Servicios Personalizados
Revista
Articulo
Indicadores
-
Citado por SciELO
-
Accesos
Links relacionados
-
Similares en SciELO
Compartir
Tecnología y ciencias del agua
versión On-line ISSN 2007-2422
Tecnol. cienc. agua vol.11 no.5 Jiutepec sep./oct. 2020 Epub 10-Jun-2024
https://doi.org/10.24850/j-tyca-2020-05-04
Articles
Understory contribution to water vapor and CO2 fluxes from a subtropical shrubland in northwestern Mexico
1Instituto Tecnológico de Sonora, Departamento de Ciencias del Agua y del Medio Ambiente, Ciudad Obregón, Sonora, México, vargastml@gmail.com
2Instituto Tecnológico de Sonora, Departamento de Ciencias del Agua y del Medio Ambiente, Ciudad Obregón, Sonora, México, enrico.yepez@itson.edu.mx
3University of Delaware, Department of Plant and Soil Sciences, Newark, USA, tonantzin.tarin@gmail.com
4University of Nottingham, Division of Plant and Crop Sciences, Sutton Bonington, Leicestershire, UK, carlos.robleszazueta@nottingham.ac.uk
5Instituto Tecnológico de Sonora, Departamento de Ciencias del Agua y del Medio Ambiente, Ciudad Obregón, México, jaime.garatuza@itson.edu.mx
6Universidad de Sonora, Departamento de Agricultura y Ganadería, Hermosillo, México, jcrod@guayacan.uson.mx
7Universidad de Sonora, Departamento de Física, Hermosillo, México, cwatts@correo.fisica.uson.mx
8 Arizona State University, School of Earth and Space Exploration, School of Sustainable Engineering and the Built Environment, Tempe, USA, vivoni@asu.edu
The net ecosystem production and evapotranspiration (ET) of arid and subtropical ecosystems is poorly understood by the lack of measurements of CO2 and water vapor fluxes. Moreover, the contributions related to the fluxes in the different strata (i.e. understory) have been poorly studied. The present investigation estimates ET and CO2 fluxes of a shrubby understory with the presence of Mimosa distachya from a subtropical shrubland in relation to the net estimates of ecosystem ET and net CO2 fluxes determined with the eddy covariance technique. Instantaneous ET and CO2 exchange measurements were carried in four plots for different time periods during the day (9, 11, 14 and 18 hours) using a static chamber (16.4 m3) coupled with a fast response infrared gas analyzer. The variation of the fluxes during diurnal cycles was used to integrate the magnitude of the flux during the daylight hours. There was great variation in the understory fluxes, during July and September where there was more CO2 gain from the atmosphere towards the ecosystem with a net CO2 exchange of -1.35 ± 1.93 g C m-2 d-1 and -1.15 ± 0.74 g C m-2 d-1, respectively, indicating that photosynthesis was higher than respiration in this stratum. In a dry period during August the CO2 flux was -0.85 ± 0.73 g C m-2 d-1. During these periods, ET was 3.63 ± 0.15 mm d-1 in July; 2.71 ± 0.08 mm d-1 in August, and 1.59 ± 0.5 mm d-1 in September. Comparing these results with the total ecosystem ET and the net CO2 exchange, it was found that the understory contributes with 17 and 21% to CO2 fluxes and from 25 to 39% in water vapor fluxes during the North American Monsoon.
Keywords: Biogeosciences; ecohydrology; understory; evapotranspiration; net CO2 exchange; Mimosa distachya
La productividad neta del ecosistema y evapotranspiración (ET) de los ecosistemas áridos y subtropicales es poco entendida por la escasez de mediciones de flujos de CO2 y vapor de agua. Todavía las contribuciones relativas a los flujos en los diferentes estratos (p. ej., sotobosque) han sido pobremente analizadas. Este estudio estima ET y los flujos de CO2 en un estrato arbustivo con presencia de Mimosa distachya de un matorral subtropical, en relación con estimaciones netas de la ET y el flujo de CO2 del ecosistema, determinado con la técnica de covarianza de vórtices. Se tomaron lecturas instantáneas de ET y del intercambio de CO2 en cuatro parcelas durante distintos periodos durante el día (9, 11, 14 y 18 horas), usando una cámara estática (16.4 m3), equipada con un analizador de gases infrarrojo de rápida respuesta. La variación de flujos durante los periodos de medición se usó para integrar la magnitud del flujo durante el día. Se presentó gran variación en los flujos durante julio y septiembre, donde hubo más ganancia de CO2 de la atmósfera hacia el ecosistema, con un intercambio neto de CO2 de -1.35 ± 1.93 g C m-2 d-1 y -1.15 ± 0.74 g C m-2 d-1, para cada mes, respectivamente, lo cual indica que la fotosíntesis fue más alta que la respiración en este estrato. En un periodo seco durante agosto, el flujo de CO2 fue de -0.85 ± 0.73 g C m-2 d-1. Durante estos periodos, el ET fue de 3.63 ± 0.15 mm d-1 en julio; 2.71 ± 0.08 mm d-1 en agosto, y 1.59 ± 0.5 mm d-1 en septiembre. Comparando estos resultados con los flujos netos de vapor de agua y CO2 del ecosistema, se encontró que el estrato arbustivo aporta entre 17 y 21% a los flujos de CO2 y de 25 a 39% en los flujos de vapor de agua durante el monzón de Norteamérica.
Palabras clave: biogeociencias; ecohidrología; sotobosque; evapotranspiración; intercambio neto de CO2; Mimosa distachya
Introduction
Arid and semi-arid ecosystems cover about 40% of the earth's surface (Shaw, Huxman, & Lund, 2005). These ecosystems have taken an important interest in the study of the global carbon cycle (C) due to the complexity in the dynamic functional processes that define their productivity; representing up to 30% of net primary productivity, store about 15% of the organic C content in the soil and control the global CO2 sink potential in terrestrial ecosystems (Schlesinger & Bernhardt, 2013; Poulter et al., 2014; Ahlström et al., 2015), generally are limited by water availability, where precipitation is the main factor that regulates their productivity (Biederman et al., 2017). Rainfall regimes in these ecosystems are seasonal, precipitation events occur as “intermittent pulses” (Ehleringer, 2001; Huxman et al., 2004), making their biogeochemical processes highly sensitive to intensity and frequency of precipitation (Austin et al., 2004; Verduzco et al., 2015), which makes the eco-physiological activity from the vegetation and soil organisms very dynamic and difficult to predict (Vargas et al., 2013; Biederman et al., 2016).
