Introduction
Montane systems represent a useful model for analyzing the interaction of ecological and evolutionary factors on diversity patterns variation. Mountains differ in terms of age, size, historical stability, climatic conditions and topography, reason why they represent “natural laboratories” for examining the factors that promote the diversification and maintenance of biota (Graham et al., 2014). Montane biota distribution depends strongly on its current ecological and spatial structure, but also on its geographic history (Halffter, 1987; Mastretta-Yanes et al., 2015). In the dynamic processes that have given rise to the Mexican Transition Zone (MTZ), the mountains are occupied by lineages with different evolutionary history and origin than those occupying the lowlands. The MTZ temperate mountains play a main role as a penetration path for the Holarctic biota through the horizontal colonization process, where mountains were colonized during interglacial periods by lineages originated in higher latitudes. The Neotropical biota is scarcely represented on MTZ temperate mountains because of the vertical colonization process, where lineages from surrounding lowlands at the same latitude colonize mountains, being limited by physiological restrictions related to evolutionary history (Escobar et al., 2007; Halffter, 1987; Mastretta-Yanes et al., 2015; Moctezuma, Halffter et al., 2016). In addition, the MTZ mountains act as differentiation and speciation areas, especially in the case of the Transmexican Volcanic Belt (TMVB). The historical-geographic dynamic of the mountains can promote the appearance of endemism centers in fragile and naturally fragmented ecosystems, which are particularly susceptible to the impact of climatic change (García-López et al., 2013; García-Robledo et al., 2016; McCain & Colwell, 2011). In mountain systems, the beta component makes a prominent contribution to biological biodiversity because of processes of isolation and speciation (García-López et al., 2013; Mastretta-Yanes et al., 2015).
The National Park model appeared during the 19th century, as a response for conservation purposes to protect natural sources and scenic beauty of landscapes and for the benefit and enjoyment of the people. This model concept was expanded in the 1960’s and 1970’s with a strong influence of the classic theory of Island Biogeography (Losos & Ricklefs, 2010; MacArthur & Wilson, 1967; Whittaker & Fernández-Palacios, 2007). Since then, the application of Island Biogeography to the design of National Parks favors the protection of large size areas: the larger the surface of the conservation unit, the more species it will contain, and the more resilient against external changes (Higgs, 1981). Therefore, a debate focused on the relative value of single large or several small refuges (SLOSS) aroused. But the SLOSS debate concluded during the late 1980’s and nature reserve size became irrelevant although it achieves conservation goals such as the keystone species population viability, saving the largest possible fraction of a community and including corridors for facilitating gene flow and dispersal of individuals between reserves (Soulé & Simberloff, 1986).
Derivations of the island biogeography theory do not combine the ecological elements with productive activities (apart from tourism) in the planning of conservation (Halffter, 2002, 2005; Higgs, 1981; Whittaker & Fernández-Palacios, 2007). Given this limitation, UNESCO’s Man and Biosphere Program (MAB) developed and put into practice in the 1970’s with the concept of the Biosphere Reserve, has been modified over the years. In its current format, each Biosphere Reserve includes 1 or several core zones strictly dedicated to conservation, and buffer zones necessary to ensure the permanence of the core zones in the face of the influences of anthropic changes, as well as 1 or several areas dedicated to sustainable productive activities, thus making the conservation of the “natural capital” compatible with its use (Halffter, 1984, 2005). However, although the Biosphere Reserve (particularly the Mexican modality; Halffter, 1984) was an innovative approach in many aspects, they maintain the same approach as modern National Parks in terms of design, favoring large areas for conservation purposes. A failure of the Biosphere Reserve and the National Park models lies in the fact that both area types were not conceived considering the complementarity between the alpha, beta and gamma components of biological diversity.
Faced with the need to protect ecosystems where even an extensive area cannot comprise all of the regional diversity because of a high species turnover (e.g., mountain systems), the Archipelago Reserve model arises (Halffter, 2005, 2007). The Archipelago Reserve model is defined as a broad group of protected areas that seek to fully represent regional diversity through complementarity. In a social and political context, the Archipelago Reserve aims to be a driver to change the normativity models for land ownership and for currently protected areas, to increase interconnectivity between fragile ecosystem remnants and fragments. The Archipelago Reserve model seeks to protect traditional and rustic agroecosystems that conserve a high biodiversity, enhancing the appreciation of productive practices and achieving the sustainable development (Halffter, 2007; Peresbarbosa et al., 2007).
