Servicios Personalizados
Revista
Articulo
Indicadores
-
Citado por SciELO
-
Accesos
Links relacionados
-
Similares en SciELO
Compartir
Revista mexicana de ciencias agrícolas
versión impresa ISSN 2007-0934
Rev. Mex. Cienc. Agríc vol.14 no.4 Texcoco may./jun. 2023 Epub 04-Ago-2023
https://doi.org/10.29312/remexca.v14i4.3200
Articles
Inhibitory activity of alcoholic extracts of ediblefungi against Rhizoctonia solani
1Posgrado en Ciencias Agropecuarias, Facultad de Ciencias Agrícolas- Universidad Veracruzana. Circuito Gonzalo Aguirre Beltrán s/n, 91000, Xalapa, Veracruz, México (mjnmescorp@gmail.com; gabrielauv@gmail.com).
2Instituto de Química Aplicada-Universidad Veracruzana. Luis Castelazo Ayala S/N, Col. Industrial Animas, 91190, Xalapa, Veracruz, México (remendoza@uv.mx).
3Colegio de Postgraduados-Campus Montecillo. Carretera México-Texcoco km 36.5, 56230, Montecillo, Estado de México, México (jalmaraz@hotmail.com).
Fungal diseases represent one of the main causes of crop losses annually. Rhizoctonia solani is a pathogenic fungus of worldwide distribution associated with root and tuber diseases of different crops; it causes important economic losses in perennial and annual plants, including almost all horticultural crops. To try to mitigate the losses caused by the fungus, fungicides of synthetic origin have been used; nevertheless, many of these substances are associated with carcinogenicity and are highly toxic to the environment. Edible macromycete fungi are a potential source of antifungal compounds that could be used to control fungal diseases in crops of agricultural interest. This work evaluated the antifungal activity of extracts (alcoholic and dilutions) of edible fungi (Lactarius deliciosus, Ustilago maydis, Amanita jacksonii and Amanita rubescens) against the phytopathogen R. solani by means of three techniques: well and disc diffusion and plate dilution. The results show that with the well diffusion technique, no effect on the growth of R. solani was observed with the alcoholic extracts and their dilutions. While with the disc diffusion technique, a slower growth was observed qualitatively compared to the controls of R. solani with the ethanolic extract of A. jacksonii and in its 1:1 methanol-water dilution. In the plate dilution technique, it was found that the 1:1 ethanol-water dilution of L. deliciosus had 88% inhibition on the growth of R. solani, followed by the ethanol extract of L. deliciosus (65%), methanol extract of U. maydis (63%) and methanol extract of L. deliciosus (57%). Therefore, extracts and dilution should be studied further, by being tested in an in vivo system and identifying the secondary metabolites present.
Keywords: Lactarius; Ustilago; phytopathogen
Las enfermedades fúngicas representan una de las principales causas de pérdidas en cultivos anualmente. Rhizoctonia solani es un hongo patógeno de distribución mundial asociado a enfermedades de raíces y tubérculos de diferentes cultivos; ocasiona pérdidas económicas importantes en plantas perennes y anuales, incluyendo casi todos los cultivos hortícolas. Para tratar de mitigar las pérdidas ocasionadas por hongo se ha hecho uso de fungicidas de origen sintético; sin embargo, muchas de estas sustancias están asociadas a carcinogenicidad y son altamente tóxicas para el medio ambiente. Los hongos macromicetos comestibles son una fuente potencial de compuestos antifúngicos que podrían usarse para controlar enfermedades fúngicas en cultivos de interés agrícola. Este trabajo evaluó la actividad antifúngica de extractos (alcohólicos y diluciones) de hongos comestibles (Lactarius deliciosus, Ustilago maydis, Amanita jacksonii y Amanita rubescens) contra el fitopatógeno R. solani por medio de tres técnicas: difusión de pozos y discos y dilución en placa. Los resultados muestran que con la técnica de difusión de pozos no se observó ningún efecto sobre el crecimiento de R. solani con los extractos alcohólicos y sus diluciones. Mientras que con la técnica de difusión de discos se observó un crecimiento más lento de manera cualitativa en comparación con los controles de R. solani con el extracto etanólico de A. jacksonii y en su dilución 1:1 de metanol-agua. En la técnica de dilución en placa se encontró que la dilución etanol-agua 1:1 de L. deliciosus tuvo 88% de inhibición sobre el crecimiento de R. solani, seguido del extracto de etanol de L. deliciosus (65%), extracto de metanol de U. maydis (63%) y extracto de metanol de L. deliciosus (57%). Por lo cual, los extractos y la dilución se deben estudiar a mayor profundidad, al ser probados en un sistema in vivo e identificar los metabolitos secundarios presentes.