The balance of C and H2O in terrestrial ecosystems is delimited by the exchange of elements and energy, which occurs through the CO2 assimilation during photosynthesis (GPP, gross primary productivity) and the CO2 release into the atmosphere through ecosystem respiration (Reco). This CO2 exchange between the atmosphere and the ecosystem is the net balance of GPP and Reco being known as net ecosystem exchange (NEE), which is a robust approximation of net ecosystem productivity (PNE = -NEE) (Chapin, Matson, & Vitousek, 2011; Grace, Mitchard, & Gloor, 2014).
The exchange of elements and energy between the ecosystem and the atmosphere has been measured by different techniques, but at ecosystem level the most widely used is the eddy covariance technique (EC; Baldocchi, 2008). However, this technique has limitations to represent fluxes from different strata of the ecosystem, since, it assumes a relatively large and homogeneous area of land, it does not distinguish between the different strata of the ecosystem (i.e., understory and soil microbiota). Therefore, the relative contributions to ecosystem fluxes from canopy and understory elements are not widely known (Scott et al., 2003). It has been found that due to the understory conditions such as low wind speed, high heterogeneity and intermittent turbulence, EC measurements are inconsistent. Static chambers are a suitable technique to study the gas exchange dynamic at small scales with a wide spatial variability (Angell, Svejcar, Bates, Saliendra, & Johnson, 2001; Yépez et al., 2005) and present an acceptable correlation with the eddy covariance technique (Angell & Svejcar, 1999; Czóbel et al., 2005; Wohlfahrt, Fenstermaker, & Arnone III, 2008).
Due to the structure of the understory, which consists in a mosaic of heterogeneous patterns, very common in arid and semi-arid areas (Loik, Breshears, Lauenroth, & Belnap, 2004), the use of static chambers is recommended to detect the effects of vegetation and land use change in CO2 and water vapor fluxes (Delgado-Balbuena et al., 2013). Few researches have quantified evapotranspiration and CO2 fluxes at intermediate scales or in specific compartments from ecosystems (Scott et al., 2003). However, it has been observed that in arid and semi-arid ecosystems the understory or lower strata of the ecosystem can contribute with 44% of the net C fluxes (Misson et al., 2007) and 62% of the ET in a mesquite forest (Yépez et al., 2007).
Because it is possible with the use of static gas exchange chambers to quantify these ecosystem fluxes (Arnone & Obrist, 2003; Jasoni, Smith, & Arnone, 2005; Delgado-Balbuena et al., 2013), in this study CO2 and water vapor fluxes were measured in an understory with the presence of Mimosa distachya in a subtropical shrubland from Northwest Mexico, to estimate the relative contribution to the net ecosystem fluxes. We hypothesize that understory contributes with a significant proportion of the total CO2 and water vapor fluxes of the ecosystem, besides that, their relative contribution varies in the soil water availability during the rainy season.
Materials and methods
Study site
The study site is a subtropical shrubland located in the municipality of Rayón in the central part of the State of Sonora (29.74 ° N, -110.54 ° W) at an altitude of ~632 m.s.n.m (Vivoni et al., 2010). The climate of the area is semi-warm arid -BSoh (x') - according to the classification of García (1998). This area has a strong seasonality influenced by the North American Monsoon System that allows an active rainy season during the summer, representing ~70% of the annual rainfall (Villarreal et al., 2016). Historical records report a mean annual temperature of 21.4 ° C with a mean precipitation of 502 mm year-1 during the period 1974-2016 (CICESE, 2018). The site is equipped with an eddy covariance system (Baldocchi et al., 2001; Baldocchi, 2008), which continuously measures the CO2 and water vapor flux from the shrubland (Watts et al., 2007). Soils are shallow (~1 m) and are classified as regosol-yermosol with sandy loam texture (from 0 to 30 cm) and sandy clay (from 30 to 100 cm) (Verduzco et al., 2018).
The vegetation at the site is characterized mainly by microphyll species, with the presence of thorns and the loss of leaves during the dry season (Rzedowski, 2006). It has a two-strata defined by: (1) a tree layer, dominated by Prosopis velutina, Acacia cochliacantha), Parkinsonia praecox and Fouquieria macdougalii consortia with Jatropha cordata; and (2) a shrub layer formed by Ambrosia cordifolia, Encelia farinosa, Lycium berlandieri and a notable presence of Mimosa distachya (Tarin et al., 2014).
Net CO2 exchange (NCE) and evapotranspiration of the shrub layer (ETarb)
For convenient purposes in the present study we use the terms of net CO2 exchange (NCE) and evapotranspiration of the shrub layer (ETarb) to refer to the CO2 exchange and the measured water vapor flux with the static chamber. Meanwhile, net ecosystem exchange (NEE) and evapotranspiration of the ecosystem (ET) are referred to the exchange of CO2 and water vapor flux from the eddy covariance technique (EC).
Gas exchange measurements were made during the summer of 2012, on the days of the year 199, 220-221 and 258-259 during the North American monsoon season. The CO2 and water vapor fluxes were quantified using a static gas exchange static chamber, a method developed and explained in detail in Arnone and Obrist (2003). The chamber is a modified version of the 4.2-meter diameter dome manufactured by Shelter Systems Inc. (Menlo Park, CA, USA). The chamber has a height of 2.0 m, a weight of 30 kg, volume of 16.4 m3 and covers an area of 12.25 m2. Its structure is formed by a moderate semi-transparent and translucent polyethylene (PE) cover that allows passing 90% of the light into the chamber (Arnone & Obrist, 2003). The version used by Arnone and Obrist (2003) was modified by constructing a lower base for greater support and sealing (Figure 1).

Figure 1 Experimental design for gas exchange measurements with a static chamber and a diagram of the measurement, control and storage system.
Four experimental plots with presence of M. distachya and a similar leaf area were selected, which was determined with a ceptometer (Sun Scan SS1, Delta T Devices Ltd., Cambridge, UK) (Table 1). The measurements length were performed to 120 from 140 seconds the chamber was placed on each experimental plot, after each measurement, the chamber was removed and aerated for several minutes before the next measurement in another plot (Hamerlynck et al., 2011). Instantaneous measurements of NCE and ETarb were taken in the experimental plots in four periods of the day (9, 11, 14 and 18 hours).
Table 1 Leaf area index for experimental plots in the understory of the subtropical shrubland.
