Servicios Personalizados
Revista
Articulo
Indicadores
-
Citado por SciELO
-
Accesos
Links relacionados
-
Similares en SciELO
Compartir
Revista mexicana de ciencias agrícolas
versión impresa ISSN 2007-0934
Rev. Mex. Cienc. Agríc vol.11 no.5 Texcoco jun./ago. 2020 Epub 03-Oct-2021
https://doi.org/10.29312/remexca.v11i5.2272
Articles
Biological control of Lasiodiplodia theobromae and Fomitopsis meliae causing the regressive death of citrus
1Universidad Autónoma de Nuevo León. Av. Universidad s/n, Ciudad Universitaria, San Nicolás de los Garza, Nuevo León. México. CP. 66455. (omaralvarado085@gmail.com; emolivares@gmail.com).
2Instituto Dominicano de Investigaciones Agropecuarias y Forestales. Calle Rafael Augusto Sánchez No. 89, Ensanche Evaristo Morales, Santo Domingo, República Dominicana. (polancolaura7@gmail.com).
3Biociencia SA de CV. Monterrey, Nuevo León. México. (biociencia01@prodigy.net.mx).
The research was carried out in the state of Nuevo León, Mexico, during 2018, with the aim of isolating and identifying strains of soil microorganisms and evaluating their antagonistic capacity in vitro against citrus pathogens Lasiodiplodia theobromae and Fomitopsis meliae. For the isolation of the microorganisms, 17 rhizosphere samplings were carried out in different locations and types of vegetation; the samples were heat treated and spread by extension in the potato dextrose agar culture medium added with 0.3% yeast extract and 0.3% malt extract (PDLMA). The isolates obtained were preliminarily evaluated by observation and growth comparison. Of 70 isolates, 15 presented antagonistic activity, which showed inhibition percentages of 39-91%. The actinomycete strains M4 R and M1-101, and the fungus M104 (Penicillium citrinum) caused 84, 91 and 85% inhibition respectively against L. theobromae and 83, 91 and 91% respectively against F. meliae. Isolation of bacteria was achieved, actinomycetes and fungi from the soil capable of inhibiting the growth of L. theobromae and F. meliae fungi causing regressive citrus death.
Keywords: Fomitopsis meliae; Lasiodiplodia theobromae; antagonism; inhibition
La investigación fue realizada en el estado de Nuevo León, México, durante el año 2018, con el objetivo de aislar e identificar cepas de microorganismos de suelo y evaluar su capacidad antagónica in vitro frente a los patógenos de cítricos Lasiodiplodia theobromae y Fomitopsis meliae. Para el aislamiento de los microorganismos se realizaron 17 muestreos de rizósfera en diferentes localidades y tipos de vegetación; las muestras se trataron con calor y se sembraron por extensión en el medio de cultivo papa dextrosa agar adicionado con 0.3% de extracto levadura y 0.3% de extracto de malta (PDLMA). Los aislamientos obtenidos se evaluaron preliminarmente por observación y confrontación del crecimiento. De 70 cepas aisladas, 15 presentaron actividad antagónica, las cuales mostraron porcentajes de inhibición de 39-91%. Las cepas de los actinomicetos M4 R y M1-101, y el hongo M104 (Penicillium citrinum) causaron inhibición de 84, 91 y 85% respectivamente contra L. theobromae y de 83, 91 y 91% respectivamente contra F. meliae. Se logró el aislamiento de bacterias, actinomicetos y hongos del suelo capaces de inhibir el crecimiento de los hongos L. theobromae y F. meliae causantes de muerte regresiva de los cítricos.
Palabras clave: Fomitopsis meliae; Lasiodiplodia theobromae; antagonismo; inhibición
Introduction
Citrus fruits (Citrus spp.) are considered the universal fruit since they are present in more than 100 countries and are the most economically important group of fruits, covering 20% of the world fruit market. Currently, the majority of the citrus harvest comes from 10 countries that contribute 77% of production, of which China and Brazil produce 42% and Mexico occupies the fourth position after the USA (Lerma et al., 2015).
Diseases are a limiting factor in the production of these crops in the producing areas of Mexico, caused by around 50 pathogens that cause various symptoms such as gummy, cracking, fruit drop, greasy spot, scab, death of branches, among others. (Orozco et al., 2013). The fungus Lasiodiplodia spp. has been reported as causing regressive death and other symptoms in citrus and in a wide range of crops in various countries (Adesemoye et al., 2014; Rodríguez et al., 2016).