Palabras clave: Lactarius; Ustilago; fitopatógeno
Introduction
Fungal diseases represent one of the main causes of crop losses annually, causing 60% of them during production and postharvest (Sommer et al., 2002). Rhizoctonia solani is a worldwide pathogenic causative agent associated with root and tuber diseases of different crops that causes important economic losses. The families and crops where this phytopathogen has been found are mentioned below. Poaceae or Gramineae: rice, wheat, barley, oats; Fabaceae: soybeans, peanuts, dried beans, alfalfa, chickpeas, lentils, peas; Solanaceae: tobacco and potato; Amaranthaceae: sugar beet; Brassicaceae: rapeseeds; Rubiaceae: coffee; Malvaceae: cotton and Asteraceae: lettuce (Ajayi and Bradley, 2018).
This necrotrophic pathogen (requires first killing the cells of its host and then taking the nutrients) is one of the most destructive found in the soil (Khaledi et al., 2015), among the diseases they commonly cause is the so-called damping-off of seedlings and root rot (Arcos and Zúñiga, 2015). The symptoms caused by the pathological complex of R. solani are observed in the underground and aerial parts of plants in two phases: 1) stem canker, which is the infection of growing plants; and 2) black crust or tuber infection with the formation of sclerotia. The ‘black crust’ is the most obvious sign of disease caused by R. solani and is characterized by black sclerotia of irregular sizes in the tuber, which, in a state of severity, can deform and crack it (Hide et al., 1973; Frank and Leach, 1980; Tsror, 2010).
To counteract the effects of this phytopathogen, fungicides belonging to different chemical groups with different active ingredients have been used. Four groups have been mainly reported: 1) quinone outside inhibitors (QoI) and active ingredients such as azoxystrobin, pyraclostrobin and trifloxystrobin; 2) succinate dehydrogenase inhibitors (SDHI) and active ingredients such as carboxin, penflufen and sedaxane; 3) demethylation inhibitors (DMI) and active ingredients such as penconazole and prothioconazole; and 4) inhibitors of phenylpyrroles (PP) with the active ingredient fludioxonil (Ajayi and Bradley, 2018).
Nevertheless, the Fungicide Resistance Action Committee (FRAC) stated that the risk of developing resistance to fungicides is high since they act at a single site within a metabolic pathway of the phytopathogen, so the dependence on these chemical fungicides for its control increases the risk that these organisms are resistant to these substances (FRAC, 2017). Although the use of broad-spectrum fungicides helps in the control of diseases generated by R. solani, these substances are highly toxic and have caused serious environmental and health problems (associated with carcinogenicity) (Patiño et al., 2020).
The strategies for the control of this phytopathogen are limited because of the high survival rates due to the formation of sclerotia, other natural and less toxic alternatives have been sought. Such is the case of plant extracts with antifungal activity, an example is the work of Al-Askar and Rashad (2010), who test hydroalcoholic extracts of four medicinal plants: cinnamon (Cinnamomum verum Presl.), anise (Pimpinella anisum L.), black cumin (Nigella sativa L.) and cloves (Syzygium aromaticum L. Merr. and Perry) against R. Solani in peas (Pisum sativum L.).
They found that clove extract at 1% completely inhibited radial fungal growth; while for the other hydroalcoholic extracts (4%) of plants, an inhibition of 90.5 to 100% was observed. The authors mention that the antifungal effect of the hydroalcoholic clove extract can be attributed to eugenol, the main active compound of the essential oil of this plant, which can form bonds in the active sites of the enzymes by deactivating them. In other research, Rafiq et al. (2021) evaluated the antifungal potential of methanolic extracts of leaves of safflower (Carthamus oxycantha) against R. solani, finding 27 to 37% inhibition in the growth of the phytopathogenic fungus. The observed effects were attributed to 95 compounds found in methanolic extracts, among which a derivative of D-ribofuranose, phthalate and, to a lesser extent, some fatty acids stand out.
While in the case of macromycete fungi, it has been reported (Patiño et al., 2020) that these organisms possess a potential source of antifungal compounds that could be used to control pathogens in plants and humans. Such is the case of metabolites from ethanolic extracts of Amanita rubescens, which showed antibacterial activity and antioxidant activity against Candida albicans, Streptococcus agalactiae and Staphylococcus aureus (Martínez et al., 2021). Another example is glycolipids (biosurfactants) obtained from Ustilago maydis, which have shown antimicrobial activity against Salmonella enterica and S. aureus (Cortes et al., 2011).