Plot | Mean | Standard deviation | Standard error |
---|---|---|---|
1 | 0.6 | 0.30 | 0.12 |
2 | 0.6 | 0.32 | 0.11 |
3 | 0.8 | 0.46 | 0.16 |
4 | 0.9 | 0.77 | 0.27 |
Before each measurement, an air mixing system consisting of six 12 cm diameter fans was placed at the center of each plot, four fans were oriented horizontally and two oriented vertically to homogenize the air inside the chamber. The air inside the static chamber was circulated to an infrared gas analyzer (IRGA, LI-840, LI-COR Biosciences, Lincoln, NE, USA) to determine the CO2 and water vapor concentrations (change). For this, a pneumatic pump (GAST, Benton Harbor, MI, USA) was used to circulate the air into IRGA, a flow regulator (Dwyer Instruments, Inc., Michigan City, IN, USA) to control the air at 1 liter per minute and a filter (Balston DFU, Haverhill, MA, USA) to prevent impurities towards the IRGA. Data was collected by the IRGA and was stored in a laptop using the data acquisition software from the LI-840 (Figure 1).
Flux calculation with static chambers
The NCE and ETarb calculation in each experimental plot was obtained through the flux equation for static chambers from Jasoni et al. (2005):
where F is the instantaneous net flux of CO2 (μmol m2 s-1) or H2O (mmol m2 s-1), m is the change ratio of CO2 and H2O concentration with respect to time (i.e.Figure 2), V is the total volume of the static chamber (m3), P is the atmospheric pressure (kPa), R is the ideal gases constant (8.3144 m3 Pa mol-1 K-1), T is the mean air temperature during the measurement (K) and A is the area of the static chamber (m2).
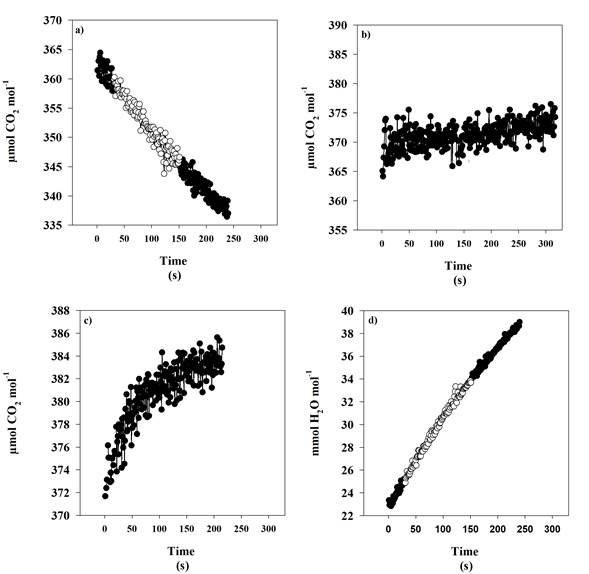
Figure 2 Examples of instantaneous changes in CO2 and water vapor concentrations inside the chamber along a measurement period in an experimental plot with the presence of M. distachya. Panel a) represents CO2 assimilation; b) compensation between assimilation and emission; c) CO2 emission and d) water loss. The white portion is the 120 seconds considered for NCE and ETarb calculation.
During each measurement P was obtained from the IRGA and T with a thermocouple. To obtain the slope (m) of each measurement, the data of gas concentration increments were recorded 30 s after the measurements starting, in order to allow stabilization conditions inside the chamber. In each measurement, instantaneous increases in CO2 and H2O were plotted to determine m, negative slopes represent CO2 assimilation from the ecosystem, while positive slopes are CO2 and water vapor emissions into the atmosphere (Figure 2).
To integrate the CO2 and water vapor fluxes during daytime, the procedure of Potts et al. (2006) was followed, where the instantaneous NCE and ETarb values of each plot were calculated using an adjustment curve and the integration trapezoidal function (SigmaPlot v.12, Systat Software, Inc., Chicago, IL, USA).
Net ecosystem exchange (NEE) and evapotranspiration (ET)
The eddy covariance technique (EC) employs high frequency measurements (10 Hz) of gas concentrations (i.e. H2O, CO2 or CH4), the wind direction in three dimensions allows to estimate the vertical exchange of energy and gases between the ecosystem and the atmosphere based on the turbulence that occurs in the atmosphere (Baldocchi, 2014; Aubinet, Vesala, & Papale, 2012). The flux is defined as the covariance of the vertical fluctuations of the wind speed and the concentration of CO2 and water multiplied by the mean air density in a period of time (Delgado-Balbuena et al., 2019).
The eddy covariance system at the study site consisted of a three-dimensional sonic anemometer (CSAT3, Campbell Scientific, Logan, Utah, USA) and an open-path infrared gas analyzer (LI-7500, LI-COR Biosicences, Lincoln, Nebraska, USA) that perform the measurements at 20 Hz and were located at a height of 6 m above the vegetation. Additionally, meteorological variables measurements were made including; relative humidity and temperature (HMP45D, Vaisala, Helsinki, Finland), precipitation (TR-525USW, Texas Electronics, Dallas, TX, USA) and soil moisture (CS616, Campbell Scientific, Logan, UT, USA). Data derived from the EC system and the meteorological sensors were stored in a datalogger (CR5000, Campbell Scientific, Logan, Utah, USA). Subsequently, data were processed and averaged in half hours to obtain NEE and ET (Verduzco et al., 2018). Previous studies describe in more detail the EC system instrumentation and the meteorological sensors at study site, as well as, the processing and quality control of NEE and ET data (Watts et al., 2007; Vivoni et al., 2010; Méndez-Barroso et al., 2014; Tarin et al., 2014).
To represent the daytime fluxes and to compare with the NCE and ETarb measurements, a mean of seven days of NEE and ET observations were made, that included three days previous to measurements with the static chamber, the day of measurement and three days after.
Results
Environmental conditions
Figure 3 shows the variations of the environmental conditions during the CO2 and water vapor fluxes measurements period in the subtropical shrubland of Rayón, Sonora, Mexico. Temperature (T) varied between 24.48 and 34.79 ° C, while relative humidity (RH) showed an inverse behavior to temperature with values between 77.90 and 21.52%. During the rainy season at the study site 39 precipitation events were recorded, of which 62% were greater than 3 mm and the remaining (39%) were less than 3 mm, with dry periods (intervals between the of precipitation events less than or equal to four days). Similar to RH, soil moisture (SM) responded to precipitation patterns. Soil moisture increased with the presence of rain and decreased when the precipitation pulses ended, the highest value observed of SM was 0.20 m3 m-3 after a precipitation event greater than 70 mm and the lowest was 0.01 m3 m-3.