Recently Polanco et al. (2019) reported to Lasiodiplodia theobromae and Fomitopsis meliae as the fungi that cause the regressive death of citrus in trees in orchards in the states of Nuevo León and Tamaulipas, Mexico. In general, the method of controlling this fungus is through applications of synthetic fungicides. Chemical fungicides can produce side effects such as resistance to pests and diseases, the reduction of populations of beneficial microorganisms and contamination of the environment (Adeniyi et al., 2013; Tovar et al., 2013; Alvárez, 2015).
The agents used for biocontrol significantly reduce the populations of phytopathogens and with their use it is intended to balance the ecological disturbances caused by agrochemicals (Badii and Abreu, 2006) through various mechanisms such as the production of toxins (Carisse et al., 2001), the competition for nutrients and space (Mutawila et al., 2011), inhibition of mycelial growth of the pathogen and mycoparasitism (Cheng et al., 2012).
Among the groups of microorganisms used for biocontrol are actinomycetes, which have been extensively studied for their ability to produce antibiotic and antifungal compounds (Lee et al., 2002), especially against plant pathogens of agricultural importance (Sreevidya and Gopalakrishnan 2012; Abdallah et al., 2013; Dávila et al., 2013). Actinomycetes are gram positive bacteria, generally found in soil, rivers, lakes, decomposing organic material, among other places. Many are saprophytes and some species are pathogenic (Quiñones et al., 2016), as is the case of Streptomyces scabiei causing common scabies from potato cultivation.
For several decades, the number of actinomycetes used in agriculture has been constantly increasing as a means of controlling or reducing plant pathogen populations (Dávila et al., 2013). Although the microorganisms have been extensively studied in the control of phytopathogenic fungi, few works have been carried out in the control of Lasiodiplodia theobromae and Fomitopsis meliae fungi. Sajitha and Florence (2013); Kamil et al. (2018), evaluated the antagonistic capacity of actinomycetes for the control of Lasiodiplodia theobromae causing damage to rubber tree wood and the regressive death of mango plants, respectively, with good results; in addition Caro et al. (2019) working with actinomycetes obtained promising results for the control of Lasiodiplodia sp., in potato cultivation.
Even so, there is little that is known of control works of the main fungi that cause the regressive death of critics, Lasiodiplodia theobromae and Fomitopsis meliae, which are normally treated with the use of chemical products or sanitary practices of the culture (pruning). Therefore, the objective of this work was to isolate strains of soil microorganisms and evaluate their antagonistic capacity in vitro against the phytopathogens Lasiodiplodia theobromae and Fomitopsis meliae, causing the regressive death of citrus fruits.
Materials and methods
Phytopathogenic fungal strains
The fungal strains Lasiodiplodia theobromae and Fomitopsis meliae were provided by the Faculty of Agronomy of the Autonomous University of Nuevo León (FAUANL).
Isolation of microorganisms from the soil
17 soil samplings with different types of vegetation were carried out in areas of the state of Nuevo León (Table 1), samples of the rhizosphere were collected at a depth of 10 to 15 cm, after removing the surface layer. For sampling, five different points were randomly selected to form a sample. Samples were placed in plastic bags, sealed, and stored in the laboratory in cool weather conditions.
Table 1 List of sampling locations and predominant vegetation type.
Location | Coordinates | Type of vegetation |
FAUANL, Marin Campus, Marin Municipality | 25° 52’ 25.76” north latitude; 100° 3’ 21.25” west latitude | Citrus cultivation and wasteland |
FAUANL, La Ascensión Campus, Aramberri municipality | 24.32° north latitude; 99.91° west latitude | Garlic cultivation |
Mina municipality | 25° 52’ a 26° 44’ north latitude; 100° 26’ a 101° 13’ west latitude | Wild plants |
One gram of each sample was dissolved in 9 mL of sterile distilled water. The dilutions were heat treated (50 °C) for 10 min. Serial dilutions 1:100, 1:1 000 and 1:10 000 were prepared from each suspension. From each dilution 100 µL were transferred to Petri dishes with potato dextrose agar added with yeast extract (0.3%) and malt extract (0.3 %) (PDLMA) using the extension method. Petri dishes were incubated at 25 ±2 °C. Microorganisms exhibiting inhibition halos were isolated and purified (Oskay et al., 2004).
Morphological and genetic characterization of actinomycetes and bacteria with antagonistic activity
Actinomycete strains were identified according to their macroscopic growth characteristics (shape, color of aerial mycelium, texture, and pigment production in the culture medium). Additionally, microscopic observations were made using Gram stain. For the identification of the bacteria, the shape, color and texture of the colonies were considered, for the microscopic visualization the Gram stain was used.