In the case of methanolic extracts of Lactarius sp., their antitumor and antimicrobial activity against S. aureus has been reported, attributed to metabolites such as sesquiterpenes, steroids, nitrogenous compounds and other secondary metabolites (Feussi et al., 2017). Doğan and Akbaş (2013) report the antimicrobial activity of chloroform, acetone and methanol extracts of Amanita caesarea against four gram-positive bacteria (Bacillus subtilis, Staphylococcus aureus, Listeria monocytogenes and Streptococcus pyogenes), five gram-negative bacteria (Escherichia coli, Klebsiella pneumoniae, Pseudomonas aeruginosa, Proteus vulgaris and Salmonella enteritidis) and a yeast (Candida albicans) by the microdilution method, finding a high inhibitory effect for C. albicans at a concentration of 4.8 μg mL of acetone extract, following the scale of Craig (1998).
In addition, Doğan and Akbaş (2013) report that the methanolic extract presented the maximum antimicrobial effect against Staphylococcus pyogenes and E. coli (MIC values, 78 and 156 mg mL, according to Craig’s scale), attributing this effect to diterpenes, triterpenes and polysaccharides. The most used solvents to perform the extraction of metabolites in fungi are water, ethanol, methanol, acetone and ethyl acetate (Elbatrawy et al., 2015), which was a point to consider when making the extracts; in the same way it was sought that the chosen solvents were easily accessible and handled by producers in the agricultural sector, in order to seek their applicability in the future.
It is worth mentioning that reports on the use of macromycete fungi extracts for the control of phytopathogens are scarce (Patiño et al., 2020; Volcão et al., 2021). Reinoro et al. (2013) proved that extracts of macromycete fungi native to Chile, by means of the disc diffusion technique, have active biological activity against phytopathogenic fungi.
The extract of Mycena hyalinotricha showed greater antifungal activity in the growth of Penicillium notatum, Ceratocistys pilifera and Rhizoctonia solani as it formed inhibition halos. The authors concluded that edible fungi extracts are good sources of antifungal active compounds and suggest the need to further investigate the potential of these metabolites in the agroforestry industry as a control of phytopathogens. Another study that explores the effect of active metabolites of edible fungi against phytopathogens is reported by Priya et al. (2019), who studied eight species of fungi, of which Lentinus edodes or shiitake fungus, Schizophyllum commune, Coprinus comatus and Volvariella volvaceae are edible, against the growth of the phytopathogenic fungus Colletotrichum capsici.
They found that L. edodes showed the maximum antifungal activity by inhibiting the mycelial growth of C. capsici by 45.55% with a maximum inhibition zone of 4.86 mm. The identification and study of bioactive compounds secreted by edible macromycetes are necessary in order to develop broad-spectrum fungicides against plant pathogens. Therefore, the present research aimed to test the antifungal effect of alcoholic extracts (methanol and ethanol) of four edible fungi (Lactarius deliciosus, Ustilago maydis, Amanita jacksonii and Amanita rubescens) against the phytopathogen R. solani through three methodologies.
Materials and methods
Obtaining edible fungi
The edible fungi were obtained in the local markets of the city of Xalapa, Veracruz in the period of July-August 2021. Subsequently, the specimens were taken to the Laboratory of Microbial Biotechnology of the Institute of Applied Chemistry to be cleaned and cut.
Obtaining hydroalcoholic extracts
Three hundred thirty grams of the fungal material of each respective fungus were weighed, then cut into fragments of approximately 4 cm x 4 cm, then the fragments were placed in an airtight glass bottle of 3 L capacity. Six containers with fungal material were established, 350 mL of methanol were added to three of them and 350 mL of ethanol were added to the other three containers. The containers with fungal material and solvents were left to macerate for five days at room temperature and after this time, they were filtered by gravity with filter paper of medium filtration, 8 μm pore, then the supernatant obtained from each respective fungus and solvent was placed in an Erlenmeyer flask of 500 mL.
The liquid obtained in each treatment was evaporated in a rotary vapor to obtain the concentrated extract, which regularly presents pasty-oily consistency. The three methanolic extracts and the three ethanolic extracts were established as stock solutions and from these, the hydroalcoholic extracts (treatments) were prepared with the following proportions: ethanol-water 1:1, methanol-water 1:1, ethanol-water 3:7 and methanol-water 3:7 (all in triplicate).
