Servicios Personalizados
Revista
Articulo
Indicadores
-
Citado por SciELO
-
Accesos
Links relacionados
-
Similares en SciELO
Compartir
Revista mexicana de ciencias agrícolas
versión impresa ISSN 2007-0934
Rev. Mex. Cienc. Agríc vol.15 no.3 Texcoco abr./may. 2024 Epub 25-Ago-2024
https://doi.org/10.29312/remexca.v15i3.3655
Articles
Antagonistic microorganisms as management of Fusarium oxysporum wilt of blackberry
1Facultad de Agrobiología ‘Presidente Juárez’-Universidad Michoacana de San Nicolás de Hidalgo. Paseo Lázaro Cárdenas esquina con Berlín, Uruapan, Michoacán, México. CP. 60040. (1153378b@umich.mx; atahualpa.guzman@umich.mx; antonio.garcia@umich.mx; spaleo@umich.mx).
2Facultad de Biología Universidad Michoacana de San Nicolás de Hidalgo. Avenida Francisco J. Múgica s/n, Ciudad Universitaria, edificio B-1, Morelia, Michoacán, México. CP. 5806. (margarita.vargas@umich.mx).
In the states of Michoacán, Mexico and California, USA, blackberry (Rubus sp.) production is affected by wilt, which causes the loss of thousands of hectares. In the present work, the causative agent of the disease was identified and control alternatives with antagonistic microorganisms were proposed. In 2022, the causative agent of the disease was isolated and morphologically identified from an orchard in Tocumbo, Michoacán. A preliminary laboratory bioassay was performed with strains of B. subtilis, B. amyloliquefaciens, P. fluorescens, and Trichoderma spp., native to Tacámbaro and Taretan, Mich. The outstanding strains were tested in a greenhouse on plants of blackberry cv Tupy as a preventive measure and as a control, under an experimental design of randomized blocks with six repetitions. In the preliminary tests, all the strains used showed an inhibition of the mycelial growth of the pathogen greater than 50%, with Trichoderma sp. from Tacámbaro, B. subtilis and P. fluorescens standing out. In the tests carried out in situ, preventively applied treatments decreased the incidence of wilt by 57% to 66%. In terms of severity and plant height, Trichoderma sp. stood out, while the percentage of root necrosis decreased by 56% to 70% with all treatments. Control treatments also showed antagonistic efficacy, although to a lesser extent, they decreased the incidence of wilt by 2 to 58% and the percentages of root necrosis by 19 to 62%. Antagonistic microorganisms can be incorporated as a comprehensive management strategy with high efficiency.
Keywords: Rubus; Fusarium; biocontrol; comprehensive management; wilt
En los estados de Michoacán, México y California, EUA, la producción de zarzamora (Rubus sp.) se ve afectada por una marchitez que ocasiona la pérdida de miles de hectáreas. En el presente trabajo se identificó el agente causal de la enfermedad y propuso alternativas de control con microorganismos antagonistas. En el año 2022 de un huerto en Tocumbo, Michoacán, se aisló e identificó morfológicamente al agente causal de la enfermedad. Se realizó un bioensayo preliminar en laboratorio con cepas de B. subtilis, B. amyloliquefaciens, P. fluorescens y Trichoderma spp, nativas de Tacámbaro y Taretan, Mich. Las cepas sobresalientes se probaron en invernadero en plantas de zarzamora cv Tupy de forma preventiva y como control, bajo un diseño experimental de bloques al azar con seis repeticiones. En las pruebas preliminares todas las cepas utilizadas mostraron una inhibición del crecimiento micelial del patógeno superior al 50%, sobresaliendo Trichoderma sp. de Tacámbaro, B. subtilis y P. fluorescens. En las pruebas realizadas in situ los tratamientos aplicados de forma preventiva disminuyeron la incidencia de marchitez desde 57 hasta 66%. En términos de severidad y altura de la planta destacó Trichoderma sp., mientras que el porcentaje de necrosis radicular disminuyó desde 56 hasta 70% con todos los tratamientos. Los tratamientos de control también mostraron eficacia antagonista, aunque en menor medida, disminuyeron la incidencia de marchitez desde 2 al 58% y los porcentajes de necrosis radicular desde 19 a 62%. Los microorganismos antagonistas pueden incorporarse como una estrategia de manejo integral con gran eficiencia.
