Servicios Personalizados
Revista
Articulo
Indicadores
-
Citado por SciELO
-
Accesos
Links relacionados
-
Similares en SciELO
Compartir
Revista mexicana de ciencias pecuarias
versión On-line ISSN 2448-6698versión impresa ISSN 2007-1124
Rev. mex. de cienc. pecuarias vol.10 no.1 Mérida ene./mar. 2019
https://doi.org/10.22319/rmcp.v10i1.4327
Technical Notes
Mean lethal dose (LD 50 ) and growth reduction (GR 50 ) due to gamma radiation in Wilman lovegrass (Eragrostis superba)
a Instituto del Nacional de Investigaciones Forestales, Agrícolas y Pecuarias (INIFAP). Campo Experimental La Campana. Carretera Chihuahua-Ojinaga, Km 33.3. Aldama, Chihuahua, México.
b Universidad Autónoma de Chihuahua. Facultad de Zootecnia y Ecología. Perif. Francisco R. Almada, Km 1. 31000 Chihuahua, Chihuahua, México.
c Instituto del Nacional de Investigaciones Forestales, Agrícolas y Pecuarias (INIFAP). Campo Experimental Rosario Izapa, Chiapas. México.
d Servicio Nacional de Sanidad, Inocuidad y Calidad Agroalimentaria SENASICA. Departamento de Irradiación; complejo MOSCAMED-MOSCAFRUT, Metapa de Domínguez, Chiapas. México.
Gamma radiation can be utilized as a mutagenic agent for inducing genetic variability in plant species. However, the adequate dose of radiation must be established before starting a mutagenesis-based breeding program. The objective was to determine the mean lethal dose (LD50) and the dose for the mean growth reduction (GR50) in Wilman lovegrass (Eragrostis superba Peyr.). For this purpose, the seeds were radiated with doses of 0 (control treatment), 100, 200, 300, 450, 600, 900, 1400, 2000 and 4000 Gray. The evaluated variables were germination percentage, germination speed index, plumule length, radicle length, forage yield, seed production, leaf-stem ratio and chlorophyll concentration index. The data were analyzed through regression analyses and the Dunnett’s mean comparison test. The LD50 and GR50 generated for each of the evaluated variables were calculated with the resulting regression equation. Based on the values of LD50 and GR50, a weighted mean was estimated; the LD50 was weighted with 30 % while the GR50 with 10%. In general, all the evaluated characteristics were affected by the radiation treatment (P<0.05), and their behavior was adjusted to linear square models (P<0.05), allowing the determination of the LD50 and the GR50. The weighting average of both parameters, estimated at 2486 Gy, is recommended for the genetic breeding of Wilman lovegrass. The results of this study will allow implementing mutagenesis-based plant breeding programs in Wilman lovegrass.
Key words: Cobalt 60; Gamma radiation; Mutations; DL50; RC50
La radiación gamma puede ser utilizada como agente mutagénico para inducir variabilidad genética en especies vegetales. No obstante, antes de comenzar un programa de mejoramiento mediante mutagénesis, es necesario establecer la dosis de irradiación adecuada. El objetivo fue determinar la dosis letal media (DL50) y de reducción media del crecimiento (RC50) en pasto garrapata (Eragrostis superba Peyr.). Para ello, se irradió semilla con dosis de 0 (tratamiento testigo), 100, 200, 300, 450, 600, 900, 1400, 2000 y 4000 Gray. Las variables evaluadas fueron porcentaje de germinación, índice de velocidad de germinación, longitud de plúmula, longitud de radícula, rendimiento de forraje, producción de semilla, proporción hoja-tallo e índice de concentración de clorofila. Los datos se analizaron por regresión y comparación de medias con la prueba de Dunnett. Con la ecuación de regresión resultante se calculó la DL50 y RC50 para cada variable. Con estos valores se obtuvo una media ponderada, donde la DL50 se ponderó con 30 % y las RC50 con 10 %. En general, todas las características evaluadas se vieron afectadas por la irradiación (P<0.05), y su comportamiento se ajustó a modelos lineales y cuadráticos (P<0.05), lo cual permitió determinar la DL50 y las RC50. Para el mejoramiento genético del pasto garrapata, se recomienda utilizar la ponderación de ambos parámetros, la cual se obtuvo a los 2,486 Gy. Los resultados de este estudio permitirán la implementación de programas de mejoramiento genético por mutagénesis en pasto garrapata.