For molecular identification of actinomycetes, DNA was extracted using the DNeasy Plant Mini Kit™ (Qiagen Inc.) and amplified by PCR using primers 8F (5’-agagtttgatcctggctcag-3’) and 1492R (5’-ggttaccttgttacgactt-3’) (Sacchi et al., 2002). Amplification reactions were carried out in a Thermo™ thermocycler, which consisted of denaturation at 94 ºC for 1 min, followed by 35 cycles at 94 ºC for 30 s, ringing at 48 ºC for 50 s, and extension at 72 ºC. for 80 s and a final extension at 72 ºC for 4 min. Products were visualized by 1% agarose gel electrophoresis stained with 0.5 ng µl-1 of ethidium bromide. The ladder-100™ (Axygen) molecular weight marker was used.
Morphological and genetic characterization of fungi with antagonistic activity
The morphological identification of the isolates obtained was based on the characteristics of the colonies (color, type and shape) in addition to the morphology of conidia and conidiophores using an optical microscope (Urbaez et al., 2013). In addition, lamella mounts were made using the cotton blue lactophenol dye as a solution, and the particular characteristics of the structures were described. Fungi were identified following the criteria of Barnett and Hunter (1998); Phillips et al. (2013).
For genetic characterization, genomic DNA was extracted from mycelium following the instructions of the manufacturer of the DNeasy Plant Mini Kit™ (Qiagen Inc.). DNA was quantified with a Take3™ spectrophotometer (Bioselec). The ITS-1 region, the 5.8S ribosomal gene and ITS-2 were amplified by PCR using the ITS-1fu 5’-tccgtaggtgaacctgcgg-3’ and ITS-4 5’-tcctccgcttatttgatatgc-3’ primers (White et al., 1990). Amplification reactions were carried out in a Termo™ thermocycler, which consisted of denaturation at 94 ºC for 1 min, ringing at 55 ºC for 25 s, polymerization at 72 ºC for 50 s, with 35 cycles, followed by a final extension at 72 ºC for 4 min. The PCR products were sequenced and compared with GenBank sequences to confirm the identity of the strains at the species level.
In vitro antagonism test
A preliminary test was carried out to select the strains that presented the greatest inhibitory effect on pathogenic fungi. The confrontations were carried out with each strain of the microorganisms isolated against the phytopathogenic fungi L. theobromae and F. meliae. A hoe of each of the microorganisms was seeded at the four cardinal points (3 cm distance from the center, in Petri dishes) in the PDLMA medium. Forty-eight hours later, a 0.5 cm growth explant from each plant pathogen fungus was placed in the center. The boxes were incubated at 25 ±2 ºC for 5 days. Each microorganism was taken as a treatment with three repetitions, considering each Petri dish as one repetition and a negative control with each fungus without antagonist. The test was carried out twice.
The inhibitory capacity of the microorganisms was quantified by measuring the diameter of the fungal growth with a vernier, using two cardinal diameters previously drawn on the bottom of each Petri dish as a reference.
The percent inhibition was calculated using the following formula: (%) inhibition = [(A1-A2)/A1] x 100. Where: A1= growth of the phytopathogen colony (control); A2= growth of the fungal colony of the phytopathogen growing against the actinomycete.
Based on the results of the preliminary tests of the in vitro confrontations, the M1-101 and M104 strains were selected for a second test because they presented the highest inhibition values. The antagonist strains were grown in potato dextrose broth added with yeast extract and 0.3% malt extract (CPDLM) and incubated at 25 ±2 ºC and 125 rpm for 10 days. Subsequently, the content was centrifuged, filtered and placed in sterile bottles.
The inhibition activity of each antagonist was evaluated at concentrations of 25, 50 and 100% of the filtrate. The technique consisted of spreading 100 µl of the antagonist by extension. Subsequently, a 5 mm disk of the mycelial growth of the plant pathogen was placed in the center of the Petri dish. In addition, each antagonist was confronted with the two phytopathogenic fungi at the same time, for which 100 µl of the antagonist were spread by the box extension method and immediately a disc of mycelial growth of each fungus of 5 mm diameter was placed at a 4 cm distance between them. Daily observations were made for five days, at the end of which the mycelial growth of the fungi was measured, when the growth of the control completely covered the Petri dish.