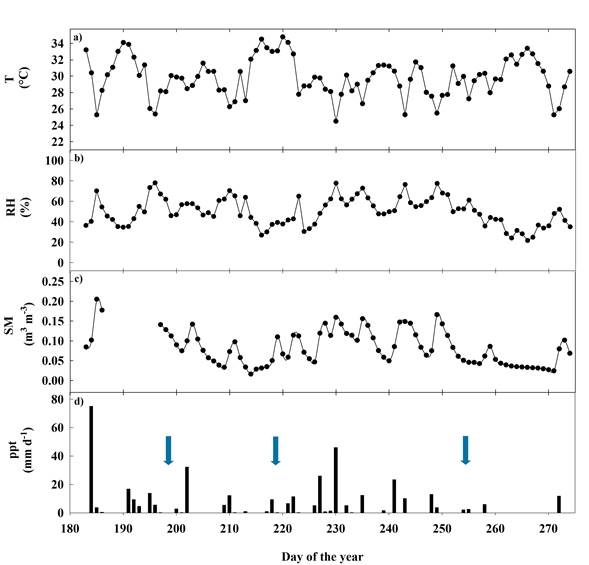
Figure 3 Mean daytime micrometeorology for the period July-September 2012: a) temperature (T), b) relative humidity (RH), c) soil moisture (SM) and d) precipitation (ppt). The arrows indicate the days of the year (199, 220-221 and 258-259) when the NCE and ETarb measurements were made with the static chamber.
On the other hand, the mean value of ETarb at 09:00 hours, during July was 2.40 ± 0.09 mm d-1, while in August showed a value of 1.54 ± 0.10 mm d-1 and September of 0.84 ± 0.10 mm d-1. Maximum ET values were observed between 11:00 and 14:00 hours with values of 2.59 ± 0.16, 1.73 ± 0.09, 2.54 ± 0.07 mm d-1 for July, August and September, respectively, showing minimum values at 18:30 hours (in the range of 0.07 to 0.72 mm d-1).
NCE and water vapor flux at the shrub layer
Figure 4 illustrates the magnitude and variations of NCE and ETarb during a diurnal cycle in summer of 2012. Maximum mean (n = 4) in NCE fluxes were detected at 11:00 hours with values of -2.46 ± 0.54 and -5.08 ± 0.96 µmol m-2 s-1 for August and September, respectively, except for July which showed the highest NCE value at 9:00 with -6.83 ± 1.32 µmol m-2 s-1. During the afternoon, the mean fluxes decreased to -0.35 ± 1.72, -1.02 ± 0.10 and -0.21 ± 0.27 µmol m-2 s-1. Notably, the same variations of NCE with respect to NEE are observed during the daytime periods, coinciding with its maximum CO2 assimilation values at the same times of the day.
Evapotranspiration and net ecosystem exchange
The ecosystem ET and the CO2 fluxes obtained with the EC technique are shown in Figure 4. Negative values of NEE began approximately at 7:00 hours during July and August with ranges between -1.28 and -0.08 µmol m- 2 s-1, while in September the ecosystem begin to show a net CO2 gain at 7:30 with -2.23 µmol m-2 s-1. These values starting to show a continuous and quick increasing until approximately 11:00 hours for the three months, with maximum values of CO2 assimilation of -18.54, -15.14 and -17.12 µmol m-2 s-1, for July, August and September respectively. During the afternoon, the ecosystem responds to the diminution in solar radiation and negative CO2 decreased to minimum values of -0.51, -0.29 and -3.37 µmol m-2 s-1.
Water vapor exchange at the ecosystem level begin at 6:30 hours with solar radiation availability and the increase in temperature. During July mean values were between 1.10 and 17.14 mm d-1; August showed ET ranges between 1.50 and 12.43 mm d-1. The results measured by EC technique indicate that September differs from the previous measured months, the beginning of the water vapor flux dynamic in the ecosystem occurred at 07:00 hours and concluded with the day light cycle at 17:30 hours, showing a range of values between 1.13 and 10.27 mm d-1. Evapotranspiration values did not start near or equal zero, because the measurements were made only with the solar radiation availability.
Dynamics of integrated fluxes of the shrub layer and the ecosystem
The understory with the presence of the legume M. distachya has an important contribution to the CO2 and water vapor fluxes dynamic during daylight hours in the subtropical shrubland. It presented great variability in the total daytime fluxes, where July and September were the months with the highest C gains, with values of -1.3 ± 1.93 g C m-2 d-1 and -1.15.85 ± 0.74 g C m-2 d-1, respectively, suggesting that in this understory during these periods photosynthesis was stronger than respiration. In August, when a dry period occurred, NCE was -0.85 ± 0.73 g C m-2 d-1, lower than the other two months. The ETarb in July was 3.63 ± 0.15mm d-1, while August was 2.71 ± 0.08 mm d-1 and September with 1.59 ± 0.05 mm d-1. Notably, evapotranspiration variations followed the pattern of water availability with the lowest fluxes in September (Figure 5).
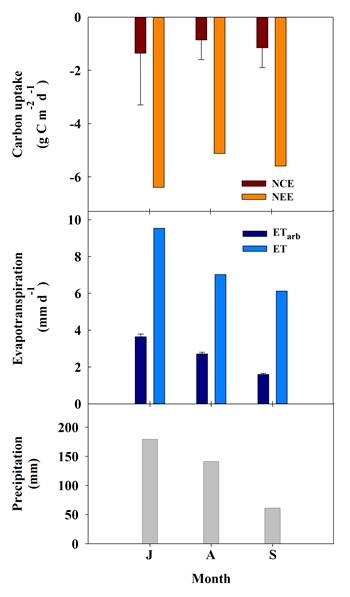
Figure 5 Comparison of NCE and ETarb fluxes and total fluxes from the ecosystem (NEE and ET) during the study period, where J = July, A = August, S = September.
The contribution to the CO2 and water fluxes from the understory was considerable. Its relative contribution to net CO2 ecosystem flux during the studied period was 20% and 35% to water flux. Maximum CO2 gain values were observed during the month of July, both measured by the static chamber and the EC system. On the other hand, NCE and NEE varied according to the availability of moisture. Notably, the variation in ETarb and ET followed the pattern of water availability in the soil, showing the lowest fluxes in September.
Discussion
Dynamics of CO2 and H2O fluxes are more active during the rainy season. The relative contribution of the understory or lower strata of the ecosystem is still unknown. In our study site, there is dominance of the leguminous M. distachya in the understory, thus our hypothesis suggested that this shrub has an important contribution to water and CO2 fluxes magnitude and dynamics in this ecosystem.