Palabras clave: Cobalto 60; Rayos gamma; Mutaciones; DL50; RC50
The median lethal dose (LD50) and the median growth reduction (GR50) are parameters utilized to establish the adequate irradiation dose to induce mutations in plant breeding programs. Mutation induction has the purpose of generate genetic and phenotypic variability, which may allow to select plants with characteristics that are not found in nature. Several researchers agree that the highest probability to generate useful mutations for breeding programs occurs at doses where the 50% of the irradiated individuals die1-4. Likewise, other researchers have pointed out that another dose with a high probability of producing effective mutations, besides the LD50, is where the 50% of a growth reduction (GR50) occurs5,6. Notably, both parameters (LD50 and GR50) are based on the assumption that low doses of irradiation produce minimum impacts on the genome, which rarely generate phenotypic changes; whereas high doses may produce multiple impacts on the genome which consistently produce aberrations or negative changes7,8. Therefore, the first step in a mutagenesis-based breeding process is to determine the LD50 and the GR50.
In Mexico, most of the arid and semi-arid grasslands are degraded due to the overgrazing and other anthropogenic practices9. Such degradation has caused a drop in the vegetation cover, large stretches of bare ground and biodiversity loss, reducing the functionality and productivity of these ecosystems. An alternative to rehabilitate these degraded systems is the revegetation by seeding, for which the use of native vegetation is recommended. Nevertheless, this type of vegetation has a limited establishment ability in areas with a high degree of degradation.
Therefore, the alternative is to use introduced species with a higher rusticity and establishment ability, like the Wilman lovegrass (Eragrostis superba). This grass is considered one of the species with the highest potential to be used in grassland rehabilitation programs10, as it has a higher establishment ability than the native species used in this type of programs, like sideoats grama (Bouteloua curtipendula) and blue grama (Bouteloua gracilis), and even introduced species like weeping lovegrass (Eragrostis curvula)11. However, it has a lower nutritional value and forage yield than other introduced grasses, such as buffel grass (Pennisetum ciliare), klein grass (Panicum coloratum) and even weeping lovegrass12. The aforementioned suggests the possibility to subject the Wilman lovegrass to a plant breeding process. Nevertheless, since it is an introduced species, there may be little genetic variability of this species in Mexico. In this sense, mutagenesis may be an alternative for the genetic improvement of Wilman lovegrass, as it has proven to be a viable technique for inducing variability in grasses13,14,15. However, the dose with the highest probabilities of producing effective mutations in this species is unknown so far. For this reason, the objective was to determine the LD50 and the GR50 in Wilman lovegrass in order to establish the optimal dose of gamma radiation for mutagenesis induction into this species.
The experiment was carried out under laboratory and greenhouse conditions. Seeds of Wilman lovegrass var. Palar were radiated. The seeds were imported from the Curtis & Curtis company, located in Clovis, New Mexico, USA. Nine radiation doses and a control were evaluated: 0 (control), 100, 200, 300, 450, 600, 900, 1400, 2000 and 4000 Gray (Gy). The exposure times for the evaluated doses were determined using the Gafchromic dosimetry system and a RADCAL® Accu-dose ion chamber (Serial No. 4094118, USA). The seeds were radiated at the MOSCAFRUT SAGARPA//IICA Complex in Metapa de Domínguez, Chiapas, Mexico, in collaboration with the “Rosario Izapa” Experimental Station of INIFAP in Chiapas. The doses were applied within a Nordion GammaBeamTM-127 panoramic Gamma irradiator, with a dry storage source and an activity of 15 000 Curies of Co-60.
The experimental design utilized for this test was completely randomized, with 10 radiation treatments and four repeats per dose, and considering each 90 mm Petri dish with cotton and filter paper and 50 seeds per repeat as the experimental unit. The Petri dishes were moisturized with 25 ml of water at the beginning of the test, and were subsequently sprayed with 2.0 ml every other day during the 15 d of duration of the test. The Petri dishes were then placed in a Precision Scientific 6M incubator at a temperature of 28 ± 2 °C. Those seeds that attained a 0.5 cm plumule or radicle were considered as germinated16. The assessed variables were: germination percentage (GP), germination speed index (GSI), plumule length (PL), and radicle length (RL). In order to obtain the RL and the PL, three seedlings per Petri dish were allowed to grow for 7 d after germination. The GSI was estimated using the following equation16:
Where: GSI = germination speed, n¡ = number of seeds germinated per day, t = day of germination.
The greenhouse experiment was assessed using a randomized block experimental design with 10 repeats per treatment, establishing the blocks perpendicularly at the sunlight expose during the day. Twenty (20) seeds were sown in each flowerpot in order to ensure emergence and establishment; however, only one plant was left per pot. The seeds were sown in black polyethylene bags with a height of 26 cm and a diameter of 18 cm, perforated at the bottom. The bags were filled with 23 cm of alluvial loamy sandy soil. They were watered up to the saturation point every two or three days, according to the desiccation of the soil. The test was conducted during 16 wk from April to August. The assessed variables were forage yield (FY), seed production (SP), leaf:stem ratio (LSR), and chlorophyll concentration index (CCI). All the seeds of each plant were collected and put into paper bags in order to calculate the SP. For the FY, the biomass was cut at 5 cm from the ground; the stems and the leaves were then separated and placed in paper bags. The samples of both forage and seeds were dried in an oven at 65 °C during 72 h. Once the samples were dry, they were weighed in a Mettler Viper BC analytical scale. The CCI was measured at the middle part of five leaves selected at random, using an Opti-Sciences CCM-200 meter.