Inhibition of germination of conidia and mycelium
Based on the preliminary results, the M1-101 strain was selected for the conidia and mycelium germination inhibition test of the fungi F. meliae and L. theobromae, respectively. The M1-101 strain was seeded in 50 ml of CPDLM broth by adding a square of the strain on agar of approximately 1 cm2 for each Erlenmeyer flask 250 mL fired. The flasks were incubated with shaking at 125 rpm and 25 ± 2ºC for 10 days. At the end of the incubation period, the culture was filtered and the filtrate was diluted to concentrations of 25, 50 and 100%.
For the preparation of the inoculum of the phytopathogenic fungi, strains incubated for 6 days in potato dextrose agar (PDA) were used. 10 ml of sterile distilled water was added to each Petri dish to resuspend F. meliae conidia and L. theobromae mycelium by scraping the surface of the box with a bacteriological loop. The inoculum was preserved in 15 ml conical tubes.
Germination inhibition activity of the antagonist was evaluated at concentrations of 25, 50 and 100%. The technique consisted of spreading, with the help of a glass handle, 100 µl of each concentration in each Petri dish. After absorption, 100 µl of the inoculum of the plant pathogens was added to the previously prepared solution (conidia and mycelium) of the plant pathogens. Three replicates of each concentration were evaluated and the Petri dishes were incubated at 25 ±2 ºC. Observations were made every 24 h to see the inhibition of conidia germination and possible changes in mycelial morphology.
The count of germinated and non-germinated conidia was performed using a compound microscope (Leica DM500). 100 conidia cm-2 were counted, 500 conidia in total (germinated and non-germinated). The following formula was used to determine the percentage of germinated conidia: % germination = (a/a + b) x 100. Where: a= number of germinated conidia; and b= number of conidia without germinating. With L. theobromae, only the changes in mycelial morphology induced by the presence of the antifungal compounds produced by the antagonist were observed at a microscopic level.
Results
70 strains of microorganisms were isolated, of which 15 had inhibitory activity, and of these, 8 had typical characteristics of actinobacteria, 5 bacillary bacteria and 2 fungi (Table 2).
Table 2 Microorganisms isolated from soil from different areas of the state of Nuevo León with antagonistic activity.
Strains | Sampling location | Classification |
A7 | Mina | Bacillus |
A14 | Mina | Bacillus |
A18 | Mina | Bacillus |
A20 | Mina | Bacillus |
A23 | Mina | Bacillus |
CH 102 | La Ascensión | Actinomycete |
CH 103 | La Ascensión | Actinomycete |
M 104 | Marin | Fungus |
M1-101 | Marin | Actinomycete |
M2 R1 | Mina | Actinomycete |
M2 R2 | Mina | Actinomycete |
M3 | Marin | Actinomycete |
M4 | Marin | Actinomycete |
M4 R | Mina | Actinomycete |
M5-102 | Marin | Fungus |
Morphological and genetic characterization of actinomycetes and bacillary bacteria
The colonial growth characteristics of the actinomycetes in culture were dry, powdery aerial masses with irregular edges, of various pigmentations, showing shades of yellow, gray and others of orange. Under the microscope (40X) they showed the typical ramifications of actinomycetes. Gram staining revealed Gram positive sporulated streptobacilli.
The bacteria showed variable shades such as yellow and cream (Figure 1), with a watery texture and regular edges. Gram staining showed Gram positive and Gram negative rods. Molecular identification of actinomycete strains was not achieved due to a lack of PCR amplification possibly attributed to the fact that primers or primers have been used more with other bacteria and may not have sufficient specificity to bind to actinomycete sequences.
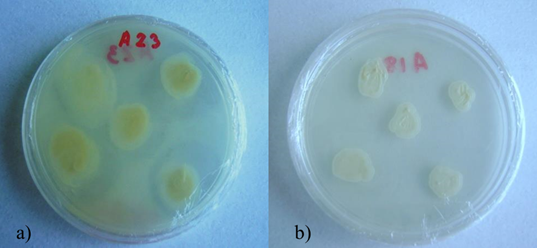
Figure 1 Bacteria isolated from the soil. a) strain A23 showing halo of inhibition; and b) strain A18.
The obtained bacillary bacteria were amplified by PCR and part of the 16S gene was sequenced. The consensus sequences of the bacillary bacteria were compared with sequences deposited in GenBank through the Blast program; strain A18 corresponded to Achromobacter xylosoxidans with 96% homology and A23 showed 96% similarity to Delftia sp.