There are methodologies that allow the measurement of CO2 and water fluxes and separate these fluxes at ecosystem and understory level, such as static chambers, which are useful tools for measuring CO2 and water vapor fluxes in different strata in ecosystems and experimental plots (Arnone & Obrist, 2003; Yépez et al., 2005).
The area covered by static chambers includes all representative components of the ecosystem, thus eliminating the need to separate flux measurements in shrub spaces (soil vs. grasslands) and the shrub layer, a necessary procedure for small chambers used by Angell and Svejcar (1999) and Angell et al. (2001), in addition to allow replication which facilitates statistical rigor (Yépez et al., 2005).
Moreover, it has been demonstrated that static chamber methods have a consistent relation with fluxes estimated with the EC technique (Oechel et al., 1998; Angell et al., 2001; Wohlfahrt et al., 2008), without losing the representativeness of the ecosystem.
Our results show a dynamic and variable patterns of CO2 and water vapor fluxes over the daytime periods, prevailing a considerable CO2 assimilation values (Figure 4), which represents a relative contribution between 17% and 21% of the net CO2 ecosystem flux (i.e. NEE), while ET of the understory represented between 25% and 39% of the total ET flux in the ecosystem. This response suggests that there was enough moisture in the soil during the monsoon season for M. distachya to remain physiologically active, since photosynthesis during daylight hours was always dominant, despite presenting a major decrease starting at noon, which probably limited its contribution to net CO2 ecosystem flux (Figure 4).
The soil microorganisms activity was probably also influenced by the availability of water in the soil to contribute to gas exchange dynamics.
In our experimental plots, there is an important presence of biological soil crusts, which could contribute to the net CO2 exchange. In the Mojave Desert, Jasoni et al. (2005) attribute that high assimilation rates may have been due to the photosynthesis of the autotrophic soil communities such as biological crusts, where under optimal conditions of water availability and temperature are able to carry out their biological activity and significantly contribute to the biogeochemistry of the ecosystem (Delgado-Baquerizo, Maestre, & Gallardo, 2013).
Most precipitation events in arid and semi-arid ecosystems are rain pulses less than 3 mm (Loik et al., 2004), small rain pulses cause a response from most plants, but large enough to stimulate a response from biological crusts, which are usually from 1 to 2 mm from the soil surface and between the vegetation cover (Austin et al., 2004). Therefore, it would be plausible to consider an important contribution from the biological soil crusts to the C fluxes in the understory. For example, at our site in average presented 3-day intervals between each precipitation event and 58% was greater than 3 mm (Figure 2). Considering that, there was an optimal presence of moisture in the plots of the understory with the presence of M. distachya, an ideal microenvironment was suitable for a high physiological activity of this legume and the biological soil crusts.
We are not aware about studies that provide data from daytime NCE and ET patterns in lower ecosystem strata such as the understory in semi-arid ecosystems. However, the relative contribution of the understory with the presence of M. distachya is similar to previous studies reported in several semi-arid areas (Misson et al., 2007; Yépez, Scott, Cable, & Williams, 2007). Likewise, it is possible to compare our NCE estimates (Figure 4) measured with the static chamber during the rainy season with other shrubland ecosystems. For example, Jasoni et al. (2005) reports daytime assimilation values of -1.5 µmol m-2 s-1 in the Mojave Desert; while Obrist, DeLucia, and Arnone, (2003), and also Prater Obrist, Arnone III, and DeLucia (2006) report values of -3 µmol m-2 s-1 and ranges from 0.2 to 4.3 µmol m-2 s-1, respectively while Angell et al. (2001) shows values between -7 and -10 µmol m-2 s-1 in the Great Basin Desert. In the context of daytime C gains in grasslands and managed crops, it can be mentioned that our estimates are within the ranges of -0.1 and -6 g C m-2 d-1reported by Delgado-Balbuena et al. (2013).
The net CO2 exchange of the subtropical shrubland studied during the monsoon season show similar values with other seasonally dry ecosystem from Mexico (Delgado-Balbuena et al., 2019) and particularly those that belong to the North America monsoon region (Hinojo-Hinojo et al., 2019). In general, C gain values are consistent with other arid and semi-arid ecosystems, since the observed gain of -6.39 g C m-2 d-1 is in the values range reported for the Mojave desert of -1.7 g C m-2 d-1 (Wohlfahrt et al., 2008) and for semi-arid meadows with values between -6 g C m-2 d-1 (Zhao et al., 2006) and -3.9 g C m-2 d-1 (Kato et al., 2004).
Conclusion
The variability CO2 and water vapor fluxes in the ecosystem were influenced by the presence of rainfall during the summer monsoon season. The use of static chambers (with an area of 12.25 m2 and a volume of 16.4 m3) is a useful tool to measure ecosystem gas exchange and allows studying the relative contribution and variability at small scales with wide spatial heterogeneity, without losing the representativeness of the ecosystem. It is important to make distinctions between the different strata present in arid and semi-arid ecosystems, since each one contributes differently to variations in gas exchange with the atmosphere. Comparing our results with EC technique estimates, we found that the understory with the presence of M. distachya, represents an important and significant contribution to the CO2 and water exchange in the ecosystem, since it can contribute up to 20 and 35%, respectively.
The study of the relative contributions of the understory and their dynamics towards CO2 and water vapor allow us to understand the functional dynamics of arid and semi-arid ecosystems.
It is important to understand the influence of precipitation in and CO2 and water vapor fluxes because it is expected that climate change will affect precipitation patterns and therefore, create a response in ecosystem flux dynamics.
Acknowledgments
This work was possible thanks to the financing of the projects “Sistema de monitoreo eco-hidrológico para la adaptabilidad ante el cambio climático en el sur de Sonora”, to the Cuerpo Académico de Ciencias del Agua, PROFAPI-ITSON, “Estimación de ET y sus componentes evaporación y transpiración en la región del Monzón Mexicano”, CONACYT CB-2009-01-132188 and “US-Mexico Border Water & Environmental Sustainability Training” NSF 1130254. MLVT was supported by CONACYT (542178) and the Programa de Fortalecimiento de la Calidad Educativa (SEP-PFCE 2019) for the preparation of the manuscript. We also acknowledge to Marco Antonio González, Lucía Nevešćanin and Margarita Guerra for their support during the field campaigns and Tola Vargas for the company during the writing process of this manuscript.