In order to ensure the ranges of temperature (T) and relative humidity (RH) required by Wilman lovegrass, measurements were taken at the nursery with an HMP60 probe (Vaisala, Woburn, MA, USA). These data were registered every second and were averaged every minute in a CR1000 datalogger (Campbell Scientific Inc., Logan, UT, USA). The mean T during the experiment was 26.7 ± 5.6 °C, with a minimum temperature of 17.1 °C and a maximum temperature of 44.7 °C. The mean RH was 52.0 ± 16.8 %.
The data were analyzed with a regression analysis for each variable. The mean lethal dose (LD50) was estimated using the resulting regression equation from the germination percentage. The same procedure was utilized to estimate the mean growth reduction (GR50) of the variables germination speed index, plumule length, radicle length, forage yield, seed weight, leaf:stem ratio, and chlorophyll concentration index. Furthermore, all the data were subjected to a variance analysis and to Dunnett’s mean comparison test, where the influence factor was the radiation dose with a significance level of 0.05 (α=0.05). The analyses were performed using the REG and GLM procedures of the SAS 9.1.3 statistical package17.
A weighted mean was estimated based on the results of the LD50 and the GR50 obtained for the eight variables. The LD50 was weighted with 30 %, and the GR50, with 10 % for the remaining variables (GP, GSI, PL, RL, FY, SP, LSR, and CCI). A single value was thus obtained, whereby the optimal dose of gamma radiation for inducing mutagenesis in Wilman lovegrass was established. The germination percentage was the variable to which the highest weighting was assigned, because the death of the individual indicates the maximum damage that radiation can produce.
The behavior of the GP exhibited a quadratic tendency (P=0.008). Furthermore, there were differences (P<0.05) between all the radiation doses and the control treatment (T-0). In general, the GP tended to diminish as the radiation dose increased (Figure 1a). The regression equation indicates that the LD50 in Wilman lovegrass occurs at 1,529.1 Gy. Likewise, the GSI exhibited a quadratic tendency (P=0.001), and there were differences (P<0.05) between all the radiation treatments and the T-0. The GSI also diminished as the radiation dose increased (Figure 1b). According to the regression equation, for this variable, the GR50 occurred at 805.3 Gy.