Morphological and genetic characterization of fungi with antifungal activity
Colonies of strain M104 in culture medium were white, compact and with irregular edges, and the mycelium of strain M5-102 was brown (Figure 2). The M104 strain was identified as Penicillium sp. The characteristics of the conidiophore showed brush shape and hyaline conidia, unicellular in a cylindrical shape. The M5-102 strain presented conidiophores and aspergillary heads, hyaline, unicellular and cylindrical conidia; this strain was identified as Aspergillus sp. Morphological identification was confirmed by its DNA sequence, corresponding to Penicillium citrinum and Aspergillus sp.
In vitro antagonism test
Acromobacter xylosoxidans reduced the mycelial growth of L. theobromae 63% and F. meliae 59%. On the other hand, Delftia sp., inhibited both plant pathogenic fungi by 39%. Aspergillus sp., reduced the growth of L. theobromae and F. meliae by 83%. The strain identified as P. citrinum was able to reduce L. theobromae mycelial growth by 83% by keeping it inhibited for more than 20 days. The highest inhibition values were obtained with the fungus P. citrinum (M104) and the acinomycetes M4R, and M1-101, which showed percentages of inhibition of 85, 84 and 91% against L. theobromae and 91, 83, and 91%, against F. meliae respectively.
In the inhibition test with the different concentrations of the actinomycete and P. citrinum filtrates (25, 50 and 100%), there were statistically significant differences (p< 0.001) in the percentage of inhibition of the pathogens. The control showed the highest mycelial growth at 5 days, after the fungi were placed on PDA. L. theobromae and F. meliae fungi had a similar growth, showing no significant differences between them. The treatments that showed greater inhibition of the pathogens were L. theobromae and F. meliae against P. citrinum at 100%, followed by L. theobromae + P. citrinum and F. meliae + P. citrinum with the concentration at 100%. There were no differences between the three concentrations of the M101 strain against F. meliae. In the inhibition test with the different concentrations of the filtrates of actinomycete and P. citrinum (25, 50 and 100%), there were statistically significant differences (p= 0) in the percentage of inhibition of the pathogens.

Figure 2 Microorganisms with antifungal activity. a) colonies of strain M1-101 in PDA; b) Gram positive bacillus observed under a microscope; c) strain M5-102 in PDA; d) conidiophores and aspergillary heads of Aspergillus sp.; e) strain M104 in PDA; and f) conidiophores, phialid and conidia of P. citrinum.
Inhibition of germination of conidia
L. theobromae at 96 h of incubation completely covered the Petri dish without obtaining any reproductive structure. However, in the presence of the antagonist, L. theobromae produced dark brown chlamydospores and conidiogenous cells (Figure 3). With the confrontation, the conidia of F. meliae are inhibited and were not able to germinate, the conidia were observed to clump and thicken, tending to form chlamydospores (Figure 3).
Discussion
In this study the bacteria A. xylosoxidans was effective in reducing the in vitro growth of L. theobromae and F. melie. The ability of this bacterium to biocontrol these pathogens is comparable to the results reported by Moretti et al. (2008), who used this bacterium to control Fusarium oxysporum causing tomato wilt. Bagheri and Ahmadzadeh (2016) also reported that A. xylosoxidans can inhibit Curvularia lunata and Bipolaris sorikinian present in wheat crops.
On the other hand, some species of the Delftia genus have been reported by Han et al. (2005) inhibiting Xanthomonas oryzae pv. oryzae, Rhizoctonia solani and Pyricularia oryzae. Additionally, these bacteria play an important role in bioremediation technologies (Ubalde et al., 2012). Jergensen et al. (2009) cite Delftia with the ability to break the glycosidic bonds in chitin and peptidoglycan, which could show greater inhibition capacity of phytopathogenic fungi like those used in this study.
In general, species of the genus Aspergillus are considered as saprophytic or opportunistic fungi (Cortes and Mosqueda, 2013). Some races of Aspergillus sp. are considered as atoxigenic because they do not produce aflatoxins and have been registered as biocontrolling strains, whose mechanism of action is competitive exclusion (Cotty, 1994). Due to these qualities, an Aspergillus strain was formulated and has been successfully marketed as a biocontrol agent for the aflatoxin-producing species Aspergillus flavus (Dorner, 2009).
In this study, Aspergillus sp. was able to inhibit the growth of L. theobromae and F. meliae. These results are confirmed by the findings obtained by Adeniyi et al. (2013) who reported A. niger as a biocontrol agent for L. theobromae, although they suggest that its potential in other phytopathogens should be exploited and possible adverse effects in crops should be managed.
Despite the fact that the fungus P. citrinum can cause diseases in field crops and post-harvest products (Marquardt, 1996), according to our results, some Penicillium species also have the potential to inhibit pathogens. These results can be explained according to Quiroz et al. (2008), who reported that different species of fungi have both antagonistic and pathogenic mechanisms.
