Introduction
Eptesicus fuscus is a medium-sized bat that exhibits sexual dimorphism, with females being slightly larger than males (Kurta and Baker 1990). This species is widely distributed from North America to northern South America and some Caribbean islands (Kurta and Baker 1990). This is the largest neotropical Eptesicus, and currently, there are 11 subspecies recognized with parapatric or allopatric distributions (Figure 1), which are differentiated based mainly on size and fur coloration (Burnett 1983a; Hoffman and Genoways 2008). However, in North America, subspecific delimitation of E. fuscus is problematic due to multiple contact zones among the subspecies (Hoffman and Genoways 2008).
Eptesicus fuscus miradorensis is the only documented subspecies of E. fuscus in the continental Neotropics (Davis and Gardner 2008). This subspecies is found in eastern and southern México throughout high elevations in Central America (Davis and Gardner 2008; Turmelle et al. 2011) till the Andes of Colombia, Venezuela (Davis and Gardner 2008), and southernmost records in Ecuador (Lönnberg 1921; Arguero and Albuja 2012). The subspecies occurs in an elevational range from 900 to 3,100 m, but mostly over 1,500 m (Davis and Gardner 2008; Solari et al. 2013); however, the climatic factors that potentially constrain the distribution of the species to high elevations have not been assessed.
Despite being historically and currently considered a Neotropical subspecies of E. fuscus (Moratelli et al. 2019; Burgin et al. 2020), mitochondrial DNA analyses suggested that samples of specimens from México and Venezuela attributable to E. f. miradorensis were significantly differentiated from most other populations of E. fuscus (Turmelle et al. 2011). Similarly, E. f. miradorensis specimens have been recovered as a monophyletic group (Yi and Latch 2022a) or as a weakly diverged lineage based on ultra-conserved elements - UCE (Yi and Latch 2022b).
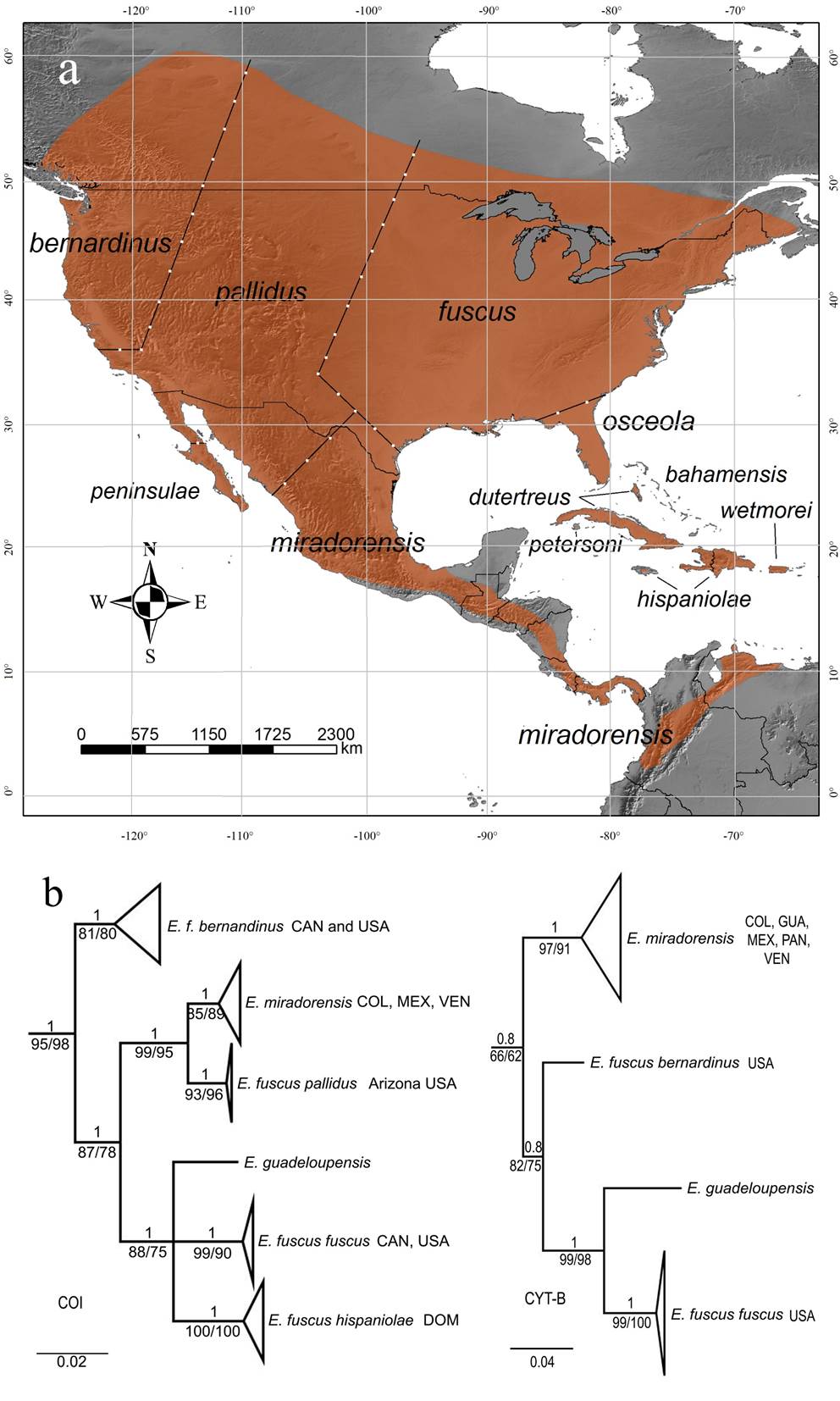
Figure 1 a. Map of the distribution of the currently recognized subspecies of Eptesicus fuscus. b. Bayesian gene trees of Cyt-b and COI of the E. fuscus complex. Upper values of branches show the posterior probability of the Bayesian inference. Values below the branches indicate the maximum likelihood inference's nonparametric (SH-aLRT) and ultrafast (UFBoot) bootstrap values. Country abbreviations. COL: Colombia, CAN: Canada, DOM: Dominican Republic, GUA: Guatemala, PAN: Panama, USA: United States, VEN: Venezuela.