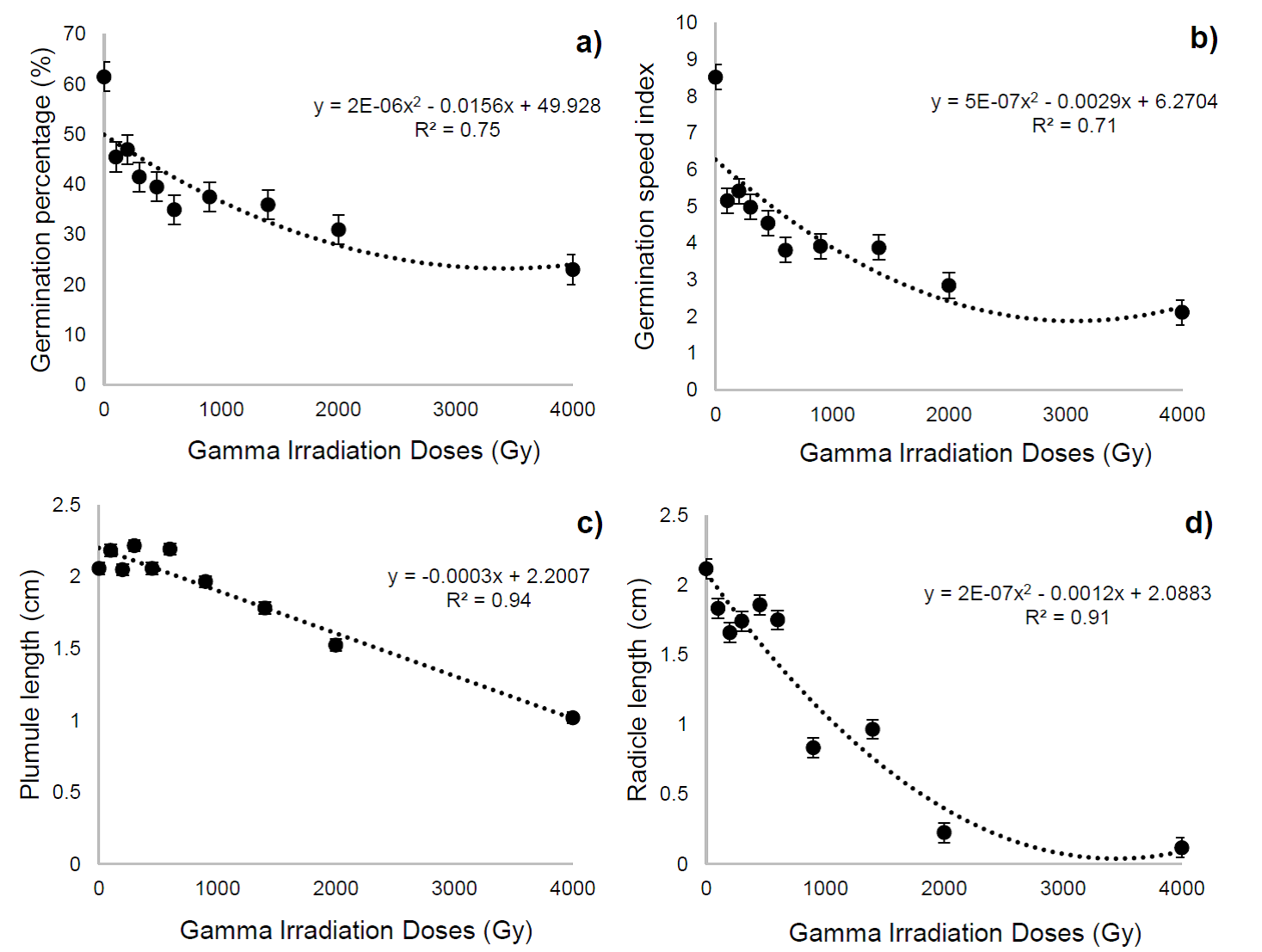
Figure 1: Effect of different doses of radiation with Co-60 on the germination percentage (a), the germination speed index (b), the plumule length (c) and the radicle length (d) of Wilman lovegrass (Eragrostis superba)
The PL exhibited a negative linear tendency (P<0.0001). However, only the doses of 2,000 and 4,000 Gy exhibited a lower PL (P<0.05) than the T-0 (Figure 1c). According to the regression equation, in this variable the GR50 occurred at 3,905.3 Gy. Likewise, the RL exhibited a quadratic tendency (P<0.0001). For this variable, only the 900, 1400, 2000 and 4000 Gy treatments had a lower RL (P<0.05) than the T-0 (Figure 1d). The equation showed that, for this variable, the GR50 occurred at 1 037.8 Gy.
The behavior of the FY exhibited a quadratic tendency (P=0.0009). There was an increase in this variable (P<0.05) with the doses of 450 and 600 Gy. It subsequently tended to diminish until it reached 4000 Gy, with which dose all the plants died before reaching 21 days of age (Figure 2a). The regression equation for this variable indicates that the GR50 occurred at 2,262.8 Gy.
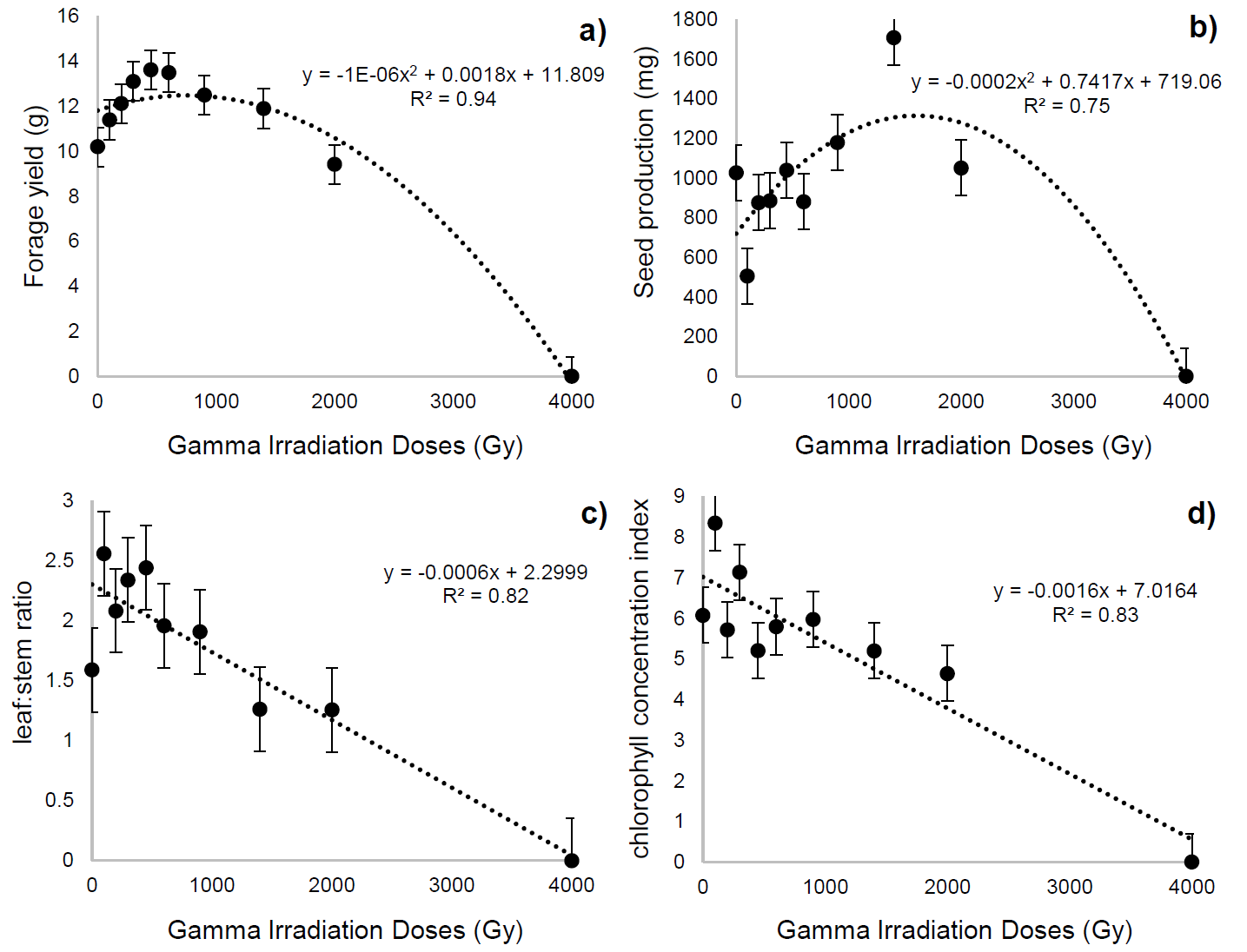
Figure 2: Effect of different doses of radiation with Co-60 on the forage yield (a), seed production (b), leaf:stem ratio (c), and chlorophyll concentration index (d) of Wilman lovegrass (Eragrostis superba)
The SP exhibited a quadratic tendency (P<0.05). However, the only treatments with differences (P<0.05) in relation to the control were 1,400 and 4,000 Gy. There was an increase in the SP with the 1,400 Gy dose (P<0.05; Figure 2b). According to the regression equation, the GR50 on the SP occurred at 3,968.7 Gy. The LSR showed a linear tendency (P<0.0001). The only treatment that differed (P<0.05) from the control was 4,000 Gy radiation, because all the plants with this treatment died, and therefore the LSR was equal to 0. The GR50 for this variable occurred at 2,509.9 Gy. The CCI exhibited a linear behavior (P<0.0001). However, as the former variable, the only treatment that was different (P<0.05) from the control was 4,000 Gy. According to the regression equation, the GR50 occurred at 2,486.6 Gy. The weighted mean of the LD50 and GR50 resulting from the eight variables was 2 159.2 Gy.