Of the 15 strains that were isolated and evaluated, actinomycetes showed the highest inhibition values (M4 R and M1-101), with M1-101 being the strain with the highest antagonistic activity, with percentages of inhibition against L. theobromae and F. meliae of 84 and 91%, respectively. Sánchez et al. (2011); Wang et al. (2015) isolated actinomycetes with the ability to inhibit agriculturally important plant pathogens such as Fusarium equiseti.
The results obtained in this study demonstrate that the ability of microorganisms to inhibit pathogens can be increased or decreased, depending on the conditions of the survival structures that the latter can produce and that the antagonist manages to reduce their growth by the production of antifungal compounds or competitive exclusion.
Conclusions
Isolation of actinomycetes was obtained from soil samples, capable of inhibiting the growth of pathogens that cause the regressive death of citrus L. theobromae and F. meliae. The M4R and M104 strains presented percentages of inhibition greater than 84% against the previous phytopathogens.
Aspergillus sp. and P. citrinum were able to reduce the growth of L. theobromae and F. meliae. Due to the antagonistic capacity demonstrated in the present study, the actinomycete strains M4R and M104 and the fungi Aspergillus sp. and P. citrinum could be considered as potential candidates to be used in L. theobromae and F. meliae control programs, but it is necessary to continue with the studies of this antagonistic capacity in vivo.
Literatura citada
Abdallah, M. E.; Haroun, S. A.; Gomah, A. A.; El-Naggar, N. E. and Badr, H. H. 2013. Application of actinomycetes as biocontrol agents in the management of onion bacterial rot diseases. Archives of Phytopathology and Plant Protection. 46(15):1797-1808. http://dx.doi.org/10.1080/03235408.2013.778451. [ Links ]
Adeniyi, D. O.; Adedeji, A. R.; Oduwaye, O. F. and Kolawolw, O. O. 2013. Evaluation of biocontrol agents against Lasiodiplodia theobromae causing inflorescence blight of cashew in Nigeria. Journal of Agriculture and Veterinary Science. 5(3):46-48. [ Links ]
Adesemoye, A. O.; Mayorquin, J. S.; Wang, D. H.; Twizeyima, M.; Lynch, S. C. and Eskalen, A. 2014. Identification of species of Botryosphaeriaceae causing bot gummosis in citrus in California. Plant Disease. 98(1):55-61. http://dx.doi.org/10.1094/PDIS-05-13-0492-RE. [ Links ]
Álvarez, L. A. 2015. Eficacia fungicida en el control de Lasiodiplodia theobromae en plantas de palto (Persea americana) con el uso del bioestimulante a base de algas Fertimar. Plagas y Enfermedades. VIII Congreso Mundial de la Palta 135-140. www.wacperu2015. [ Links ]
Badii, M. H. y Abreu, J. L. 2006. Control biológico una forma sustentable de control de plagas. International Journal of Good Conscience. 1(1):82-89. [ Links ]
Bagheri, N. and Ahmadzadeh, M. 2016. First report of Acromobacter xyloxidans on wheat rhizosphere in Iran and its biocontrol activity. Scientia Agriculturae. 16(1):36-42, DOI: 10.15192/PSCP.SA.2016.16.1.3642. [ Links ]
Barnett, H. L. and Hunter, B. B. 1998 Illustrated Genera of Imperfect Fungi. The American Phytophatological Society. Minnesota. 4ta . Ed. 217 p. [ Links ]
Carisse, O.; El Bassam, S. and Benhamou, N. 2001. Effect of Microsphaeropsis sp. strain P130A on germination and production of sclerotia of Rhizoctonia solani and interaction between the antagonism and the pathogen. Phytopathology. 91(8):782-791. https://doi.org/10.1094/PHYTO.2001.91.8.782. [ Links ]
Caro, C. J.; Mateo, T. C.; Cisneros, M. J.; Galindo, C. N. y León, Q. J. 2019. Aislamiento y selección de actinomicetos rizosféricos con actividad antagonista a fitopatógenos de la papa (Solanum tuberosum spp. andigena). Ecología Aplicada. 18(2):101-109. http://dx.doi.org/10.21704/rea.v18i2.1329. [ Links ]