Antifungal activity of alcoholic extracts
Disc diffusion
Petri dishes were prepared with potato dextrose agar (PDA) medium, then filter paper discs (medium filtration, 8 μm pore) with 0.5 mm diameter were cut and sterilized in autoclave at 120 lb pressure for 20 min. Subsequently, under aseptic conditions, the filter paper discs (four discs for each alcoholic extract) were impregnated with 150 μL of each alcoholic extract and left to stand until the disc completely absorbed the extract. Then the Petri dishes with PDA were inoculated with a disc of the fungus R. solani, which was placed in the center of the dish with culture medium.
Four discs impregnated with each extract were placed equidistant to the disc with mycelium of R. solani. R. solani was isolated from the rhizosphere of poblano pepper (Capsicum annuum L.) and is part of the strain collection of the Department of Phytopathology of the Colegio de Postgraduados Montecillo Campus, State of Mexico. Treatments included a control of sterile distilled water, a methanol control and an ethanol control. The dishes were incubated at 28 °C for three days, after the incubation time it was observed if there was inhibition on the growth of R. solani and the diameter of inhibition around the disc impregnated with the extract was measured in millimeters with a ruler, all treatments were carried out in triplicate.
Well diffusion
Petri dishes were prepared with PDA medium, and three holes were made in the agar with the help of a punch of 0.5 mm in diameter. One hundred microliters of each alcoholic extract (ethanol-water 1:1, methanol-water 1:1, ethanol-water 3:7 and methanol-water 3:7) were added to each well, then a disc of R. solani with fresh mycelium was placed in the center of each dish (Cruz et al., 2010). Treatments included a control of sterile distilled water, a methanol control and an ethanol control (all in triplicate). Subsequently, the dishes were incubated at 28 °C for three days, after the incubation time it was observed if there was inhibition on the growth of R. solani close to the well, to measure the inhibition halo, it was measured in millimeters.
Plate dilution
Determination of the concentration of a solution of R. solani mycelium fragments
One hundred milliliters of potato dextrose broth were prepared, then the culture medium was inoculated with a 7 mm diameter disc of fresh mycelium of R. solani. It was incubated for four days at 28 °C, after this time the fungal solution was filtered through a sterile filter system (plastic column packed with cotton), to allow the passage of the smallest fragments of mycelium. The concentration of the mycelium fragments of the filtered liquid was estimated using a Neubauer chamber.
Once the concentration of the solution of fungal mycelium fragments (2 x 107 mycelium fragments / mL, 1X104 CFU) was known, the antifungal activity was determined by the plate microdilution method using the method described by Casaril et al. (2011); Ren et al. (2014) with modifications. In each well of a 96-well plate, the following were added: 100 μL of potato dextrose broth, 50 μL of the respective alcoholic extract of edible fungi (ethanol-water 1:1, methanol-water 1:1, ethanol-water 3:7 and methanol-water 3:7) and 50 μL of the solution of mycelium fragments of R. solani. Treatments included a control of sterile distilled water, a methanol control, an ethanol control, a control of potato dextrose broth, and a control of potato dextrose, all with solution of R. solani mycelium fragments (all in triplicate).
Subsequently, the dishes were incubated at room temperature in a horizontal stirrer at 400 rpm for four days, after this time the absorbance was read at 595 nm with a microplate reader. Antifungal activity was measured by comparing the optical densities of wells containing the same extracts and controls. The percentage of fungal growth inhibition was calculated using the following equation:
Where: AF is the absorbance of the fungal control without the addition of the extract; AFE is the absorbance of the mixture (alcoholic extract and suspension of mycelium fragments), and AE is the absorbance of the corresponding extract.
Statistical analysis
For the plate dilution technique, the bioassay was established under a completely randomized experimental design with three repetitions. The data obtained were performed an analysis of variance Anova and the mean comparison test (Tukey, α= 0.05) with the statistical program SAS Institute, 2020.
Results and discussion
Antifungal activity of hydroalcoholic extracts by well diffusion, disc diffusion and plate dilution techniques
Disc diffusion
In the results obtained with the disc diffusion method, it was observed that the fungus in some treatments such as ethanolic extract of A. jacksonii in its 1:1 methanol-water dilution, but also the extract of A. rubescens in the 3:7 ethanol-water dilution and 1:1 methanol-water dilution; extract of U. maydis 3:7 methanol-water dilution and of L. Deliciosus 3:7 ethanol-water dilution; grew slower and less abundantly compared to the controls (Figure 1).