Palabras clave: Rubus; Fusarium; biocontrol; manejo integral; marchitez
Introduction
Mexico stands out as a global producer of berries, with blackberry (Rubus sp.) being the most prominent (Ricárdez-Luna et al., 2016). Michoacán contributes significantly 93.3% of blackberry production (SIAP, 2021). Although this fruit adapts well to temperate conditions, it suffers from production and marketing restrictions due to Fusarium oxysporum, which causes wilt in blackberry plantations in California and Mexico (Gordon et al., 2017).
A new lineage of F. oxysporum, recently identified, has emerged as responsible for the disease, becoming a devastating threat in Mexico, especially with severe epidemic expression in the Tupy variety in commercial plantations (Hernández-Cruz et al., 2020).
Due to the environmental and health risks posed by the extensive use of synthetic fungicides and fumigants (Guédez et al., 2009; Vásquez-Ramírez, 2017), it is imperative to reduce the use of chemical pesticides that affect the ecosystem (Villa et al., 2005). The application of antagonistic microorganisms emerges as an indispensable alternative, not only for phytopathological control but also to improve stress tolerance in plants (Guédez et al., 2008; Tian et al., 2018).
Given the urgency of approaches with lower environmental impact, this work aims to evaluate the efficacy of antagonistic microorganisms in the management of blackberry wilt disease.
Materials and methods
Collection, isolation, and identification
In 2022, in the municipality of Tocumbo, a targeted sampling of wilt symptomatic plants was carried out in an orchard of ‘Tupy’ blackberry (19° 38’ 21.5” north latitude and 102° 29’ 02.9” west longitude) at 1 300 masl. The second-year and conventionally managed plant was grown in the open field. Phytopathological techniques from Agrios (2005) and Trigiano et al. (2004) were followed. Symptomatic roots were sown in ½X potato dextrose agar (PDA) culture medium and incubated at room temperature in darkness, hyphae tips. Monosporic cultures were obtained in Spezieller Nührstoffarmer agar (SNA) culture medium and stored at -70 °C in sterile 25% glycerol.
Barnett and Hunter (1998); Leslie and Summerell (2006) keys were used for identification, comparing morphologically with fixed preparations. A cultural characterization was performed in 1XPDA medium and 20 μl of the conidia suspension was inoculated with 25% glycerol. Morphological and cultural characteristics were recorded, including mycelium type and thickness, colony color, agar pigmentation, sporodochia presence and color, presence of chlamydospores, and possible presence of rolled hyphae at 15, 30, and 60 days.
Additional morphological characterization was performed in carnation leaf agar (CLA) medium, 5 μl of the suspension was inoculated, and the length and width of 50 macroconidia and 50 microconidia of each isolate were measured. The characteristics determined were shape, foot type and apical cell in macroconidia and shape, arrangement, and type of phialide in microconidia, along with the presence and arrangement of chlamydospores and rolled hyphae at 10 days.
For growth rate, 5 μl of the suspension was inoculated in paperless SNA medium, incubating for 7 days at 25 °C in darkness. A 6 mm diameter disc was transferred to the center of a Petri dish with PDA medium, incubated for 72 h, and the growth (mm) rate was recorded. The analysis of variance was performed with the R program, version 3.5.1 (R Core Team, 2020) and the R Studio interface, version 1.1.463 (Rstudio Team, 2020), with six repetitions and two repetitions of the experiment.
Pathogenicity tests
According to Dhingra and Sinclair (1985), healthy blackberry seedlings from a nursery in Zirimícuaro, Michoacán, grown in granzón as a substrate, were inoculated with 100 ml in a 1 x 10⁶ conidia ml-1 suspension. The plants were evaluated every 15 days, observing from the first symptoms to the total wilting characteristic of the disease. Koch’s postulates were corroborated.
In vitro bioassay
In vitro confrontation tests were performed between antagonists (Trichoderma native to Tacámbaro and Taretan, Bacillus subtilis, Bacillus amyloliquefaciens and Pseudomonas fluorescens) and the pathogen using the quadrant confrontation method, in Petri dishes with 20 ml of PDA culture medium. The dish was divided into quadrants, placing discs (10 mm in diameter) of the pathogen and antagonist at opposite ends, the control treatment consisted only of the pathogen without the antagonist.
A completely randomized experimental design with six repetitions was used, measuring radial growth every 24 h. Measurements of the radial growth of the pathogen (RG mm) in interaction with the antagonists and of the isolated control (pathogen) determined the in vitro antagonistic effect. An analysis of variance of daily growth was performed for eight days, with Tukey’s mean separation (0.05). The analyses were performed using the R program, version 3.5.1 (R Core Team, 2020) and the R Studio interface, version 1.1.463 (Rstudio Team, 2020). Based on the results of the in vitro assay, the antagonists were confronted under greenhouse conditions to evaluate their in vivo efficiency in controlling the pathogen.
