. Influent concentrations were between 2.4 and 2.9 mg/L for diclofenac, and between 4.5 and 5.4 mg/L for naproxen; the HRT was 3 days, and the flow was maintained to 10±2 mL/min. Diclofenac concentrations in the effluent remained constant from day 16 onwards, where the concentration detected was 1.68±0.31 mg/L; the same was observed for naproxen whose concentration was 3.32±0.02 mg/L. Thus, after 16 days of experimentation, the gravel removal contributions were 20.4 % for diclofenac and 30.8 % for naproxen; at the same period of experimentation, the total removal of diclofenac and naproxen in the planted wetland were 88.0 % and 76.0 %, respectively.
Several processes could be occurring in the HSSF-CW and contributing to the removal of diclofenac and naproxen. One of this process could be the adsorption by the substrate for the plants, like gravel in this work. Zapata et al. [17] estimated that T. latifolia removed more than 90% of diclofenac or naproxen in batch systems with single solutions of the pharmaceuticals at the concentrations tested in this work. According to the results of Zapata et al. [17], T. latifolia can remove diclofenac and naproxen. In this work, by considering the substrate contribution, the removal attributed to the plants is estimated at 67.6 % for diclofenac and 45.2 % for naproxen however no distinction was made about the processes occurring at the root level like adsorption, absorption and those attributed to the microorganisms developed in the root that could contribute to the assimilation of these substances by plants, as proposed by Zapata et al. [17]. Avila et al. [32] conducted a test in pilot scale vertical flow (VF) constructed wetlands with sand or gravel and planted with Phragmites australis exposed to eight pharmaceuticals and found that the constructed wetlands with sand removed higher concentrations than those with gravel except for diclofenac for which the VF constructed wetland based on sand removed 85 ± 17% and that with gravel 74 ± 15%, thus, the substrate was important in the removal efficiency of the pharmaceuticals. Avila et al. [32] indicated that a higher removal of diclofenac was observed in wetlands with less oxidative conditions; this aspect was not evaluated in this work.
Ilyas et al. [33] utilized horizontal flow constructed wetlands (HF-CW) and indicated that the removal efficiency of diclofenac is low due to the limitation of photodegradation and aerobic biodegradation. Spite diclofenac has a high bioaccumulation potential (log KOW 4.51) and be accumulated by the plants, also, the environmental conditions in HSSF-CW play a considerable role in its elimination mechanisms since in constructed wetlands, the aerobic biodegradation can promote the elimination of the organic substances. It has also been suggested that removal efficiency can be improved under anaerobic conditions occurring in HSSF wetlands.
Since the role of microorganisms associated with drug-exposed plant roots is not ruled out, our previous results [17] suggested that microorganisms isolated from the root of Typha latifolia can tolerate concentrations of up to 2500 mg/L diclofenac and naproxen individually. These bacterial isolates are also shown to be plant growth promoters, showing the ability to produce indoleacetic acid, siderophores, and solubilize phosphates, which is observed in the development of plants that do not show phytotoxic effects when exposed to the pharmaceuticals.
Chemical interactions among diclofenac, naproxen, and cellulose
Optimized molecules are shown in the supplementary material.
a) Molecular interactions of diclofenac and naproxen with cellulose
Optimized interactions of cellulose with naproxen neutral/anionic or diclofenac neutral/anionic are shown in Fig. 3.
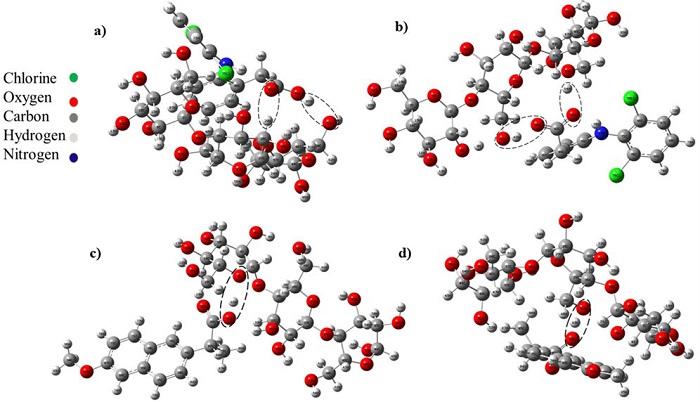
Fig. 3 Interactions of diclofenac and naproxen with cellulose: (a) Interaction between neutral diclofenac and cellulose; (b) anionic diclofenac with cellulose; (c) neutral naproxen interaction with cellulose, and (d) interaction between anionic naproxen with cellulose.
For all systems, the observed interaction between cellulose and diclofenac or naproxen occurred by means of hydrogen bonds. The interatomic distance among the atoms that form the hydrogen bonds is also shown in Table 1. For a hydrogen bond X----H-B, where X is the hydrogen bond acceptor and B is the hydrogen bond donor, the X----H interatomic distance is an indicator of the hydrogen bond strength. According to Buemi and Zuccarello [32], the hydrogen bond interactions could be weak (greater than 2.2 Å), moderate (1.5-2.2 Å), and strong (1.2-1.5 Å). In the neutral diclofenac-cellulose system (Fig. 3a) two hydrogen bonds of moderate strength are observed, H95-O63 and O93-H29, the first is formed between hydrogen from the diclofenac COOH group and oxygen from cellulose with an interatomic distance of 1.7175 Å; the second is formed between oxygen from the diclofenac COOH group and hydrogen from cellulose with an interatomic distance of 2.1875 Å. For the neutral naproxen-cellulose system (Fig. 3c) the hydrogen from the naproxen COOH group interacts with oxygen from cellulose forming a hydrogen bond of moderate strength, with an interatomic distance H92- O5 of 1.73489 Å. In the anionic diclofenac-cellulose system (Fig.3b) two hydrogen bonds of moderate strength are observed, O93-H64 and O94-H43, both are formed from the interaction between the oxygens in the diclofenac COO- group whit cellulose. For the anionic naproxen-cellulose system (Fig. 3d) one strong hydrogen bond are formed from the interaction between the oxygen from naproxen COO- group and hydrogen from cellulose, with an H43-O90 interatomic distance of 1.3292 Å.