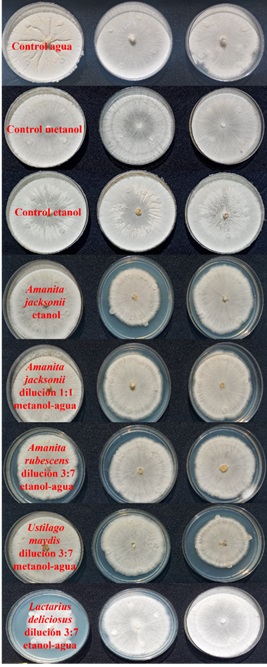
Figure 1 Alcoholic extract and dilutions of Amanita jacksonii against Rhizoctonia solani at 28 °C for three days of incubation.
It was qualitatively observed that R. solani grew more slowly in the extract of A. rubescens in the 3:7 ethanol-water dilution, where the fungus did not grow homogeneously and in the entire surface of the PDA as in the case of the controls. While the metabolic profile of each fungus is unique (Shen et al., 2017), the observation of the impact on growth and development of R. solani could be due to metabolites reported in alcoholic extracts of edible fungi, such as low molecular weight phenolic compounds that have antimicrobial activity (Alves et al., 2013).
Another possibility is that a minimal percentage of polar metabolites of phenolic nature were released in the alcoholic extracts of some macromycetes and could diffuse on the agar showing a slower growth in R. solani with respect to the controls (Naczk and Shahidi, 2006). In addition, disc diffusion depends directly on the diffusion capacity of the active substances present in the extracts, which can cause low mobility of metabolites in the agar if they have low solubility or high molecular mass.
Well diffusion
No effect on R. solani growth was observed with the alcoholic extracts and their dilutions, Figure 2, as R. solani grew in and out of the wells with extracts. These results could be related to the molecular weight of the compounds present in the extract, since if the molecular weight of the compounds is very high or if they have low solubility, their mobility through the agar will be difficult (non-diffusible), this could be a reason why no inhibitory effect from the alcoholic extracts and their dilutions was observed (Ren et al., 2014; Martínez et al., 2021).
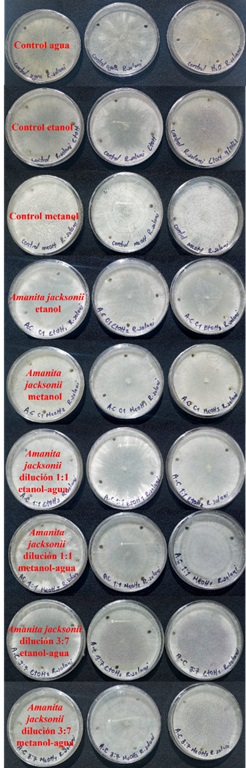
Figure 2 Dilutions of alcoholic extracts of Lactarius deliciosus, Ustilago maydis, Amanita jacksonii and Amanita rubescens against Rhizoctonia solani at 28 °C for three days of incubation.
Although it is not known what type of metabolites are present in the extracts of the edible fungi tested, it should be noted that the fungal material was used fresh and due to the type of solvents used (methanol, ethanol and water), it was sought to drag, in the extracts, polar metabolites such as polyphenols, sugars, alkaloids, saponins, glycosides and some proteins from macromycete fungi (Naczk and Shahidi, 2006; Akpi et al., 2017). The presence or absence of these metabolites in the extracts will depend on the environment in which the fungus develops and the different sources of nutrients available during its growth (Shen et al., 2017).
There may also be the possibility that the polar compounds present in the alcoholic extracts of edible fungi did not have inhibitory activity against R. solani in the well diffusion technique. On the other hand, it has been reported that, for the genus Amanita, peptides are the main constituents of polar extracts, these metabolites have greater antimicrobial activity in liquid medium or broth than in solid media such as agar, because they have low solubility (Li and Oberlies, 2005).
Plate dilution
The results indicate that the greatest inhibitory activity was shown by the 1:1 ethanol-water dilution of L. deliciosus (88%), while the lowest inhibitory activity was presented by the ethanolic extract of A. rubescens (0.5%), Figure 3. These results differ from those found in well and disc diffusion techniques. This behavior can be explained by what is reported in the literature (Ren et al., 2014; Balouiri et al., 2016), as they mention that, in the plate dilution technique, the metabolites present in the extracts tend to diffuse better in liquid media than in solid media, such is the case of the potato dextrose broth that was used in this technique.