Greenhouse bioassay
In healthy four-month-old Tupy blackberry plants from a commercial nursery in Zirimícuaro, Michoacán, 100 ml of a solution of 1 x 10⁶ CFU ml-1 of Bacillus subtilis and Pseudomonas fluorescens, and 100 ml of a solution of 1 x 10⁶ conidia ml-1 of Trichoderma sp., a native to Tacámbaro, were applied as a preventive measure and as a control, individually and in combination, generating 14 treatments under a randomized block design. Each experimental unit consisted of 6 repetitions, the plants were placed in sterile granzón as a substrate.
Preventive treatments were applied two weeks prior to inoculating the plants with a conidia solution of 1 x 10⁶ conidia ml-1 of pathogen. In control treatments, inoculation of the pathogen preceded the antagonists by two weeks, using the same concentration of spores. Controls were established for each case.
The variables of incidence of yellowing and wilting of foliage were evaluated, determining the percentage of foliage with symptoms of the disease, and it was evaluated according to the following formula:
Disease severity was measured on a scale of 1 to 100% based on foliar damage. Plant height was compared to healthy plants. Weight, length, and necrosis of the root were recorded, establishing percentages of rot on a scale of 1 to 100%. The data were transformed with the arcsine function for analysis.
Statistical analyses of each variable for a completely randomized design and Tukey’s mean separation (0.05) were performed with the R program, version 3.5.1 (R Core Team, 2020) and the R Studio interface, version 1.1.463 (Rstudio Team, 2020).
Results and discussion
An isolate was obtained from the isolations carried out, which by comparison of its morphological characteristics belongs to the genus Fusarium.
Cultural characteristics
The colony in PDA had radial growth, abundant, cottony, floppy, aerial, entire-margin mycelium. White on the obverse and white to pink, light violet, and dark violet on the reverse as the colony aged (Figure 1).
Temperature, darkness, and culture medium are important factors for the formation of pigments in this genus. PDA medium, due to its carbohydrate content, allows ideal development and expression of the culture such as shape, color, pigmentation of the medium and growth (Leslie and Summerell, 2006).
Morphological characteristics
Elliptic microconidia in false heads, without septa, rarely with 1 septum, intercalated chlamydospores were observed in PDA medium. In SNA medium, uniform microconidia, in short monophialides, with aerial mycelium. The formation of macroconidia was only observed in the CLA culture medium, almost straight in shape, with three septa, curved only at the tips, thin, with a curved apical cell and basal cell in the shape of a foot with 3 septa (Figure 2).
Growth rate
The rate ranged from 28 to 30 mm at 72 h at 25 °C and although it is a secondary trait, traditionally in PDA medium and allowed to grow for three days at 25 or 30 °C, it allows to distinguish fast and slow growing species (Leslie and Summerell et al., 2006). The characteristics of the colony in the PDA, SNA and CLA culture media, as well as the growth rate, coincide with those reported by Leslie and Summerell (2006) for the species Fusarium oxysporum.
Pathogenicity tests
The symptoms appeared two months after inoculation, they began with yellowing and flaccidity in the leaves at the base of the plant, wilting the plant five months after inoculation. The roots showed necrosis and brown discoloration of the xylem tissues. Root re-isolation confirmed the presence of the inoculated Fusarium oxysporum. Uninoculated plants continued without the presence of symptoms (Figure 3).
In vitro bioassay
The results showed significant variation between treatments. From the fourth day, the effects of Trichoderma sp., Tacámbaro, Trichoderma sp., Taretan, and B. subtilis were statistically similar to and superior to B. amyloliquefaciens and P. fluorescens in inhibiting the radial growth (RG) of Fusarium. On the eighth day, P. fluorescens showed a 78% decrease in RG, while Trichoderma sp., Tacámbaro, Trichoderma sp., Taretan, and B. subtilis recorded a decrease of 71%, 68%, and 65%, respectively (Figures 4 and 5).