REFERENCES
Ahlström, A., Raupach, M. R., Schurgers, G., Smith, B., Arneth, A., Jung, M., Reichstein, M., Canadell, J. G., Friedlingstein, P., Jain, A. K., Kato, E., Poulter, B., Sitch, S., Stocker, B. D., Viovy, N., Wang, Y. P., Wiltshire, A., Zaehle, S., & Zeng, N. (2015). The dominant role of semi-arid ecosystems in the trend and variability of the land CO2 sink. Science, 348(6237), 895-899. DOI: 10.1126/science.aaa1668 [ Links ]
Angell, R. F., Svejcar, T., Bates, J., Saliendra, N. Z., & Johnson, D. A. (2001). Bowen ratio and closed chamber carbon dioxide flux measurements over sagebrush steppe vegetation. Agricultural and Forest Meteorology, 108(2), 153-161. DOI: https://doi.org/10.1016/S0168-1923(01)00227-1 [ Links ]
Angell, R., & Svejcar, T. (1999). A chamber design for measuring net CO2 exchange on rangeland. Journal of Range Management, 52(1), 27-31. DOI: 10.2307/4003488 [ Links ]
Arnone III, J. A., & Obrist, D. (2003). A large daylight geodesic dome for quantification of whole-ecosystem CO2 and water vapour fluxes in arid shrublands. Journal of Arid Environments, 55(4), 629-643. DOI: https://doi.org/10.1016/S0140-1963(02)00291-4 [ Links ]
Aubinet, M., Vesala, T., & Papale, D. (Eds.). (2012). Eddy covariance: A practical guide to measurement and data analysis. Springer Science & Business Media. DOI: https://doi.org/10.1007/978-94-007-2351-1 [ Links ]
Austin, A. T., Yahdjian, L., Stark, J. M., Belnap, J., Porporato, A., Norton, U., Ravetta, D. A., & Schaeffer, S. M. (2004). Water pulses and biogeochemical cycles in arid and semiarid ecosystems. Oecologia, 141(2), 221-235. DOI: 10.1007/s00442-004-1519-1 [ Links ]
Baldocchi, D. (2014). Measuring fluxes of trace gases and energy between ecosystems and the atmosphere-the state and future of the eddy covariance method. Global change biology, 20(12), 3600-3609. DOI: 10.1111/gcb.12649 [ Links ]
Baldocchi, D. (2008). ‘Breathing’ of the terrestrial biosphere: Lessons learned from a global network of carbon dioxide flux measurement systems. Australian Journal of Botany, 56(1), 1-26. DOI: 10.1071/BT07151 [ Links ]
Baldocchi, D., Falge, E., Gu, L., Olson, R., Hollinger, D., Running, S., Anthoni, P., Bernhofer, C., Davis. K., Jose-Fuentes, R. E., Goldstein, A., Katul, G., Law, B., Lee. X., Malhi, Y., Meyers, T., Munger. W., Oechel. W., Paw, K. T., Pilegaard, K., Schmid, H. P., Valentini, R., Verma, S., Vesala, T., Wilson, K., & Wofsy, S. (2001). FLUXNET: A new tool to study the temporal and spatial variability of ecosystem-scale carbon dioxide, water vapor, and energy flux densities. Bulletin of the American Meteorological Society, 82(11), 2415-2434. DOI: 10.1175/1520-0477(2001)082<2415:FANTTS>2.3.CO;2 [ Links ]
Biederman, J. A., Scott, R. L., Bell, T. W., Bowling, D. R., Dore, S., Garatuza‐Payán, J., Kolb, T. E., Krishnan, P., Krofcheck, D. J., Litvak, M. E., Maurer, G. E., Meyers, T. P., Oechel, W. C., Papuga, S. A., Ponce‐Campos, G. E., Rodriguez, J. C., Smith, W. K., Vargas, R., Watts, C. J., Yépez, E. A., & Goulden, M. L. (2017). CO2 exchange and evapotranspiration across dryland ecosystems of southwestern North America. Global Change Biology, 23(10), 4204-4221. DOI: 10.1111/gcb.13686 [ Links ]
Biederman, J. A., Scott, R. L., Goulden, M. L., Vargas, R., Litvak, M. E., Kolb, T. E., Yépez, E. A., Oechel, W. C., Blanken, P. D., Bell, T. W., Garatuza‐Payán, J., Maurer, G. E., Dore, S., & Burns, S. P. (2016). Terrestrial carbon balance in a drier world: The effects of water availability in southwestern North America. Global Change Biology, 22(5), 1867-1879. DOI: 10.1111/gcb.13222 [ Links ]
CICESE, Centro de Investigación Científica y de Educación Superior de Ensenada. (2018). Base de Datos Climatológica Nacional (Sistema CLICOM). Recuperado de http://clicom-mex.cicese.mx/mapa.html [ Links ]
Chapin III, F. S., Matson, P. A., & Vitousek, P. (2011). Principles of terrestrial ecosystem ecology. New York, USA: Springer. DOI: 10.1007/978-1-4419-9504-9 [ Links ]
Czóbel, S., Fóti, S., Balogh, J., Nagy, Z., Bartha, S., & Tuba, Z. (2005). Chamber series and space-scale analysis of CO2 gas-exchange in grassland vegetation: A novel approach. Photosynthetica, 43(2), 267-272. DOI:10.1007/s11099-005-0043-9. [ Links ]
Delgado-Balbuena, J., Yépez, E. A., Paz-Pellat, P., Ángeles-Pérez, G., Aguirre-Gutiérrez, C., Alvarado-Barrientos, M. S., Arredondo, T., Ayala-Niño, F., Bullock, S. H., Castellanos, A. E., Cueva, A., Figueroa-Espinoza, B., Garatuza-Payán, J., González- del-Castillo, E., González-Sosa, E., Guevara-Escobar, A., Hinojo-Hinojo, C., Kyaw-Tha, P. U., Lizárraga-Celaya, C., Maya-Delgado, Y., Oechel, W., Pérez-Ruiz, E. R., Quesada-Avendaño, M., Robles- Zazueta, C. A., Rodríguez, J. C., Rojas-Robles, N. E., Tarin- Terrazas, T., Troyo-Diéguez, T., Uuh-Sonda, J., Vargas-Terminel, M. L., Vargas, R., Vega-Puga, M. G., Verduzco, V. S., Vivoni, E. R., & Watts, C. J. (2019). Flujos verticales de carbono en ecosistemas terrestres. En: Paz-Pellat, F., Hernández-Ayón, J. M., Sosa-Ávalos, R., & Velázquez-Rodríguez, A. S. (eds.). Estado del ciclo del carbono: agenda azul y verde. (716 p.). Texcoco, México: Programa Mexicano del Carbono. [ Links ]