Due to the lack of knowledge about montane diversity patterns and its different components in the design of protected areas, it is necessary to reconsider the configuration strategy of these areas. In this article, we suggest a protection model taking as a study case some mountains of the eastern TMVB and the southern Sierra Madre Oriental. We reanalyzed the information generated in this mountain area using dung beetles as a focal group in previous biodiversity research (Arriaga-Jiménez et al., 2018; Moctezuma, Halffter et al., 2016). Therefore, a series of questions developed: How are the diversity patterns of insect communities in these mountain systems? What are the geographic factors influencing these diversity patterns? What would be the most suitable strategy for the conservation of diversity, according to the observed results? These ideas lead us to discuss the best conservation model for the mountain ranges of central Mexico and ecosystems with similar diversity patterns.
Materials and methods
As a study area, we chose the eastern part of the TMVB (Fig. 1). This is a mountain chain in which hundreds of volcanic structures are present, with different ages (from the Miocene to the present) and ecological conditions (Mastretta-Yanes et al., 2015). The TMVB has presented periods of fragmentation and vicariance, with subsequent reconnection and colonization during interglacial events (Arriaga-Jiménez et al., 2016; Halffter, 1987; Mastretta-Yanes et al., 2015; Moctezuma, Halffter et al., 2016). Therefore, the TMVB represents for the North American montane entomofauna a colonization route and an important center of endemism and diversification, as well as presenting naturally fragmented ecosystems (Halffter, 1987; Corona et al., 2007; Escalante et al., 2009; Ruiz-Sánchez & Specht, 2013).

Figure 1 Study sites (Arriaga-Jiménez et al., 2018; Moctezuma, Halffter et al., 2016) and protected areas in the eastern part of the Transmexican Volcanic Belt: a) orographic landscape features highlighting some of the highest mountains, b) regional land use. Unprotected mountains and native vegetation could be utilized to establish a system of interconnected islands in an Archipelago Reserve for the study area.
The eastern region of the TMVB encompasses some of the highest peaks and largest forested areas of this mountain chain, including Pico de Orizaba (5,640 m), Sierra Negra (4,580 m), La Malinche (4,440 m), Cofre de Perote (4,220 m), Las Derrumbadas (3,485 m) and El Pinal (3,280 m). Three important protected areas have been established in this region: La Malinche National Park (45,711 ha), Pico de Orizaba National Park (19,601 ha) and Cofre de Perote National Park (11,550 ha) (Inafed, 2010; Neira-Jáuregui, 2012; Semarnat & Conanp, 2016).
Dung beetles (Coleoptera: Scarabaeinae, Aphodiinae and Geotrupidae) are a highly diverse group of insects with high functional value for productive systems: they perform cleansing and fertilization of the soil, bioturbation, nutrient cycling, pest control and seed dispersion (Beynon et al., 2015; Nichols et al., 2008; Ridsdill-Smith & Edwards, 2011; Scholtz et al., 2009). In addition, dung beetles can be evaluated with standardized methods for the study of diversity (Favila & Halffter, 1997; Halffter & Favila, 1993; Nichols & Gardner, 2011; Spector, 2006). A considerable knowledge of dung beetles in the eastern region of the TMVB has been achieved, thanks to decades of intensive collection (Arriaga-Jiménez et al., 2016, 2018; Escobar et al., 2007; Halffter et al., 1995; Lobo & Halffter, 2000; Martín-Piera & Lobo, 1993; Moctezuma, Halffter et al., 2016; Moctezuma, Rossini et al., 2016; Sánchez-Huerta et al., 2015). As a result, we used information available in Moctezuma, Halffter et al. (2016) and Arriaga-Jiménez et al. (2018) that was obtained with similar methodology in 6 different mountains (Fig. 1): La Malinche, El Pinal, Las Derrumbadas, Cofre de Perote, Pico de Orizaba and Sierra Negra. On each mountain, 2 study sites were located on the eastern slope and 2 on the western slope (Table 1).
Table 1 Geographic characteristics of the study sites located in the mountains of the Transmexican Volcanic Belt in Central Mexico, and information of protection status is included. NP: Protected by National Park.