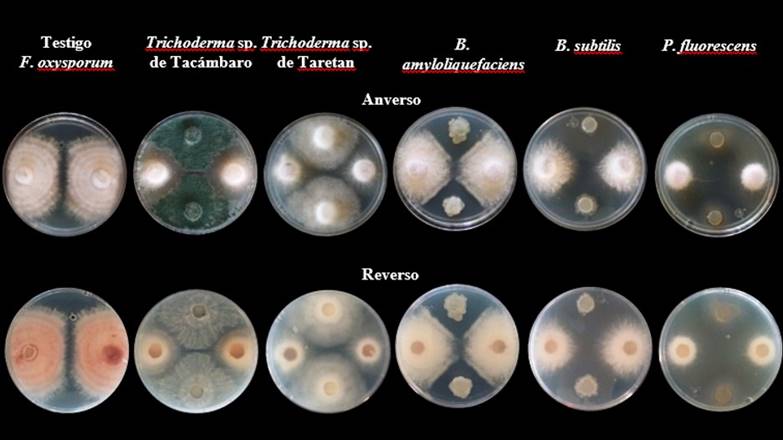
Figure 4 Obverse and reverse of the quadrant confrontation of Fusarium oxysporum with each of the antagonistic treatments on day eight.
Microorganisms such as P. fluorescens, B. subtilis and both Trichoderma species, which stand out for their efficacy in controlling Fusarium spp. and F. oxysporum in controlled environments, have been supported by previous studies (Guerra et al., 2011; Tejera-Hernández et al., 2011; Guédez et al., 2012; Mejía-Bautista et al., 2016; Boughalleb-M’Hamdi et al., 2018).
Biocontrol with Trichoderma is attributed to the production of enzymes with antifungal activity, affecting the mycelium of F. oxysporum by penetration and lysis (Martínez et al., 2013). Competition for space and nutrients also play a role (Infante et al., 2009). In the treatment with Trichoderma sp. from Tacámbaro, an initial parasitism of the pathogen was observed, corroborating previous findings with T. viride against P. infestans (Bouziane et al., 2016).
On the other hand, the antagonism of P. fluorescens and B. subtilis could be attributed to antibiosis, competition for resources, and induction of systemic resistance. In addition, the production of enzymes that degrade the cell wall and volatile organic compounds, phytoalexins and siderophores (Cornelis et al., 2008; Bhattacharyya and Jha, 2012; Singh and Singh, 2013).
Greenhouse bioassay
Yellowing and wilting in blackberry plants inoculated with F. oxysporum and with the antagonists showed significant variation. Preventive treatments with B. subtilis (37.65%), Trichoderma sp. (33.64%), P. fluorescens (42.32%) and the consortium of antagonistic agents (37.46), as well as P. fluorescens (41.97%) as a control, showed to be statistically equal, decreasing the incidence compared to the control inoculated with Fusarium, which had 100%; the preventive treatment with Trichoderma sp. reduced the incidence by 66.36% (Figure 6).
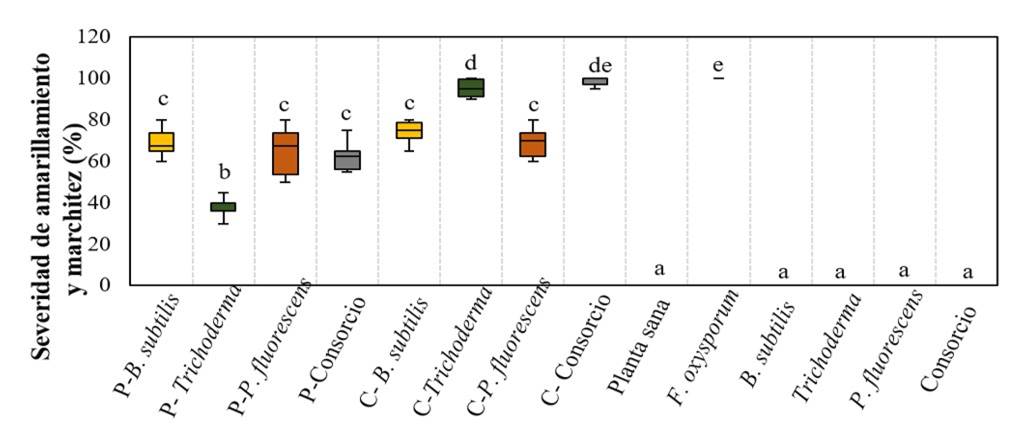
Figure 6 Comparison of means and percentage of severity of foliar symptoms in blackberry plants inoculated with F. oxysporum. P= preventive and C= control.