Wilman lovegrass germination decreased as the radiation dose increased. This result agrees with those of several authors, who found that the germination of various plant species tends to diminish with increasing radiation doses18-21. The reason for this may be that high doses of radiation can inhibit functions that are vital to the cells, which may result in the death of certain cells, and even of the embryo in the seed. This phenomenon tends to increase with the radiation dose, and it is the cause of reduced germination20. The radiation may damage compounds related to the plant metabolism -such as auxins, ascorbic acid, chlorophyll, and proteins-, potentially inhibiting the growth of the seedlings22,23. It has been observed that, due to these disorders, seeds with high radiation doses cannot germinate, or their seedlings cannot survive beyond a few days24, as was the case with the 4,000 Gy dose in this study. Furthermore, germination reduction has been ascribed to the fact that the frequency in chromosomal damage augments with increasing doses22. This may change the expression of proteins and influence the functioning of the cells, potentially affecting the development of the seedlings and preventing germination25.
One of the grasses whose radiosensitivity has been most extensively studied is rice (Oryza sativa). A study of this species exposed 13 varieties to gamma radiation, and found that the LD50 ranged, according to the variety, between 345 and 423 Gy26. Another study carried out in two varieties of rice established the LD50 at 288 and 354 Gy27, while other researchers established it at 229 Gy28. In other grasses, such as Pennisetum glaucum and Pennisetum typhoides, the LD50 has been determined at 669.3 and 200 Gy, respectively28,29. As for forage grasses, the LD50 determined for different varieties of sudan grass (Sorghum sudanense) ranged between 307 and 342 Gy3. Notably, the same agent was utilized in the above studies as in the present one. However, the results are lower than the LD50 obtained in this study for Wilman lovegrass. This result may be due, among other factors, to the variations in the moisture content of the seed between species, since the ionizing radiation may break the covalent bonds and cause the breakdown of water molecules, forming free radicals that may indirectly damage the various organelles of the cell and even the DNA molecules within the cell30.