Site | Mountain | Slope | Elevation | Latitude | Longitude | NP | |
1 | Cofre de Perote | Eastern | 2700 | 19°25’00” N | 97°08’40” | W | no |
2 | Cofre de Perote | Eastern | 3200 | 19°30’05” N | 97°07’15” | W | yes |
3 | Cofre de Perote | Western | 2700 | 19°30’04” N | 97°13’47” | W | no |
4 | Cofre de Perote | Western | 3400 | 19°27’40” N | 97°10’43” | W | yes |
5 | La Malinche | Eastern | 2800 | 19°15’58” N | 97°58’07” | W | yes |
6 | La Malinche | Eastern | 3200 | 19°14’38” N | 97°59’35” | W | yes |
7 | La Malinche | Western | 2800 | 19°14’35” N | 98°06’10” | W | yes |
8 | La Malinche | Western | 3400 | 19°15’29” N | 98°01’46” | W | yes |
9 | Pico de Orizaba | Eastern | 2600 | 19°02’16” N | 97°10’23” | W | no |
10 | Pico de Orizaba | Eastern | 3300 | 19°02’40” N | 97°12’05” | W | no |
11 | Pico de Orizaba | Western | 2800 | 19°03’37”N | 97°23’25” | W | no |
12 | Pico de Orizaba | Western | 3400 | 19°04’18” N | 97°19’02” | W | yes |
13 | Sierra Negra | Eastern | 2800 | 18°54’42” N | 97°18’43” | W | no |
14 | Sierra Negra | Eastern | 3400 | 18°57’40” N | 97°17’34” | W | yes |
15 | Sierra Negra | Western | 2800 | 18°59’28” N | 97°24’29” | W | no |
16 | Sierra Negra | Western | 3300 | 19°00’34” N | 97°20’39” | W | yes |
17 | El Pinal | Eastern | 2700 | 19°08’59” N | 97°54’01” | W | no |
18 | El Pinal | Eastern | 2900 | 19°09’12” N | 97°54’49” | W | no |
19 | El Pinal | Western | 2600 | 19°07’50” N | 97°55’24” | W | no |
20 | El Pinal | Western | 3000 | 19°08’47” N | 97°54’44” | W | no |
21 | Las Derrumbadas | Eastern | 2500 | 19°17’32” N | 97°26’43” | W | no |
22 | Las Derrumbadas | Eastern | 2800 | 19°17’02” N | 97°27’56” | W | no |
23 | Las Derrumbadas | Western | 2800 | 19°16’38” N | 97°28’07” | W | no |
24 | Las Derrumbadas | Western | 2600 | 19°15’58” N | 97°28’20” | W | no |
In order to evaluate the completeness of the inventories, we performed the method of sample coverage (Ĉm). This produces an estimate of the proportion of the total number of individuals of a community that belongs to the species represented in the sample, and allows direct comparison among samples of the same completeness, with a lower bias than traditional rarefaction methods. As a result, Ĉm allows robust estimates of the biodiversity of communities to be produced (Chao & Jost, 2012; Chao et al., 2014).
In order to determine diversity patterns, we estimated species richness. This parameter is considered a diversity measure ( q D) of the order q = 0, forming part of the Hill Numbers, and one that is insensitive to species frequency (Hill, 1973; Jost, 2006). We contrasted the richness ( 0 D) observed on each mountain, in protected and unprotected areas, with the regional diversity of the TMVB, through a diversity accumulation curve (Chao et al., 2014; Colwell et al., 2012). In order to estimate the magnitude of the contribution of the local diversity and of the turnover of species in the TMVB, we performed a multiplicative partition of diversity (Jost, 2007; Jost et al., 2010; Whittaker, 1972):
where 0Dγ: gamma diversity, 0Dα: alpha diversity, 0Dβ: beta diversity.
We considered 0Dγ to be the regional diversity of the TMVB, 0Dα the mean diversity per mountain and 0Dβ the species turnover between mountains. We calculated the 95% confidence intervals for all of the estimates of diversity (CI95%), given that these allow statistically rigorous comparisons (Colwell et al., 2012; Wasserstein & Lazar, 2016). We used the packages INEXT and VEGETARIAN with 1000 randomizations as parameters (Charney & Record, 2014; Hsieh et al., 2013).