Cheng, C. H., Yang, C. A. and Cheng, P. K. 2012. Antagonism of Trichoderma harzaianum ETS 323 grown in the presence of deactivated Botrytis cinerea mycelium in culture conditions. Phytopathology . 102(11):1054-1063. https://doi.org/10.1094/PHYTO-11-11-0315. [ Links ]
Cortés, S. A. D. y Mosqueda, O. T. 2013. Una mirada a los organismos fúngicos: Fábricas versátiles de diversos metabolitos secundarios de interés biotecnológico. Revista Química Viva. 12(2):64-90. http://www.redalyc.org/articulo.oa?id=86328550002. [ Links ]
Cotty, P. J. 1994. Influence of field application of an atoxigenic strain of Aspergillus flavus on the populations of A. flavus infecting cotton bolls and on the aflatoxin content of cotton seed. Phytopathology . 84(11):1270-1277. Doi: 10.1094/Phyto-84-1270. [ Links ]
Dávila, M. M. D.; Gallegos, M. G.; Hernández, C. D.; Ochoa, F. Y. M. y Flores, O. A. 2013 Actinomicetos antagónicos de importancia agrícola. Rev. Mex. Cienc. Agríc. 4(8):1187-1196. [ Links ]
Dorner, J. W. 2009. Biological control of aflatoxin contamination in corn using a nontoxigenic strain of Aspergillus flavus. Journal of Food Protection. 72(4):801-804. [ Links ]
Han, J.; Sun, L.; Dong, X.; Cai, Z.; Sun, X.; Yang, H.; Wang, Y. and Song, W. 2005. Characterization of a novel plant growth-promoting bacteria strain Delftia tsuruhatensis HR4 both as a diazotroph and a potential biocontrol agent against various plant pathogens. Systematic and Applied Microbiology. 28(1):66-76. Doi: 10.1016/j.syapm.2004.09.003. [ Links ]
Jergensen, N. O. G.; Brandt, K. K.; Nybroe, O. and Hansen, M. 2009. Delftia lacustris sp. nov., a peptidoglycan-degrading bacterium from fresh water, and emended description of Delftia tsuruhatensis as a peptidoglycan degrading bacterium. International Journal of Systematic and Evolutionary Microbiology. 59(9):2195-2199. Doi: 10.1099/ijs.0.008375-0. [ Links ]
Kamil, F. H.; Saeed, E. E.; El-Tarabily, K. A. and AbuQamar, S. F. 2018. Biological control of mango dieback disease caused by Lasiodiplodia theobromae using streptomycete and non-streptomycete actinobacteria in the United Arab Emirates. Frontiers in Microbiology. 9(829):1-19. Doi: 10.3389/fmicb.2018.00829. [ Links ]
Lee, C. H.; Kim, B. J.; Choi, G. J.; Cho, K. Y.; Yang, H.; Shin, C.; Min, S. and Lim, Y. 2002. Streptomyces with antifungal activity against rice blast causing fungus Magnaporte grisea. Journal of Microbiology and Biotechnology.12(6):1026-1028. [ Links ]
Lerma, C. J.; Ibarra, T. A.; Galván, G. V.; Martínez, D. J.; Rodríguez, R. G.; Tellez, G. V.; Fernández, S. C.; Núñez; G. M. y Galicia, C. J. 2015. Situación de la citricultura en Nuevo León. Corporación para el Desarrollo Agropecuario de Nuevo León. OEIDRUS, N. L. Washington 2000. 82 p. [ Links ]
Marquardt, R. R. 1996. Effects of molds and their toxins on livestock performance: a western Canadian perspective. Animal Feed Science and Technology. 58(1-2): 77-89. Doi: 10.1016/0377-8401(95)00875-6. [ Links ]
Moretti, M.; Giladi, G.; Gullino, M. L. and Garibaldi, A. 2008. Biological control of Achromobacter xylososydans for suppressing Fusarium wilt of tomato. International Journal of Botany. 4(4):369-375. Doi:10.3923/ijb.2008.369.375. [ Links ]
Mutawila, C.; Fourie, P. H., Halleen, F. and Mosteri, L. 2011. Grapevine cultivar variation to prunnin wound protection by Trichoderma species against trunk pathogens. Phytopahology Mediterranea. 50(supplement):274-276. [ Links ]
Orozco, S. M.; Robles, G. M.; Velázquez, M. J. J.; Manzanilla, R. M. A.; Hernández, F. L. M.; Manzo, S. G. y Nieto, A. D. 2013. Manejo integrado de las principales plagas y enfermedades en limón mexicano y limón persa. Memorias IX Simposio Internacional Citrícola. 113-171 pp. [ Links ]