The control treatments with the consortium of antagonistic agents and with Trichoderma sp. behaved statistically the same as the control, with 98.97% and 96.69% yellowing and wilting. The treatment without inoculation and those inoculated with the antagonists B. subtilis, Trichoderma sp., P. fluorescens alone or in consortium did not present any incidence.
As a preventive measure, each antagonist decreased the percentage of incidence, agreeing with Acosta-González et al. (2018), who tested commercial and native biological control agents on the incidence of blackberry wilt due to Fusarium and reported that strains of Trichoderma, Pseudomonas fluorescens, and bacterial complexes showed positive results as they decreased the percentage of incidence. As a preventive measure, Trichoderma decreased the severity of yellowing by 61.73%, as reported by Rebollar and Silva (2019) under greenhouse conditions for blackberry wilt.
The high incidence of wilt in the antagonist consortium as a control could be attributed to the complex interrelationships between microorganisms at the soil-plant-microorganism-environment interface. Trichoderma and Pseudomonas often rely on these factors to manifest their beneficial effects. Nevertheless, in the interaction of these three types of microorganisms, synergistic effects may arise that amplify the benefits or conversely, that do not generate any appreciable effect (Cano, 2011).
The treatment inoculated with Trichoderma sp. and the preventive treatment, both statistically equal, exhibited the greatest increase in plant height, resembling previous findings by Ruiz-Cisneros et al. (2018) (Figure 7). Trichoderma generates secondary metabolites that influence plant growth and development (Contreras-Cornejo et al., 2016). In addition, it can activate genes related to plant defense, increasing the level of ergosterol, and promoting faster growth in the soil, thus explaining its positive effects on plant growth and defense (Mayo et al., 2015).
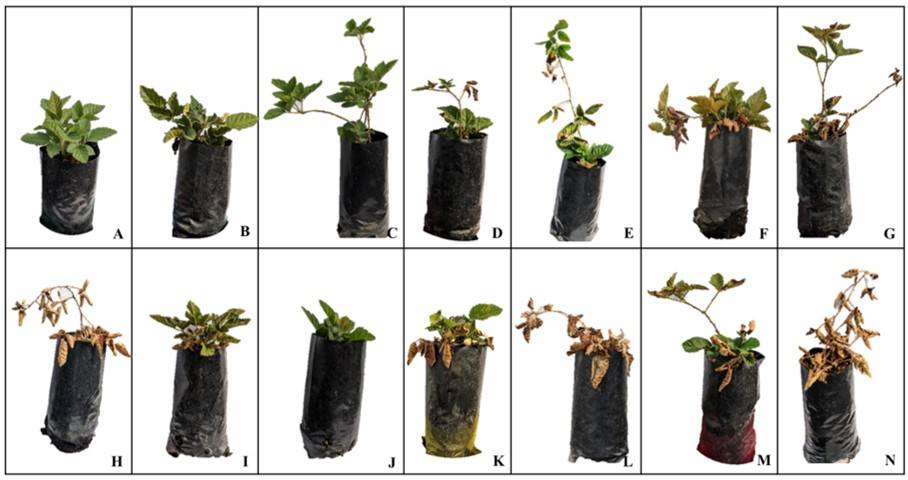
Figure 7 Appearance of blackberry plants at the end of the tests. A) healthy plant; B) control P-B. subtilis; C) control Trichoderma sp.; D) P-B. subtilis; E) P-Trichoderma sp.; F) P-P. fluorescens; G) P-consortium; H) control Fusarium oxysporum; I) control P. fluorescens; J) consortium control; K) C-B. subtilis; L) C-Trichoderma sp.; M) C-P. fluorescens; and N) C-consortium.
The roots of the blackberry plants of the treatment without inoculation and the controls inoculated with B. subtilis, Trichoderma sp., P. fluorescens and the consortium of antagonists showed a slight percentage of root necrosis, isolates of these were made and the presence of pathogens was ruled out. This necrosis could be caused by excess water in the substrate, oxygen becomes scarce, causing asphyxiation and subsequent root rot.
In the preventive or control treatments inoculated with Fusarium, B. subtilis and Trichoderma sp. were statistically the same, with mean percentage of necrosis of 29.84%, as in the case of the preventive treatment with P. fluorescens. In comparison, the control treatment with the antagonist consortium and the control exhibited similar statistics and the highest percentages of necrosis (Figure 8).