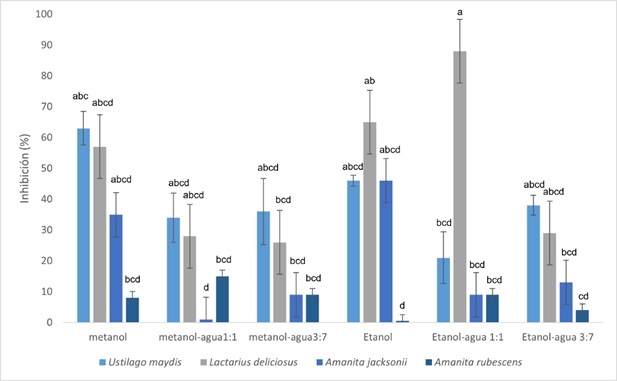
Figure 3 Alcoholic extracts and dilutions of edible fungi with inhibitory activity on the growth of Rhizoctonia solani. Mean ± standard error, n= 3.
One of the advantages of using this technique is its ability to identify antimicrobial activity and quantify it accurately, since the results are not affected by the growth rate of different microorganisms (Morales et al., 2013). This is a possible reason that there are stronger inhibitions with greater effectiveness. The inhibitory activity shown by the ethanolic extract and the 1:1 ethanol-water dilution of L. deliciosus in the growth of R. solani can be attributed to the presence of phenolic compounds contained in the ethanolic extract of this edible fungus (Zavastin et al., 2015).
L. deliciosus has proven antifungal activity as reported by Volcão et al. (2021), who evaluated the antifungal activity of the hydroalcoholic extract obtained from L. deliciosus against the phytopathogen Monilinia fructicola (Wint.), they report that the extract inhibited fungal growth and reduced mycelial development of the phytopathogen at a concentration of 1.25 mg L-1. On the other hand, the processing of the fungal material (fresh or dried), the extraction time and the type of solvent should also be considered, which will influence the antimicrobial activity of the extracts (Adhikari et al., 2018).
Among the most used solvents to perform the extraction of metabolites in fungi are water, ethanol, methanol, acetone and ethyl acetate (Elbatrawy et al., 2015). In the results found in this study, a 1:1 ethanol-water dilution of L. deliciosus was the one that presented greater antifungal activity, even greater than those extracts that did not have any dilution and were only the solvent, a possible explanation for this behavior is reported by Yim et al. (2012), who point out that the best solvent for the extraction of phenolic compounds from fungi is water since it does not break glycosidic bonds, which indicates the presence of free phenols. In the 1:1 dilution, the presence of water favored the extraction of free phenols and therefore it was possible to quantify the highest antifungal activity of this extract.
Conclusions
Regarding disc diffusion, in some treatments it was observed that the fungus did not grow homogeneously as in the controls, which qualitatively suggests slow growth of R. solani; however, more evidence and studies are needed to affirm that with this methodology there is some quantifiable inhibitory effect. While in the disc diffusion method, no inhibition of the growth of R. solani was found. Nevertheless, the results obtained by the plate dilution technique are considered reliable, since the alcoholic extracts (and dilutions) had a direct contact in liquid medium with R. solani in the microplate wells.
Therefore, the 1:1 ethanol-water dilution of L. deliciosus, ethanol extract of L. deliciosus, methanol extract of U. maydis and methanol extract of L. deliciosus should be studied in greater depth, by testing them in an in vivo system and identifying the metabolites present.