Oskay, M.; Usame, T. A. and Azeri, C. 2004. Antibacterial activity of some actinomycetes isolated from farming soils of Tuley. African Journal of Biotechnology. 3(9):441-446, http://dx.doi.org/10.1016/j.funbio.2016.06.004. [ Links ]
Phillips, A. J. L.; Alves, A.; Abdollahzadeh, J.; Slippers, B.; Wingfield, M. J.; Groenewald, J. Z. and Crous, P. W. 2013. The Botryosphaeriaceae: genera and species known from culture. Studies in Micology. 76:51-167. doi:10.3114/sim0021. [ Links ]
Polanco, F. L. G.; Alvarado G. O. G.; Pérez, G. O.; González, G. R. y Olivares, S. E. 2019. Hongos asociados con la muerte regresiva de los cítricos en Nuevo León y Tamaulipas, México. Rev. Mex. Cienc. Agríc. 10(4):757-764. [ Links ]
Quiñones, A. E. E.; Evangelista, M. Z. y Rincón. E. G. 2016. Los actinomicetos y su aplicación biotecnológica. Elementos. 101:59-64. [ Links ]
Quiroz, S. V. F.; Ferrera, C. R.; Alarcón, A. y Lara, H. M. E. 2008. Antagonismo in vitro de cepas de Aspergillus y Trichoderma hacia hongos filamentosos que afectan al cultivo de ajo. Revista Mexicana de Micologia. 26:27-34. [ Links ]
Rodríguez, G. E; Guerrero, P.; Barradas, C., Crous, P. W. and Alves, A. 2016. Phylogeny and pathogenicity of Lasiodiplodia species associated with dieback of mango in Peru. Fungal Biology. 121(4):452-465. http://dx.doi.org/10.1016/j.funbio.2016.06.004. [ Links ]
Sacchi, C.T.; Whitney, A. M.; Mayer, L. W.; Morey, R.; Steigerwalt, A.; Boras, A.; Weylant, R. S. and Popovie. 2002. Sequencing of 16S rRNA gene: A rapid tool for the identification of Bacillus anthracis. Emerging Infectious Disease. 8(10):1117-1122. Doi: 10.3201/eid0810.020391. [ Links ]
Sajitha, K. L. and Florence, E. J. M. 2013. Effects of Streptomyces sp. on growth of Rubbergood Sapstain fungus Lasiodiplodia theobromae. Journal of Tropical Forest Science. 25(3):393-399. [ Links ]
Sánchez, O. M. R.; Sánchez, P. S. R.; Gallegos, M. G. y Sánchez, A. A. 2011. Actividad inhibitoria de actinomicetos aislados de hormigas cultivadoras de hongos (Hymenoptera:Formicidae) sobre Colletotrichum lindemuthianu, y Rhizoctonia solani. Revista Agraria. 8(1):14-17. [ Links ]
Sreevidya, M. and Gopalakrishnan, S. 2012. Bacteria and actinomycetes as biocontrol agents for the control of fungal of chickpea and sorghum. International Crops Research Institute for the Semi Arid Tropics. 293 p. [ Links ]
Tovar, P. J. M.; Mora, A. J. A.; Nava, D. C.; Téliz, O. D.; Villegas, M. A. and Leyva, M. S. G. 2013. Control of Lasiodiplodia theobromae, the causal agent of dieback of sapote mamey [Pouteria sapota (Jacq.) H. E. Moore and Stern] grafts in Mexico. Revista Fitotecnia Mexicana. 36(3):233-238. http://www.redalyc.org/articulo.oa?id=61028975007. [ Links ]
Ubalde, M. C.; Braña, V.; Sueiro, F. M.; Morel, A.; Martínez, R. C.; Marquez, C. and Castro, S. S. 2012. The iof Delftia sp. isolates as tools for bioremediation and biofertilization technologies. Current Microbiology. 64(6):597-603. [ Links ]
Úrbaez, T. J. R.; Peduto, F.; Vossen, P. M.; Krueger, W. H. and Gubler, W. D. 2013. Olive twig and branch dieback: etiology, incidence and distribution in California. Plant Disease . 97(12):23-244. http://dx.doi.org/10.1094/PDIS-04-12-0390-RE. [ Links ]
Wang, L.; Xing, M.; Di, R. and Luo, Y. 2015. Identification and antifungal activities of Streptomyces aureoverticillatus HN6. Journal of Plant Pathology and Microbiology. 6:281-286. http://dx.doi.org/10.4172/2157-7471.1000281. [ Links ]
White, T. J.; Bruns, T.; Lee, S. and Taylor, J. 1990. PCR Protocols: A guide to methods and applications. Academic Press. San Diego, CA. 322 p. [ Links ]
Received: February 01, 2020; Accepted: April 01, 2020