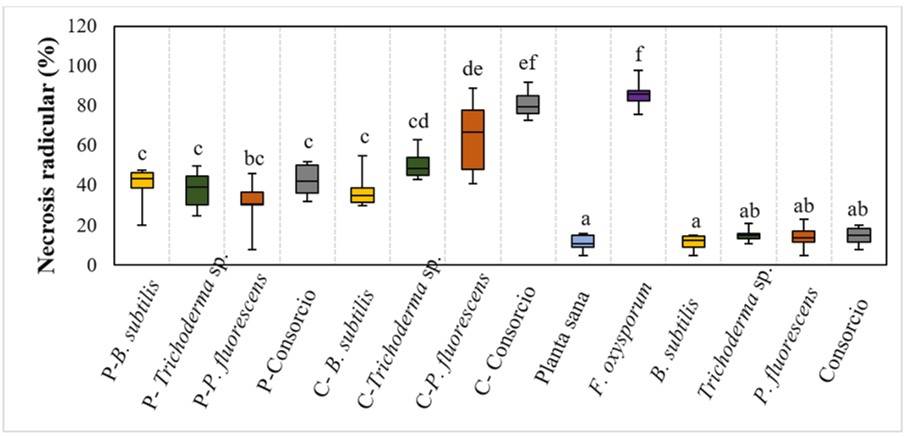
Figure 8 Comparison of means (Tukey, 0.05) of the percentage of necrosis present in the root of blackberry plants. P= preventive; C= control.
The efficiency in reducing the percentage of necrosis with the preventive treatment of P. fluorescens could be due to its ability to colonize the rhizosphere of plants and its antagonistic activity towards various pathogens (Perotti et al., 2005) as it is able to chelate iron, making it less available to pathogens or other microorganisms (Susan and Castiel, 2005). This coincides with Guerra et al. (2011), who reported efficient P. fluorescens strains for the control of F. oxysporum under controlled and greenhouse conditions.
Control and preventive treatments with Trichoderma sp. decreased the percentage of root necrosis since when colonizing the rhizosphere, any pathogen that crosses this protection is destroyed by the hyperparasitism it has, in addition to acting as a barrier to prevent the entry of pathogens into the roots (Gómez et al., 2013).
Conclusions
Fusarium oxysporum, the causative agent of yellowing and wilting in blackberry plants, was identified by its morphological and cultural characteristics. The strains of Trichoderma, Bacillus and P. fluorescens showed in vitro antagonistic capacity greater than 50% against F. oxysporum, P. fluorescens stood out with a remarkable 78% inhibition in radial growth.
In the greenhouse, preventive treatments exhibited remarkable antagonistic capacity, reducing the incidence of wilt by more than 57%. Trichoderma sp. stood out with a decrease of more than 66% and an increase in height of more than 50%. B. subtilis and P. fluorescens as controls also showed reductions of more than 45%.
In the preventive and control treatments, B. subtilis and Trichoderma sp. reduced root necrosis by more than 49%, the preventive treatment with Trichoderma sp. stood out, with a decrease of more than 62%. The preventive application of the antagonistic microorganisms showed greater efficacy in the prevention of blackberry yellowing and wilting compared to their application as a control.
Acknowledgements
We would like to thank Eng. Joel Alejandro Vargas Hernández and M. Sc. Erwin Saul Navarrete Saldaña for their help in the collection and processing of samples from the Soils and Bromatology laboratories of the ‘Presidente Juárez’ Faculty of Agrobiology of the UMSNH
REFERENCES
Acosta-González, U.; Hernández-Castrejón, J.; Rebollar-Alviter, A.; Silva-Rojas, H. V.; Romero-Bautista, A. y González-Villegas, R. 2018. Effect of chemical, biological fungicides, and plant resistance inducers for the management of blackberry wilt caused by Fusarium oxysporum. Conference: International congress of plant pathology, Boston, MA. Doi:10.13140/RG.2.2.12995.04646. [ Links ]
Agrios, G. N. 2005. Plant pathology. Fifth edition. Ed. Elsevier. USA. 635 p. [ Links ]
Barnett, H. L and Hunter, B. B. 1998. Illustrated genera of imperfect fungi. 4th Ed. Burgess Publishing Company Minneapolis, Minnesota USA. 218 p. [ Links ]
Bhattacharyya, P. N. and Jha, D. K. 2011. Plant growth-promoting rhizobacteria (PGPR): emergence in agriculture. World Journal of Microbiology and Biotechnology. 28(4):1327-1350. [ Links ]
Boughalleb-M’Hamdi, N.; Salem, I. B. and M’Hamdi, M. 2018. Evaluation of the efficiency of Trichoderma, Penicillium and Aspergillus species as biological control agents against four soil-borne fungi of melon and watermelon. Egyptian Journal of Biological Pest Control. 28(1):25-36. [ Links ]