Like the GP, the GSI of Wilman lovegrass tended to diminish with increasing radiation doses. This result agrees with the findings reported for peanut (Arachis hypogaea), where the germination speed diminished with the increased dose of radiation31. The delay in germination may be due to the fact that high radiation doses may inhibit processes that are vital for the cell, such as protein synthesis and enzyme activity, which can delay cell division and, therefore, germination20,32.
The PL and the RL were observed to diminish with increased radiation doses. This result was also found for Eleusine coracana, in which the PL and RL were affected by the increase in the radiation dose, and the GR50 occurred at 500 Gy for both variables33. The same effect was found in rice, where the GR50 occurred at 250 and 450 Gy, respectively, for the two variables34.
Reduction of the plumule and radicle lengths may be due to the fact that ionizing radiation usually affects plant metabolism and cell division, inhibiting or delaying the plant’s growth20,31. In this regard, Kiong et al22 found that gamma radiation can diminish the amount of proteins and chlorophyll compounds, affecting the plant metabolism. On the other hand, Preuss and Britt35 found that the gamma radiation may inhibit plant growth because plants have a signal transduction mechanism that monitors cellular damage. This mechanism stops cell division whenever the cell structure is damaged.
The FY and SP of Wilman lovegrass presented an increase with low radiation doses. This may be due to the fact that gamma radiation can increase the amount of photosynthetic pigments like a- and b- chlorophylls and carotenoids22. These pigments capture the sunlight photons for the plant to convert into energy compounds through photosynthesis36. Therefore, an increase in the concentration of these pigments may increase the photosynthetic rates; this may have been the cause of the increase in forage yield and seed production in this study. The increase in FY and SP may also be due to the fact that gamma radiation sometimes increases the activity of antioxidant enzymes such as the superoxide dismutase and glutathione reductase, reducing the oxidative stress in the plant. This may help the plant deal more easily with the stress factors it faces on a daily basis, such as the fluctuations of temperature and sunlight that it receives, benefitting its growth37,38.
Nevertheless, after the increase in FY and SP, there was a decrease. This is due to the fact that radiation can also cause negative effects, as the exposure to gamma radiations frequently produces free radicals during the water radiolysis process. These free radicals are unstable chemical species that may damage cell organelles. In an experiment conducted by Wi et al.39, gamma radiation was observed to produce important modifications in the structure of certain cell organelles of Arabidopsis thaliana. In this experiment, the tilacoids within the chloroplasts exhibited dilation and damages; the mitochondria displayed distorted forms, and the endoplasmic reticulum exhibited distended membranes. In addition, as it was mentioned above, radiation may cause the destruction of auxins, ascorbic acid, chlorophyll, and proteins, affecting the metabolism of the plants22,23. It is unlikely that any of these phenomena may occur with low radiation doses; however, as the radiation dose augments, the probability of their occurrence increases22. This may be why, after an increase in forage yield and seed production, there was a reduction of both variables.
The LD50 and the GR50 are parameters utilized for determining the optimal radiation dose to induce effective mutations in genetic improvement programs. This is because the impact on the genome has been observed to be minimal and difficult to reflect, while with high doses, the genome may suffer multiple impacts that consistently produce anomalies or negative changes7,8. Furthermore, low radiation doses may induce an increase in the cell division, the enzyme activity or the amount of photosynthetic pigments31,34. In contrast, high radiation doses may also produce physical damage to the organelles or chemical damages in the cells, due to the destruction of enzymes and hormones, among others. However, because these changes were not produced through genetic modifications, they will not pass on to the next generations. In this sense, low and high radiation doses may generate in plants phenotypic changes that may be mistaken for genetic changes. Therefore, the optimal radiation dose must be established before starting a mutagenesis-based genetic improvement program; this will increase the possibility of finding effective mutations. The optimal radiation dose is established through the mean lethal dose or the mean growth reduction dose6,7. In this study, the reduction that occurred in the values of all the variables due to radiation made it possible to determine the LD50 and GR50. However, the value of both parameters in each variable ranged between 805 and 3,905 Gy. Because of the amplitude of this range, a weighed mean was estimated in order to establish the optimal dose of the gamma radiation in the seed. The variable to which the highest weighting was assigned was the germination percentage, as the death of the individual indicates the maximum damage that radiation can produce.
Low gamma radiation doses can produce an increase in certain agronomic attributes in Wilman lovegrass. However, in general all the assessed physical parameters were affected by high doses of gamma radiations, which made it possible to determine the mean lethal dose and the mean growth reduction. In order to attain the genetic improvement of Wilman lovegrass, it recommends using the optimal radiation dose obtained through the weighting of both parameters (2,486 Gy). This will contribute to the obtainment of new genetic material that can be utilized for the rehabilitation of grasslands.