Delgado-Balbuena, J., Arredondo, J. T., Loescher, H. W., Huber-Sannwald, E., Chavez-Aguilar, G., Luna-Luna, M., & Barretero-Hernandez, R. (2013). Differences in plant cover and species composition of semiarid grassland communities of Central Mexico and its effects on net ecosystem exchange. Biogeosciences Discussions, 9(106), 17099-17137. DOI: 10.5194/bg-10-4673-2013 [ Links ]
Delgado-Baquerizo, M., Maestre, F. T., & Gallardo, A. (2013). Biological soil crusts increase the resistance of soil nitrogen dynamics to changes in temperatures in a semi-arid ecosystem. Plant and Soil, 366(1-2), 35-47. DOI: 10.1007/s11104-012-1404-3 [ Links ]
Ehleringer, J. R. (2001). Productivity of deserts. In: Terrestrial Global Productivity (pp. 345-362). DOI: https://doi.org/10.1016/B978-012505290-0/50016-8 [ Links ]
García, E. (1998). Climas (Clasificación de Köppen, modificada por García) Escala 1: 1 000 000. Ciudad de México, México: Comisión Nacional para el Conocimiento y Uso de la Biodiversidad. [ Links ]
Grace, J., Mitchard, E., & Gloor, E. (2014). Perturbations in the carbon budget of the tropics. Global Change Biology, 20(10), 3238-3255. DOI: 10.1111/gcb.12600 [ Links ]
Hamerlynck, E. P., Scott, R. L., Moran, M. S., Schwander, A. M., Connor, E., & Huxman, T. E. (2011). Inter-and under-canopy soil water, leaf-level and whole-plant gas exchange dynamics of a semi-arid perennial C4 grass. Oecologia, 165(1), 17-29. DOI: https://doi.org/10.1007/s00442-010-1757-3 [ Links ]
Hinojo-Hinojo, C., Castellanos, A. E., Huxman, T., Rodríguez, J. C., Vargas, R., Romo-León, J. R., & Biederman, J. A. (2019). Native shrubland and managed buffelgrass savanna in drylands: Implications for ecosystem carbon and water fluxes. Agricultural and Forest Meteorology, 268, 269-278. DOI: https://doi.org/10.1016/j.agrformet.2019.01.030 [ Links ]
Huxman, T. E., Cable, J. M., Ignace, D. D., Eilts, J. A., English, N. B., Weltzin, J., & Williams, D. G. (2004). Response of net ecosystem gas exchange to a simulated precipitation pulse in a semi-arid grassland: the role of native versus non-native grasses and soil texture. Oecologia, 141(2), 295-305. DOI: 10.1007/s00442-003-1389-y [ Links ]
Jasoni, R. L., Smith, S. D., & Arnone III, J. A. (2005). Net ecosystem CO2 exchange in Mojave Desert shrublands during the eighth year of exposure to elevated CO2. Global Change Biology, 11(5), 749-756. DOI: 10.1111/j.1365-2486.2005.00948.x [ Links ]
Kato, T., Tang, Y., Gu, S., Cui, X., Hirota, M., Du, M., Li, Y., Zhao, X., & Oikawa, T. (2004). Carbon dioxide exchange between the atmosphere and an alpine meadow ecosystem on the Qinghai-Tibetan Plateau, China. Agricultural and Forest Meteorology, 124(1-2), 121-134. DOI: https://doi.org/10.1016/j.agrformet.2003.12.008 [ Links ]
Loik, M. E., Breshears, D. D., Lauenroth, W. K., & Belnap, J. (2004). A multi-scale perspective of water pulses in dryland ecosystems: Climatology and ecohydrology of the western USA. Oecologia, 141(2), 269-281. DOI: 10.1007/s00442-004-1570-y [ Links ]
Méndez‐Barroso, L. A., Vivoni, E. R., Robles‐Morua, A., Mascaro, G., Yépez, E. A., Rodríguez, J. C., Watts, C. J., Garatuza‐Payán, J., & Saíz‐Hernández, J. A. (2014). A modeling approach reveals differences in evapotranspiration and its partitioning in two semiarid ecosystems in Northwest Mexico. Water Resources Research, 50(4), 3229-3252. DOI: 10.1002/2013WR014838 [ Links ]
Misson, L., Baldocchi, D. D., Black, T. A., Blanken, P. D., Brunet, Y., Yuste, J. C., Dorsey, J. R., Falk, M., Granier, A., & Irvine, M. R. (2007). Partitioning forest carbon fluxes with overstory and understory eddy-covariance measurements: A synthesis based on FLUXNET data. Agricultural and Forest Meteorology, 144(1-2), 14-31. DOI: 10.1016/j.agrformet.2007.01.006 [ Links ]
Obrist, D., DeLucia, E. H., & Arnone, J. A. (2003). Consequences of wildfire on ecosystem CO2 and water vapour fluxes in the Great Basin. Global Change Biology, 9(4), 563-574. DOI:https://doi.org/10.1046/j.1365-2486.2003.00600.x [ Links ]
Oechel, W. C., Vourlitis, G. L., Brooks, S., Crawford, T. L., & Dumas, E. (1998). Intercomparison among chamber, tower, and aircraft net CO2 and energy fluxes measured during the Arctic System Science Land‐Atmosphere‐Ice Interactions (ARCSS‐LAII) Flux Study.Journal of Geophysical Research: Atmospheres, 103(D22), 28993-29003. DOI: https://doi.org/10.1029/1998JD200015 [ Links ]
Potts, D. L., Huxman, T. E., Cable, J. M., English, N. B., Ignace, D. D., Eilts, J. A., & Williams, D. G. (2006). Antecedent moisture and seasonal precipitation influence the response of canopy‐scale carbon and water exchange to rainfall pulses in a semi‐arid grassland. New Phytologist, 170(4), 849-860. DOI: 10.1111/j.1469-8137.2006.01732.x [ Links ]
Poulter, B., Frank, D., Ciais, P., Myneni, R. B., Andela, N., Bi, J., Broquet, G., Canadell, J. G., Chevallier, F., Liu, Y. Y., Running, S. W., Sitch, S., & Van der Werf, G. R. (2014). Contribution of semi-arid ecosystems to interannual variability of the global carbon cycle. Nature, 509(7502), 600-603. DOI: 10.1038/nature13376 [ Links ]