Since the study sites present variable geographic conditions (different mountains, elevations and slopes), we used a Permanova to determine which factor could best explain the variation of the beta diversity (0Dβ ). The Permanova produces the partition of the dissimilarity originating from different sources of variation, and uses tests of permutation to evaluate their significance by generating pseudo-F (equivalent to the Fisher F), a value of probability and an R2 value (effect size) which shows the percentage of variation explained by the supplied sources of variation. We used the package VEGAN, the Jaccard index as a source of dissimilarity and 1,000 permutations at a level of α = 0.05 (Anderson, 2001; Anderson et al., 2006; Oksanen, 2015). We used a principle coordinates analysis (PCoA) in order to observe the differences in composition of species. Another variable that could be related to species turnover but cannot be included in the Permanova model is distance between sites. We therefore compared the matrix of dissimilarity to the distance between sites using a Pearson correlation. All analyses were performed with the program R (R Development Core Team, 2017).
Results
The inventories had a good sample coverage (regional = 0.999, La Malinche = 0.999, El Pinal = 0.999, Las Derrumbadas = 1, Cofre de Perote = 0.997, Pico de Orizaba = 0.998, Sierra Negra = 0.992). We estimated a regional diversity (0Dγ ) of 36 ± 1.16 species, a mean alpha diversity (0Dα ) per mountain equal to 13.67 ± 0.32 species and a turnover or beta diversity (0Dβ ) equal to 2.63 ± 0.07. Mean alpha diversity per mountain represents only 38% of the regional diversity.
The estimates of species richness at the local level were very variable; however, all of the mountains of the TMBV examined here had a lower diversity than expected for a sample taken at random from the regional “pool” of species. The volcanoes Pico de Orizaba and Cofre de Perote presented the highest species richness of the region, while Las Derrumbadas presented the lowest. Sites included in national parks present lower species richness than those located outside these areas, and a lower richness than expected by chance (Fig. 2).
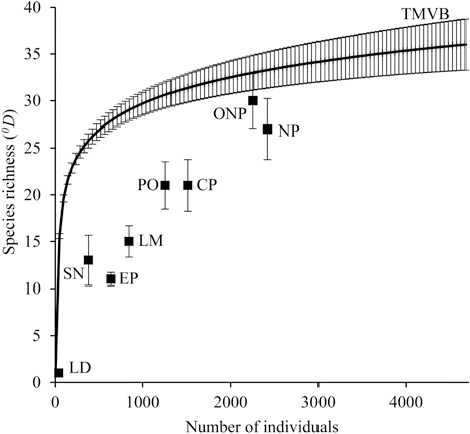
Figure 2 Species richness at regional level (continuous line) and local (squares) in the eastern region of the Transmexican Volcanic Belt. In general terms, the diversity of the mountains is significantly lower than the regional diversity. The error bars represent 95% confidence intervals. TMVB: Transmexican Volcanic Belt (0D=36 ± 2.74), LM: La Malinche (0D =15 ± 1.67), EP: El Pinal (0D = 11 ± 0.7), LD: Las Derrumbadas (0D =1 ± 0), CP: Cofre de Perote (0D = 21 ± 2.77), PO: Pico de Orizaba (0D = 21 ± 2.53), SN: Sierra Negra (0D = 13 ± 2.66), NP: National Parks (0D = 27 ± 3.23), ONP: outside of the National Parks (0D = 30 ± 2.89).
We found differences in the sources of variation for the beta diversity (Table 2). It appears that the determinant factor for species turnover are the distinct mountains, which explains a majority of the study system variation (R2 = 0.51, p < 0.001). Elevation was statistically significant (p = 0.005), but its effect size is low (R2 = 0.06). We did not find a significant effect for the factor slope or for the interactions between factors (Fig. 3). On comparison of the influence of geographic distance on the dissimilarity among sites (Fig. 4), we did not find a significant relationship between the 2 variables (rho = 0.042, p = 0.49).
Table 2 Permanova for the beta diversity considering 3 geographic variables. We used the Jaccard index as a source of dissimilarity and 1000 permutations as a parameter.