Bibliografía
Adhikari, P.; Pandey, A.; Agnihotri, V. and Pande, V. 2018. Selection of solvent and extraction method for determination of antimicrobial potential of Taxus wallichiana Zucc. Res Pharmacy.8 (1):1-9. Doi: 10.25081/rip.2018.v8.3487. [ Links ]
Ajayi-Oyetunde, O. O.; and Bradley, C. A. 2018. Rhizoctonia solani: taxonomy, population biology and management of Rhizoctonia seedling disease of soybean. Plant Pathol. 67(1):3-17. https://doi.org/10.1111/ppa.12733. [ Links ]
Akpi, U. K.; Odoh, C. K.; Ideh, E. E. and Adobu, U. S. 2017. Antimicrobial activity of Lycoperdon perlatum whole fruit body on common pathogenic bacteria and fungi. Afr. J. Clinical Exp. Microbiol. 18(2):79-85. Doi: 10.4314/ajcem.v18i2.4. [ Links ]
Al-Askar, A. A.; and Rashad, Y. M. 2010. Efficacy of some plant extracts against Rhizoctonia solani on pea. J. Plant Protec. Res. 50(3):239-243. Doi: 10.2478/v10045-010-0042-0. [ Links ]
Alves, M. J.; Ferreira, I. C.; Froufe, H. J.; Abreu, R. M. V.; Martins, A. and Pintado, M. 2013. Antimicrobial activity of phenolic compounds identified in wild mushrooms, SAR analysis and docking studies. J. Appl. Microbiol. 115(2):346-357. https://doi.org/10.1111/jam.12196 [ Links ]
Arcos, J. y Zúñiga, D. 2015. Efecto de rizobacterias en el control de Rhizoctonia solani en el cultivo de papa. Ecología Aplicada. 14(2):95-101. [ Links ]
Balouiri, M.; Sadiki, M. and Ibnsouda, S. K. 2016. Methods for in vitro evaluating antimicrobial activity: a review. J. Pharmaceutical Analysis. 6(2):71-79. [ Links ]
Casaril, K. B. P. B.; Kasuya, M. C. M. and Vanetti, M. C. D. 2011. Antimicrobial activity and mineral composition of shiitake mushrooms cultivated on agricultural waste. Braz. Archiv. Biol. Technol. 54 (5):991-1002. [ Links ]
Cortes, S. A.; Hernández S. H. and Jaramillo, F. M. 2011. Production of glycolipids with antimicrobial activity by Ustilago maydis FBD12 in submerged culture. Afr. J. Microbiol. Res. 5(17):2512-2523. Doi: 10.5897/AJMR10.814. [ Links ]
Craig, W. A. 1998. Pharmacokinetic/pharmacodynamic parameters: rationale for antibacterial dosing of mice and men. Clinical Infec. Dis. 26(1):1-10. [ Links ]
Cruz, C. A.; Rodríguez, N. N. and Rodríguez, C. E. 2010. Evaluación in vitro del efecto antibacteriano de los extractos de Bidens pilosa, Lantana camara, Schinus molle y Silybum marianum. Rev. UDCA Actualidad & Divulgación Científica. 13(2):117-124. [ Links ]
Doğan, H. H. and Akbaş, G. 2013. Biological activity and fatty acid composition of Caesar’s mushroom. Pharmaceutical Biol. 51(7):863-871. https://doi.org/10.3109/13880209.2013.768272. [ Links ]
Elbatrawy, E. N.; Ghonimy, E. A.; Alassar, M. M. and Wu, F. S. 2015. Medicinal mushroom extracts possess differential antioxidant activity and cytotoxicity to cancer cells. Inter. J. Med. Mushrooms. 17(5): 471-479. [ Links ]
Feussi, T. M.; Qin, J.; Ndongo, J. T. and Laatsch, H. 2017. New azulene-type sesquiterpenoids from the fruiting bodies of Lactarius deliciosus. Natural products and bioprospecting. 7(3):269-273. https://doi.org/10.1007/s13659-017-0130-1. [ Links ]
FRAC. 2017. Fungicide Resistance Action Committee. Fungicides sorted by mode of action including FRAC code numbering. http://www.frac.info/docs/default-source/publications/fraccode-list/frac-code-list-2017-final.pdf?sfvrsn=2. [ Links ]
Frank. J. A. and Leach S. S. 1980. Comparison of tuber-borne and soilborne inoculum in the Rhizoctonia disease of potato. Phytopathology. 70(1):51-53. [ Links ]
Hide, G. A.; Hirst, J. M. and Stedman, O. J. 1973. Effects of black scurf (Rhizoctonia solani) on potatoes. Annal. Appl. Biol. 74(2):139-148. https://doi.org/10.1111/j.1744-7348.1973.tb07733.x. [ Links ]