Literatura Citada
1. Lajonchere G, Mesa AR, Prieto M, Sánchez E. Curva de radiosensibilidad con 60Co en guinea (Panicum maximum Jacq.) cv. K-249. Rev Pastos y Forrajes 1995;18:1-8. [ Links ]
2. Morela F, González V, Castro L. Efecto de la radiación Gamma sobre la diferenciación de plantas de caña de azúcar a partir de callos. Agron Trop 2002;52:311-323. [ Links ]
3. GolubInova I, Gecheff K. M1 cytogenetic and physiological effects of gamma-rays in sudan grass (Sorghum Sudanense (piper.) stapf). Bulg J Agric Sci 2011;17:417-423. [ Links ]
4. Ángeles-Espino A, Valencia-Botín AJ, Virgen-Calleros G, Ramírez-Serrano C, Paredes-Gutiérrez L, Hurtado-De la Peña S. Determinación de la dosis letal (DL50) con Co60 en vitroplántulas de Agave tequilana var. Azul. Rev Fitotec Mex 2013;36:381-386. [ Links ]
5. Akgüm I, Tosun M. Agricultural and cytological characteristics of M1 perennial rye (Secale montanum Guss.) as affected by the application of different doses of gamma rays. Pak J Biol Sci 2004;7:827-833. [ Links ]
6. Khalil SA, Zamir R, Ahmad N. Effect of different propagation techniques and gamma irradiation on major steviol glycoside’s content in Stevia rebaudiana. The J Anim Plant Sci 2014;24:1743-1751. [ Links ]
7. Songsri P, Suriharn B, Sanitchon J, Srisawangwong S, Kesmala T. Effects of gamma radiation on germination and growth characteristics of physic nut (Jatropha curcas L.). J Biol Sci 2011;11:268-274. [ Links ]
8. Thole V, Peraldi A, Worland B, Nicholson P, Doonan JH, Vain P. T-DNA mutagenesis in Brachypodium distachyon. J Exp Bot 2011;10:1-10. [ Links ]
9. Melgoza-Castillo A, Ortega-Ochoa C, Morales-Nieto CR, Jurado-Guerra P, Velez-Sanchez-Verin C, Royo-Márques MH, et al. Propagación de plantas nativas para la recuperación de áreas degradadas: opción para mejorar ecosistemas. Tecnociencia 2007;3:38-41. [ Links ]
10. Sáenz-Flores E, Saucedo-Terán RA, Morales-Nieto CR, Jurado-Guerra P, Lara-Macías CR, Melgoza-Castillo A, et al. Producción y calidad de semilla de pastos forrajeros como respuesta a la fertilización en Aldama, Chihuahua. Tecnociencia 2015;9:111-119. [ Links ]
11. Esqueda CMH, Melgoza CA, Sosa CM, Carillo RR, Jiménez CJ. Emergencia y sobrevivencia de gramíneas con diferentes secuencias de humedad/sequía en tres tipos de suelo. Téc Pecu Méx 2005;43:101-115. [ Links ]
12. Sanderson MA, Voigt P, Jones RM. Yield and quality of warm-season grasses in central Texas. J Range Manage. 1999;52:145-150. [ Links ]
13. López CE, Prina A, Griffa S, Ribotta AN, Carloni E, Tommasino E, Luna C, Biderbost E, Grunberg K. Obtaining new germplasm inCenchrus ciliarisL. through induced-mutation and in vitro selection. Phyton 2011;80:59-64. [ Links ]
14. Pongtongkam P, Nilratnisakorn S, Piyachoknakul S, Thongpan A, Aranananth J, Kowitwanich K, Tadsri S. Inducing salt tolerance in purple guinea grass (Panicum maximum TD58) via gamma irradiation and tissue culture. Nat Sci 2005;39:681-688. [ Links ]
15. Pongtongkam P, Peyachoknagul S, Arananant J, Thongpan A, Tudsri S. Production of salt tolerance dwarf napier grass (Pennisetum purpureum cv. Mott) using tissue culture and gamma irradiation. Nat Sci 2006;40:625-633. [ Links ]
16. Maguire JD. Speed of germination-aid in selection and evaluation for seedling emergence and vigor. Crop Sci 1962;2:176-177. [ Links ]
17. Statistical Analysis System (SAS) Institute Inc. User´s guide. Vesion 9.1.3 Cary, NC, USA. 2006. [ Links ]
18. Anbarasan K, Rajendran R, Sivalingam D, Anbazhagan M, Chidambaram AA. Effect of gamma radiation on seed germination and seedling growth of Sesame (Sesamum indicum .L.) Var.TMV3. Int J Res Bot 2013;3:27-29. [ Links ]
19. Bharathi T, Gnanamurthy S, Dhanavel D, Murugan S, Ariraman M. Induced Physical mutagenesis on seed germination, lethal dosage and morphological mutants of Ashwagandha (Withania somnifera (L.) Dunal). Int J Adv Res 2013;1:136-141. [ Links ]