Source of variation | df | Sum of Sqs | Mean Sqs | Pseudo-F | R 2 | p |
Mountain | 5 | 4.112 | 0.8224 | 4.715 | 0.51 | < 0.001 |
Elevation | 1 | 0.541 | 0.541 | 3.104 | 0.067 | 0.005 |
Slope | 1 | 0.291 | 0.291 | 1.668 | 0.036 | 0.117 |
Mountain: elevation | 5 | 1.166 | 0.233 | 1.337 | 0.145 | 0.154 |
Mountain: slope | 5 | 0.863 | 0.173 | 0.989 | 0.107 | 0.502 |
Elevation: slope | 1 | 0.221 | 0.221 | 1.268 | 0.027 | 0.244 |
Residuals | 5 | 0.872 | 0.174 | 0.108 | ||
Total | 23 | 8.066 | 1 |

Figure 3 The evaluation of the beta diversity demonstrated that the difference between different mountains could have a large influence on the patterns of diversity observed, since it explains more than 50% of the variation estimated in the eastern region of the Transmexican Volcanic Belt. Given the fact that the position of the objects within the PCoA graph implies dissimilarity, we observed high dissimilarity within a group formed by 2 mountains (CP, PO). LM: La Malinche, EP: El Pinal, LD: Las Derrumbadas, CP: Cofre de Perote, PO: Pico de Orizaba, SN: Sierra Negra.
Discussion
With this study, we can infer that temperate National Parks are not particularly efficient in terms of protecting the montane entomofauna in Mexico. This conclusion is based on the fact that National Parks are established in large areas overlapped in 1 or 2 mountains. In addition, they generally have their lower limit at very high elevations, leaving the zones below unprotected. We observed that national parks established on adjacent mountains (Pico de Orizaba and Cofre de Perote national parks) could protect redundant insect communities presenting a low dissimilarity in their species communities. Our study reveals that beta diversity patterns and complementarity among sites in a mountain system could represent a first step to detect a suitable region to establish an Archipelago Reserve. Nevertheless, future spatial analysis that match beta diversity patterns and current protected area coverage, are required in order establish the best spatial configuration for future Archipelago Reserve (Arriaga-Jiménez et al., 2018).
Individual mountains make a significant contribution to regional diversity, because of the high species turnover among them. Indeed, it is possible that we may be severely underestimating the species turnover in the Transmexican Volcanic Belt. Our study included 6 mountains with highly complete dung beetle inventories, although the Transmexican Volcanic Belt encompasses hundreds of different volcanic structures with distinct elevations, isolation degrees, geological ages and ecological conditions that have never been sampled (Mastretta-Yanes et al., 2015). If this assumption is confirmed, the protection of regional diversity provided by the national parks could be very deficient since these constructs leave a large number of montane insect species and communities unprotected.
In our study, we found a high species turnover associated with the change among mountains: each mountain represents a unique habitat hosting a highly differentiated community. This result is explained because during interglacial periods species can be isolated in the mountains, triggering the process of vicariance and leading to unique insect communities (Arriaga-Jiménez et al., 2016; Kohlmann et al., 2018; Lobo & Halffter, 2000; Mastretta-Yanes et al., 2015; Moctezuma, Halffter et al., 2016). It has been observed that under this scenario a factor such as elevation has a limited role on our study area as a driver of species turnover (Escobar et al., 2007; Lobo & Halffter, 2000; Moctezuma, Halffter et al., 2016). Our results refer only to species richness at a regional level, reason why the difference in elevation does not influence largely the absence/presence of species. Elevation significantly modifies the ensemble of species at local level.
The patterns of a high species turnover observed in the Transmexican Volcanic Belt are not exclusive to dung beetles. These patterns can be observed in other groups, such as other Coleoptera (Corona et al., 2007; Gutiérrez-Velázquez et al., 2012; Marshall & Liebherr, 2000; Morón, 2013), fleas (Morrone & Gutiérrez, 2005), mammals (Escalante et al., 2004; Gámez et al., 2012; Rodríguez et al., 2003), and vascular plants (Rzedowski, 2005; Suárez-Mota et al., 2013). Consequently, the low local diversity found for different taxa in the national parks of the Transmexican Volcanic Belt is a reality.
In our study, we did not find any association between distance increase and beta diversity among locations. At a small geographic scale, a complete turnover of species can be presented. In Mexico, high beta diversity patters have been recognized for different biotic groups and geographic regions, leading to the hypothesis of Mexico as a beta-diverse country (Arita, 1993, 1997; Arita & León-Paniagua, 1993; García-de Jesús et al., 2016; Moreno & Halffter, 2001; Rodríguez et al., 2003; Sarukhán et al., 1996). This is important given the fact that beta diversity must be considered a priority in the selection of conservation sites, at both large and small scales. Beta diversity is not a characteristic exclusive to Mexico or to its mountain systems, Melo et al. (2009) reported the highest beta diversity of the Americas in mountain areas (western North America, Central America and the Andes).