Khaledi, N.; Taheri, P. and Tarighi, S. 2015. Antifungal activity of various essential oils against Rhizoctonia solani and macrophomina phaseolina as major bean pathogens. J. Appl. Microbiol. 118(3):704-717. https://doi.org/10.1111/jam.12730. [ Links ]
Li, C.; and Oberlies, N. H. 2005. The most widely recognized mushroom: chemistry of the genus Amanita. Life Sci. 78(5):532-538. https://doi.org/10.1016/j.lfs.2005.09.003. [ Links ]
Martínez, E. N. A.; Vázquez, G. F. J.; Valero, G. J.; Álvarez, P. E.; Garza, O. F.; Najera, M. J. A. and Quiñónez, M. M. 2021. Antimicrobial activity, phenolic compounds content, and antioxidant capacity of four edible macromycete fungi from Chihuahua, México. TIP. Rev. Especial. Cienc. Quím. Biológ. 24:1-11 https://doi.org/10.22201/fesz.23958723e.2021.318. [ Links ]
Naczk, M.; and Shahidi, F. 2006. Phenolics in cereals, fruits and vegetables: occurrence, extraction and analysis. J. Pharmaceutical Bio. Analys. 41(5):1523-1542. https://doi.org/10.1016/j.jpba.2006.04.002. [ Links ]
Patiño, M.; Nieto, R. I. J.; Chegwin, A. C. and Torres, R. E. 2020. Actividad biocontroladora in vitro de macrohongos contra diferentes hongos fitopatógenos. Acta Biol. Colomb. 25(2):265-279. https://doi.org/10.15446/abc.v25n2.75303 [ Links ]
Priya, K.; Thiribhuvanamala, G.; Kamalakannan, A. and Krishnamoorthy, A. S. 2019. Antimicrobial activity of biomolecules from mushroom fungi against Colletotrichum capsici (Syd) Butler and bisby, the fruit rot pathogen of Chilli. Inter. J. Current Microbiol. Appl. Sci. 8(6):1172-1186. [ Links ]
Rafiq, M.; Javaid, A. y Shoaib, A. 2021. Antifungal activity of methanolic leaf extract of Carthamus oxycantha against Rhizoctonia solani. Pak. J. Bot. 53(3):1133-1139. http://dx.doi.org/10.30848/PJB2021-3(17). [ Links ]
Reinoso, R.; Cajas, M. D.; Martinez, M.; Martin, A.; Perez, C.; Fajardo, V. and Becerra, J. 2013. Biological activity of macromycetes isolated from Chilean subantarctic ecosystems. J. Chilean Chem. Soc. 58(4):2016-2019. [ Links ]
Ren, L.; Hemar, Y.; Perera, C. O.; Lewis, G.; Krissansen, G. W. and Buchanan, P. K. 2014. Antibacterial and antioxidant activities of aqueous extracts of eight edible mushrooms. Bioactive Carbohydrates and Dietary Fibre. 3(2):41-51. https://doi.org/10.1016/j.bcdf.2014.01.003. [ Links ]
SAS. 2020. Institute Inc. The System for Windows, version 9.4; SAS Institute Inc.: Cary, NC, USA. [ Links ]
Shen, H. S.; Shao, S.; Chen, J. C. and Zhou, T. 2017. Antimicrobials from mushrooms for assuring food safety. Comprehensive reviews in Food Science and Food Safety. 16(2):316-329. https://doi.org/10.1111/1541-4337.12255. [ Links ]
Sommer, N. F.; Fortalage, J. R.; Edwuards, D. C. 2002. Postharvest Diseases of selected commodities. In: Kader, A. A. Ed. Postharvest technology of horticultural crops. University of California. 223-227 pp. [ Links ]
Tsror, L. 2010. Biology, epidemiology and management of Rhizoctonia solani on potato. J. Phytopathol. 158(10):649-658. https://doi.org/10.1111/j.1439-0434.2010.01671.x. [ Links ]
Volcão, L. M.; Halicki, P. C. B.; Christ, R. A.; Ramos, D. F.; Badiale, F. E.; Andreazza, R. and Silva J. F. 2021. Mushroom extract of Lactarius deliciosus (L.) Sf. Gray as biopesticide: antifungal activity and toxicological analysis. J. Toxicol. Environ Health. 85(2):43-55. [ Links ]
Yim, H. S.; Chye, F. Y.; Koo, S. M.; Matanjun, P.; How, S. E. and Ho, C. W. 2012. Optimization of extraction time and temperature for antioxidant activity of edible wild mushroom, Pleurotus porrigens. Food and Bioproducts Processing. 90(2): 235-242. [ Links ]
Zavastin, D. E.; Miron, A.; Gherman, S. P.; Boerescu, C. M.; Breaban, I. G. and Gavrilescu, C. M. 2015. Antioxidant activity, total phenolic and metals contents of Lactarius salmonicolor. Farmacia. 63(5):755-759. [ Links ]
Received: May 01, 2023; Accepted: June 01, 2023