Bouziane, Z.; Dehimet, L. and Kacem Chaouch N. 2016. Inhibitory activity of Trichoderma viride against Phytophthora infestans that affects the Spunta potato (Solanum tuberosum L.) variety. African Journal of Microbiology Research. 10(29):1121-1127. [ Links ]
Cano, M. A. 2011. Interacción de microorganismos benéficos en plantas: micorrizas, Trichoderma spp. y Pseudomonas spp. una revisión. Revista U.D.C.A Actualidad y divulgación científica. 14(2):15-31. [ Links ]
Contreras-Cornejo, H. A.; Macías-Rodríguez, L.; Del-Val, E. and Larsen, J. 2016. Ecological functions of Trichoderma spp. and their secondary metabolites in the rhizosphere: interactions with plants. FEMS Microbiology Ecology. 92(4):1-21. [ Links ]
Cornelis, P.; Baysse, C. and Matthijs, S. 2008. Iron uptake in Pseudomonas. In Pseudomonas: genomics and molecular biology. Brussel, Belgium. Ed. Cornelis, P. Caister Academic Press. 213-235 pp. [ Links ]
Dhingra, O. D. and Sinclair, J. B. 1985. Basic plant pathology methods. CRC Press, Inc. Boca Raton, Florida. 355 p. [ Links ]
Gómez, R, H.; Soberanis, R. W.; Tenorio, C, M. y Torres, A. E. 2013. Manual de producción y uso de hongos antagonistas. Servicio Nacional de Sanidad Agraria. 6-7 pp. [ Links ]
Gordon, T. R.; Kong, M.; Broome, J. C.; Pastrana, A. M. and Kirkpatrick, S. C. 2017. Fusarium oxysporum f. sp. mori, a new forma specialist causing Fusarium wilt of Blackberry. Plant Diesease. 101(12):2066-2072. [ Links ]
Guédez, C.; Cañizalez, L.; Castillo, C. y Olivar, R. 2009. Efecto antagónico de Trichoderma harzianum sobre algunos hongos patógenos postcosecha de la fresa (Fragaria spp.). Revista de la Sociedad Venezolana de Microbiología. 29(1):34-38. [ Links ]
Guédez, C.; Cañizalez, L.; Castillo, C. y Olivar, R. 2012. Evaluación in vitro de aislamientos de Trichoderma harzianum para el control de Rhizoctonia solani, Sclerotium rolfsii y Fusarium oxysporum en plantas de tomate. Revista de la Sociedad Venezolana de Microbiología . 32(1):44-49. [ Links ]
Guédez, C.; Castillo, C.; Cañizalez, L. y Olivar, R. 2008. Control biológico: una herramienta para el desarrollo sustentable y sostenible. Academia. 3(13):50-74. [ Links ]
Guerra, G. A.; Betancourth, C. A. y Salazar, C. E. 2011. Antagonismo de Pseudomonas fluorescens Migula frente a Fusarium oxysporum fsp. pisi Schtdl en Arveja Pisum sativum L. Revista UDCA actualidad & divulgación científica. 14(2):33-42. [ Links ]
Hernández-Cruz, A.; Saldivia-Tejeda, A.; Silva-Rojas, H. V.; Fuentes-Aragón, D.; Nava-Díaz, C.; Martínez-Bolaños, L. and Rebollar-Alviter, A. 2020. Evaluation of full-season programs for the management of Fusarium wilt of blackberry caused by a new lineage of the Fusarium oxysporum species complex. Crop Protection. 134(2020):4-11. [ Links ]
Infante, D.; Martínez, B.; González, N. y Reyes, Y. 2009. Mecanismos de acción de Trichoderma frente a hongos fitopatógenos. Revista Protección Vegetal. 24(1):14-21. [ Links ]
Leslie, J. F. and Summerell, B. A. 2006. The Fusarium laboratory manual. Ed. Blackwell Publishing. First edition. Iowa, USA. 5-30(119):212-218. [ Links ]
Martinez, B.; Infante, D. y Reyes, Y. 2013. Trichoderma spp. y su función en el control de plagas en los cultivos. Revista Protección Vegetal . 28(1):1-11. [ Links ]