High beta diversity patterns have also been reported for highly endangered and fragmented ecosystems and agroecosystems, as is the case of the cloud mountain forests and shade coffee plantations (Pineda & Halffter, 2004; Pineda et al., 2005; Rös et al., 2012; Williams-Linera et al., 2007). Previous studies have proposed that cloud forest fragments, secondary forests and traditional coffee plantations can preserve complementary regional biodiversity (Williams-Linera et al., 2007). Rös et al. (2012) and suggested that Archipelago reserves could lead to the integration of heterogeneous landscapes, ensuring cloud mountain forest protection and the sustainable development. Bandeira et al. (2005) reported that a combination of factors, such as human management, original stand cover and development stage, can promote a high beta diversity in a coffee plantations system.
The Brazilian Atlantic Forest is one of the most fragmented biodiversity hotspots, but a high beta diversity among fragments allows for the permanence of regional species pool (Beca et al., 2017; Da Silva & Medina, 2016; Filgueiras et al., 2010). The Archipelago Reserve model may be able to protect the Atlantic forest fragments and to enhance connectivity through restoration measures (Beca et al., 2017). Human-disturbed and fragmented Mesoamerican tropical rain forests can be candidates to be adequately protected under the Archipelago Reserve model, since they can support fragile communities that maintain regional diversity (Arroyo-Rodríguez et al., 2012, 2013; Garmendia et al., 2013; Navarrete & Halffter, 2008; Sánchez-de Jesús et al., 2016). Archipelago reserves could also help protect the Madagascan tropical forests, which are recognized by its endemic and highly diverse biota. Studies have reported a high beta for Malagasy ant and dung beetle communities (Fisher, 1996; Viljanen et al., 2010). The Archipelago reserves could be suitable to protect transition zones between biogeographic regions (Ferro & Morrone, 2014), since they are characterized by the presentation of a high species turnover. This transition zones not only are outstanding by their beta diversity, but they are expected to preserve high phylogenetic diversity and an important number of endemisms (Ferro & Morrone, 2014; Kreft & Jetz, 2013). Therefore, transition zones should be considered a priority for world conservation strategies.
Beta-diverse ecosystems are under strong pressure from anthropic activities. However, productive and non-productive schemes can contribute to conserve insect communities within a landscape, since a diverse mosaic of agro-environmental schemes can provide a large species pool while guarantee financial benefits from production and subsides (Mader et al., 2017). Consequently, promotion of low impact activities under a scheme of conservation is vital. Biosphere Reserves allow an approach towards the sustainable use of natural resources; however, they lack the appropriate design for protecting regions with high species turnover (Halffter 1984, 2002, 2005, 2007), because they are designed to protect zones with a high local diversity (Halffter, 2007). We therefore consider that the establishment of Archipelago reserves in mountain or beta-diverse ecosystems is an urgent requirement and a priority in terms of conservation. In cases such as those discussed here, Archipelago reserves must have complementarity among their components in order to increase the total diversity under protection, with the inclusion of different local communities.
From a biological and a social point of view, the Archipelago reserves are a new and attractive proposal for biodiversity conservation. Both national parks and biosphere reserves sought to protect areas (medium or large) with exceptional richness and characteristics, while Archipelago reserves seek to protect a set of complementary areas, which together represent a unique biota. Archipelago reserves are an ecological research application facing specific problems, such as those discussed in this article. They are not intended to replace the approach of a larger area, National Park or Biosphere Reserve, but to be supplementary to them (Halffter 2007).
The creation process of Archipelago reserves is still incipient. Existing national parks could be integrated into a system of Archipelago reserves, establishing corridors of connection to protect fragments of natural vegetation, riparian zones, canyons, secondary forests and traditionally productive systems that have been shown to be important refuges of biodiversity (Arellano et al., 2004; Chazdon, 2014; Filgueiras et al., 2015; Gray et al., 2016; Pineda et al., 2005; Rös et al., 2012; Verdú et al., 2007). The first worldwide implementation of the Archipelago Reserve has been developed in the city of Xalapa (Veracruz, Mexico-2015), in which it is intended to protect the fragile remnants of the tropical cloud forest and productive ecosystems (specially shade coffee plantations) through a set of vegetation islands. We hope that this work can promote the implementation of the Archipelago Reserve on the Transmexican Volcanic Belt, particularly for ensuring insect conservation. Additionally, we aim to encourage the replication of this conservation model in landscapes with high beta diversity, where they can complement the already existing natural reserves.