Table 1 Interaction energies and interatomic distances for diclofenac or naproxen with cellulose.
Interaction energy ΔE(kcal/mol) | Interatomic distance(Å) | ||
Neutral diclofenac-cellulose | -17.9256419 | H95-O63 O93-H29 | 1.7175 2.1875 |
Anionic diclofenac-cellulose | -34.07496308 | O93-H64 O94-H43 | 1.67928 1.64767 |
Neutral naproxen-cellulose | -8.478623125 | O5-H92 | 1.73489 |
Anionic naproxen-cellulose | -34.87168727 | H43-O90 | 1.3292 |
Neutral diclofenac-neutral naproxen | -612.8773989 | H96-O65 | 1.782 |
Anionic diclofenac-anionic naproxen | -539.087965 | O29-C36 | 1.802 |
The interaction energy (∆E) for all the systems, shown in Table 1, was calculated as [28]:
Where, Ecellulose-drug systems is the energy of the optimized cellulose - drug interaction, and Ecellulose + Edrug is the energy of the optimized cellulose fragment plus the energy of the optimized drug molecule, the drug refers to diclofenac or naproxen. The ∆E values suggest that the interaction between anionic drugs and cellulose is stronger than the interaction between neutral drugs and cellulose; also, we noted that there is not significative difference among the interaction energy of the anionic diclofenac-cellulose and the anionic naproxen-cellulose systems. Then we could explain the slightly higher removal of diclofenac (82 %) vs naproxen (74.5 %) because the diclofenac forms more hydrogens bonds than naproxen which could favor the adsorption process onto cellulose.
b) Interactions between diclofenac and naproxen
The interactions between neutral diclofenac and naproxen (a), and in the anionic forms (b) are shown in Fig. 4. In both cases, naproxen bonds to diclofenac; furthermore, hydrogen bonds are observed between the carboxylic groups of neutral diclofenac and naproxen (a). The interaction energies, Table 1, indicate that the interaction between neutral pharmaceuticals is stronger than the interaction between anionic pharmaceuticals.
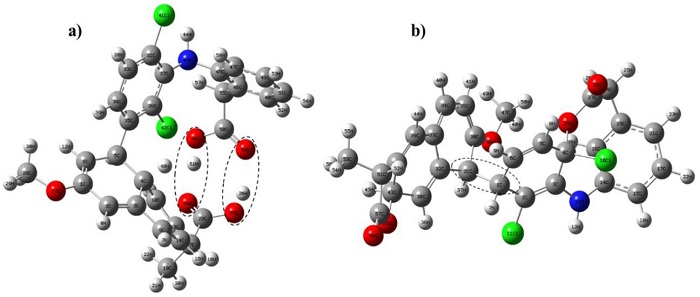
Fig. 4 Interactions between diclofenac and naproxen: (a) Interaction of the non-ionic molecules of diclofenac and naproxen; (b) Interaction between the anionic molecules of diclofenac and naproxen.
This result suggests that when naproxen and diclofenac are mixed in the wetland, they form a more complex molecule that would hinder the adsorption/absorption processes carried out by plants. This could explain the lower removal efficiencies in the constructed wetland with T. latifolia exposed to a mixture of diclofenac and naproxen (this work) compared to those previously determined using individual solutions of diclofenac or naproxen [17].
Conclusions
In this work, a synthetic solution with diclofenac (3 mg/L) and naproxen (5 mg/L) was treated using a constructed wetland of subsurface flow type planted with Typha latifolia; the input flow was maintained at 10±4 mL/min, the HRT was 3 days, and the experiment was followed up for 100 days. The HSSF-CW had a total removal efficiency of 82 % for diclofenac and 74.5 % for naproxen. Results obtained from an unplanted HSSF- CW at 30 days, the gravel bed contributed with 20.4 % for diclofenac and 30.8 % for naproxen while the plants contributed with 67.6 % for diclofenac and 46.0 % for naproxen calculated at the same period. According to data obtained of the plant’s height and the total chlorophyll content during the experiment, the plants were not affected by the pharmaceuticals. Computational modeling of the chemical interactions between diclofenac, naproxen, and cellulose at the semiempirical PM6 level of theory indicated that the intermolecular interactions are mediated by hydrogen bonds; furthermore, the strongest interaction seemed to occur between cellulose and diclofenac. This could explain the higher removal efficiency of diclofenac compared with naproxen.
Constructed wetlands planted with Typha latifolia are efficient in the removal of diclofenac and naproxen even in concentrations of the order of mg/L as shown in this work. Some operational conditions should be improved like the HRT. In this work, an HRT of 3 days was adequate but could be optimized seeking the highest removal efficiency. This work highlights the importance of considering the chemical interactions between pharmaceuticals and with the plants root which can provide information for the better understanding of the processes involved in the removal of organic pollutants within the constructed wetland. In these treatment systems, modelling of the molecular interactions by using computational systems of a higher level of theory complemented with experimental information could provide the basis to support the proposal of the mechanisms involved in the removal of organic substances in the wastewater treatment.