Prater, M. R., Obrist, D., Arnone III, J. A., & DeLucia, E. H. (2006). Net carbon exchange and evapotranspiration in postfire and intact sagebrush communities in the Great Basin. Oecologia, 146(4), 595-607. DOI: 10.1007/s00442-005-0231-0 [ Links ]
Rzedowski, J. (2006). Vegetación de México (1a ed.). México, DF, México: Comisión Nacional para el Conocimiento y Uso de la Biodiversidad. Recuperado de https://www.biodiversidad.gob.mx/publicaciones/librosDig/pdf/VegetacionMx_Cont.pdf [ Links ]
Schlesinger, W. H., & Bernhardt, E. S. (2013). Biogeochemistry: An analysis of global change (3rd ed.). Academic Press. DOI: 10.1016/C2010-0-66291-2 [ Links ]
Scott, R. L., Watts, C., Payán, J. G., Edwards, E., Goodrich, D. C., Williams, D., & Shuttleworth, W. J. (2003). The understory and overstory partitioning of energy and water fluxes in an open canopy, semiarid woodland. Agricultural and Forest Meteorology, 114(3-4), 127-139. DOI: https://doi.org/10.1016/S0168-1923(02)00197-1 [ Links ]
Shaw, M. R., Huxman, T. E., & Lund, C. P. (2005). Modern and Future Semi-Arid and Arid Ecosystems. In: Baldwin, I. T., Caldwell, M. M., Heldmaier, G., Jackson, R. B., Lange, O. L., Mooney, H. A., Schulze, E. D., Sommer, U., Ehleringer, J. R. M., Dearing, D., & Cerling, T. E. (eds.). A history of atmospheric CO 2 and its effects on plants, animals, and ecosystems. Ecological studies (analysis and synthesis) (pp. 415-440). New York, USA: Springer . DOI: https://doi.org/10.1007/0-387-27048-5_19 [ Links ]
Tarin, T., Yépez, E. A., Garatuza-Payán, J., Watts, C. J., Rodríguez, J. C., Vivoni, E. R., & Méndez-Barroso, L. A. (2014). Partición de la evapotranspiración usando isótopos estables en estudios ecohidrológicos. Tecnología y ciencias del agua, 5(3), 97-114. [ Links ]
Vargas, R., Sonnentag, O., Abramowitz, G., Carrara, A., Chen, J. M., Ciais, P., Correia, A., Keenan, T. F., Kobayashi, H., Ourcival, J.-M., Papale, D., Pearson, D., Pereira, J. S., Piao, S., Rambal, S., & Baldocchi, D. D. (2013). Drought influences the accuracy of simulated ecosystem fluxes: A model-data meta-analysis for Mediterranean oak woodlands. Ecosystems, 16(5), 749-764. DOI: 10.1007/s10021-013-9648-1 [ Links ]
Verduzco, V. S., Garatuza-Payán, J., Yépez, E. A., Watts, C. J., Rodríguez, J. C., Robles‐Morua, A., & Vivoni, E. R. (2015). Variations of net ecosystem production due to seasonal precipitation differences in a tropical dry forest of northwest Mexico. Journal of Geophysical Research: Biogeosciences, 120(10), 2081-2094. DOI: 10.1002/2015JG003119 [ Links ]
Verduzco, V. S., Vivoni, E. R., Yépez, E. A., Rodríguez, J. C., Watts, C. J., Tarin, T., Garatuza‐Payán, J., Robles‐Morua, A., & Ivanov, V. Y. (2018). Climate change impacts on net ecosystem productivity in a subtropical shrubland of Northwestern Mexico. Journal of Geophysical Research: Biogeosciences, 123(2), 688-711. DOI: 10.1002/2017JG004361 [ Links ]
Villarreal, S., Vargas, R., Yépez, E. A., Acosta, J. S., Castro, A., Escoto‐Rodriguez, M., Lopez, E., Martínez‐Osuna, J., Rodriguez, J. C., Smith, S. V., Vivoni, E. R., & Watts, C. J. (2016). Contrasting precipitation seasonality influences evapotranspiration dynamics in water‐limited shrublands. Journal of Geophysical Research: Biogeosciences, 121(2), 494-508. DOI: 10.1002/2015JG003169 [ Links ]
Vivoni, E. R., Watts, C. J., Rodríguez, J. C., Garatuza-Payán, J., Méndez-Barroso, L. A., & Saiz-Hernández, J. A. (2010). Improved land-atmosphere relations through distributed footprint sampling in a subtropical scrubland during the North American monsoon. Journal of Arid Environments, 74(5), 579-584. DOI: 10.1016/j.jaridenv.2009.09.031 [ Links ]
Watts, C. J., Scott, R. L., Garatuza-Payán, J., Rodríguez, J. C., Prueger, J. H., Kustas, W. P., & Douglas, M. (2007). Changes in vegetation condition and surface fluxes during NAME 2004. Journal of Climate, 20(9), 1810-1820. DOI: 10.1175/JCLI4088.1 [ Links ]
Wohlfahrt, G., Fenstermaker, L. F., & Arnone III, J. A. (2008). Large annual net ecosystem CO2 uptake of a Mojave Desert ecosystem.Global Change Biology, 14(7), 1475-1487. DOI:10.1111/j.1365-2486.2008.01593.x [ Links ]
Yépez, E. A., Huxman, T. E., Ignace, D. D., English, N. B., Weltzin, J. F., Castellanos, A. E., & Williams, D. G. (2005). Dynamics of transpiration and evaporation following a moisture pulse in semiarid grassland: A chamber-based isotope method for partitioning flux components. Agricultural and Forest Meteorology, 132(3-4), 359-376. DOI: https://doi.org/10.1016/j.agrformet.2005.09.006 [ Links ]
Yépez, E. A., Scott, R. L., Cable, W. L., & Williams, D. G. (2007). Intraseasonal variation in water and carbon dioxide flux components in a semiarid riparian woodland.Ecosystems, 10(7), 1100-1115. DOI: 10.1007/s10021-007-9079-y [ Links ]
Zhao, L., Li, Y., Xu, S., Zhou, H., Gu, S., Yu, G., & Zhao, X. (2006). Diurnal, seasonal and annual variation in net ecosystem CO2 exchange of an alpine shrubland on Qinghai‐Tibetan plateau.Global Change Biology, 12(10), 1940-19. DOI: 10.1111/j.1365-2486.2006.01197.x [ Links ]
Received: August 29, 2019; Accepted: January 22, 2020