Mayo, S.; Gutiérrez, S.; Malmierca, M. G.; Lorenzana, A.; Campelo, M. P.; Hermosa, R. and Casquero, P. A. 2015. Influence of Rhizoctonia solani and Trichoderma spp. in growth of bean (Phaseolus vulgaris L.) and in the induction of plant defense-related genes. Frontiers in Plant Science. 6(685):1-11. [ Links ]
Mejía-Bautista, M. A.; Cristóbal-Alejo, J.; Tun-Suárez, J. M. y Reyes-Ramírez, A. 2016. Actividad in vitro de Bacillus spp. en la inhibición de crecimiento micelial de Fusarium equiseti y Fusarium solani aislado de chile habanero (Capsicum chinense Jacq.). Agrociencia. 50(8):1123-1135. [ Links ]
Perotti, E. B. R.; Menéndez, L. T.; Gaia, O. E. y Pidello, A. 2005. Supervivencia de Pseudomonas fluorescens en suelos con diferente contenido de materia orgánica. Revista Argentina de Microbiología. 37(2):102-105. [ Links ]
Rebollar, A. A. y Silva, R. H. V. 2019. La marchitez de la zarzamora. Diagnóstico, epidemiología y manejo integrado. Universidad Autónoma de Chapingo (UACH). 1ra. Ed. México. 10-16 pp. [ Links ]
Ricárdez-Luna, G.; Aguilar-Rivera, N.; Galindo-Tovar, M. E. y Debernardi-Vázquez, T. J. 2016. Diagnóstico de la producción de zarzamora (Rubus sp.) en la zona centro de Veracruz, México. Agroproductividad. 9(6):34-38. [ Links ]
Ruiz-Cisneros, M. F.; Ornelas-Paz, J. J.; Olivas-Orozco, G. I.; Acosta-Muñiz, C. H.; Sepúlveda-Ahumada, D. R.; Pérez-Corral, D. A. y Ríos-Velasco, C. 2018. Efecto de Trichoderma spp. y hongos fitopatógenos sobre el crecimiento vegetal y calidad del fruto de jitomate. Revista Mexicana de Fitopatología. 36(3):444-456. [ Links ]
R Core Team. 2020. R: A language and environment for statistical computing. R Foundation for Statistical Computing, Vienna, Austria. https://www.R-project.org/. [ Links ]
RStudio Team . 2020. Studio: integrated development for R. RStudio, PBC, Boston, MA. http://www.rstudio.com/. [ Links ]
SIAP. 2021. Servicio de Información Agropecuaria y Pesquera. Anuario estadístico de la producción agrícola. Cierre de la producción agrícola. https://nube.siap.gob.mx/cierreagricola/. [ Links ]
Singh, J. S. and Singh, D. P. 2013. Plant growth promoting rhizobacteria (PGPR): microbes in sustainable agriculture. Management of Microbial Resources in the Environment. 361-385 pp. [ Links ]
Susan, V. R. y Castiel, A. F. 2005. Control biológico de plagas y enfermedades de los cultivos. En: Biotecnología y medioambiente. Ed. Ephemera. Madrid. 215-230 pp. [ Links ]
Tejera-Hernández, B.; Rojas-Badía, M. M. y Heydrich-Pérez, M. 2011. Potencialidades del género Bacillus en la promoción del crecimiento vegetal y el control biológico de hongos fitopatógenos. CENIC Ciencias Biológicas. 42(3):131-138. [ Links ]
Tian, L.; Shi, S.; Ji, L.; Nasir, F.; Ma, L. and Tian, C. 2018. Effect of the biocontrol bacterium Bacillus amyloliquefasciens on the rhizosphere in ginseng plantings. International Microbiology. 21(3):153-162. [ Links ]
Trigiano, N. R.; Windham, T. M. and Windham, S. A. 2004. Plant pathology, concepts, and laboratory excercises . CRC Press. 413 p. [ Links ]
Vásquez-Ramírez, L. M. y Castaño-Zapata, J. 2017. Manejo integrado de la marchitez vascular del tomate Fusarium oxysporum f. sp. lycopersici (SACC.) W. C. Snyder & H. N. Hansen: una revisión. Revista UDCA. Actualidad & Divulgación Científica. 20(2):363-374. [ Links ]
Villa, P. M.; Frías, A. y González, G. 2005. Evaluación de cepas de Pseudomonas sp para el control de hongos fitopatógenos que afectan cultivos de interés económico. ICIDCA Sobre los derivados de la caña de azúcar. 39(3):40-44. [ Links ]
Received: January 01, 2024; Accepted: March 01, 2024