20. Olasupo FO, Ilori CO, Forster BP, Bado S. Mutagenic Effects of Gamma Radiation on Eight Accessions of Cowpea (Vigna unguiculata [L.] Walp.). Am J Plant Sci 2016;7:339-351. [ Links ]
21. Rajarajan D, Saraswathi R, Sassikumar D. Determination of lethal dose and effect of gamma ray on germination percentage and seedling parameters in ADT (R) 47 rice. IJABR 2016;6:328-332. [ Links ]
22. Kiong ALP, Lai AG, Hussein S, Harun AR. Physiological responses of Orthosiphon stamineus plantlets to gamma irradiation. Am-Eurasian J Sustain Agric 2008;2:135-149. [ Links ]
23. Shah TM, Mirza JI, Haq MA, Atta BM. Radio sensitivity of various chickpea genotypes in M1 generation I-Laboratory studies. Pak J Bot 2008;40:649-665. [ Links ]
24. Marcu D, Damian G, Cosma C, Cristea V. Gamma radiation effects on seed germination, growth and pigment content, and ESR study of induced free radicals in maize (Zea mays). J Biol Phys 2013;39:625-634. [ Links ]
25. Cheng L, Yang H, Lin B, Wang Y, Li W, Wang D, Zhang F. Effect of gamma-ray radiation on physiological, morphological characters and chromosome aberrations of minitubers in Solanum tuberosum L. Int J Radiat Biol 2010;86:791-799. [ Links ]
26. Harding SS, Johnson SD, Taylor DR, Dixon CA, Turay MY. Effect of gamma rays on seed germination, seedling height, survival percentage and tiller production in some rice varieties cultivated in Sierra Leone. Am J Exp Agric 2012;2:247-255. [ Links ]
27. Ramchander S, Ushakumari R, Pillai MA. Lethal dose fixation and sensitivity of rice varieties to gamma radiation. Indian J Agr Res 2015;49:24-31. [ Links ]
28. Ousmane SD, Elegba W, Danso K. Radio-sensibility of pearl millet (Pennisetum glaucum (L.) R. Br.) and cowpea (Vigna unguiculata (L.) Walp.) seeds germination and seedling growth. IJIAS 2013;4:665-671. [ Links ]
29. Ambli K, Mullainathan L. Effect of gamma rays and ems on seed germination and seed characters in pearl millet (Pennisetum typhoides) (Burn.)Stapf. Var. CO (Cu)-9. J Chem Biol Phys Sci 2014;4:3345-3349. [ Links ]
30. Alegre BN. Reacción celular ante la radiación. Radiobiología 2001;1:9-11. [ Links ]
31. Aparna M, Chaturvedi A, Sreedhar M, Kumar DP, Venu-Babu P, Singhal RK. Impact of gamma rays on the seed germination and seedling parameters of groundnut (Arachis Hypogaea L.). Asian J Exp Biol Sci 2013;4:61-68. [ Links ]
32. Chandrashekar KRA. Gamma sensitivity of forest plants of Western Ghats. J Environ Radioact 2014;132:100-107. [ Links ]
33. Ambavane AR, Sawardekar SV, Sawantdesai SA, Gokhale NB. Studies on mutagenic effectiveness and efficiency of gamma rays and its effect on quantitative traits in finger millet (Eleusine coracana L. Gaertn). J Radiat Res Appl Sci 2014;8:120-125. [ Links ]
34. Talebi AB, Talebi AB. Radiosensitivity Study for Identifying the Lethal Dose in MR219 (Oryza sativa L. spp. Indica cv. MR219). IJASRT 2012;2:63-67. [ Links ]
35. Preuss SB, Britt AB. "A DNA-damage-induced cell cycle checkpoint in Arabidopsis." Genetics 2003;164:323-334. [ Links ]
36. Jifon JL. Growth environment and leaf anatomy affect nondestructive estimates of chlorophyll and nitrogen in Citrus Sp. leaves. J Am Soc Hortic Sci 2005;130:152-158. [ Links ]
37. Kim JH, Baek MH, Chung BY, Wi SG, Kim JS. Alterations in the photosynthetic pigments and antioxidant machineries of red pepper (Capsicum annuum L.) seedlings from gamma-irradiated seeds. J Plant Biol. 2004;47:314-321. [ Links ]
38. Wi SG, Chung BY, Kim JS, Kim JH, Baek MH, Lee JW, Kim YS. Effects of gamma irradiation on morphological changes and biological responses in plants. Micron 2007;38:553-564. [ Links ]
39. Wi SG, Chung BY, Kim JH, Baek MH, Yang DH, Lee JW, Kim JS. Ultrastructural changes of cell organelles in Arabidopsis stem after gamma irradiation. J Plant Biol 2005;48:195-200. [ Links ]
Received: November 15, 2016; Accepted: May 01, 2018