Along its range, E. f. miradorensis can be differentiated from other Neotropical congenerics by its larger size (forearm length > 48 mm; greatest length of the skull generally > 19 mm, and maxillary toothrow length 7 mm or longer; Davis and Gardner 2008), and its strong association with high elevations. Morphometric analyses of the wing and skull characters showed that males and females of E. f. miradorensis were correctly classified using discriminant functions in 90 % of cases compared with other subspecies of E. fuscus (Burnett 1983a). In addition, a clinal increase in wing and skull size from México to Honduras has been suggested, with a slight reversal in this trend in populations from Costa Rica/Panama (Burnett 1983a). In theory, forearm size decreases, but skull size increases into South America. However, this needed to be adequately tested due to the small sample size from South America included in the analyses of Burnett (1983a).
In general, and despite its wide distribution in the Neotropics, little is known about this taxon, and it is considered a relatively rare bat (Davis and Gardner 2008). We aim to assess the validity of E. f. miradorensis as a distinct species using mitochondrial genetic information and contribute to the knowledge of the species by presenting novel information and morphological data from specimens captured in the field and deposited in museum collections. We also update the geographic distribution of E. f. miradorensis along its range based on historical and new records. We predict its potential distribution to estimate the areas where the species might be found and explore environmental factors that influence its association with mountain ecosystems.
Materials and methods
Molecular analyses and systematics. To evaluate the taxonomic status of E. f. miradorensis, we used four sequences of Cytochrome b (Cytb-b) and three sequences of the mitochondrial gene Cytochrome Oxidase I (COI) of E. fuscus miradorensis from Yi and Latch (2022b) kindly provided by the first author and one sequence of Cyt-b from Colombia amplified by us in Ramírez-Chaves et al. (2021). The sequences comprise different localities along the complete distribution of the subspecies. To complete our dataset, we gathered sequences of the two genes of additional subspecies of E. fuscus from GenBank, including six sequences of Cyt-b and 134 sequences of COI. The list of the used sequences is shown in the Appendix 1.
We aligned all the sequences of each gene using the default parameters of the Clustal W algorithm in BioEdit 7.2.6 software (Hall 1999). We assessed the best-fit evolutionary model per gene using ModelFinder (Kalyaanamoorthy et al. 2017) implemented in IQ-TREE software (Nguyen et al. 2015) specifying the TPM2uf+I+G4 model for Cyt-b gene and HKY+G4 for COI gene. Then we conducted a maximum likelihood analysis with IQ-TREE (Nguyen et al. 2015) using 20,000 replicates to find the best tree. We used nonparametric SH-aLRT and ultrafast-bootstrap (UFBoot; Hoang et al. 2018) values as the branch support measure. We conducted Bayesian analysis in MrBayes 3.2.6 (Ronquist et al. 2012) and ran two independent replicates of the Metropolis coupled chain Monte Carlo analysis for 10,000,000 generations with trees sampled every 1,000 generations. Convergence was inspected in the program Tracer 1.6 (Rambaut et al. 2014) by plotting likelihood values per generation. We discarded 25 % of the samples in each run as burn-in and combined the remaining samples to estimate tree topology, the mean likelihood, and posterior probabilities. Finally, we used the program MEGA 7 (Kumar et al. 2015) to calculate average uncorrected p-distances with partial deletion, allowing less than 5 % of gaps, missing data, and ambiguous bases; resulting in two new matrices of 516 bp for COI and 649 bp for Cyt-b for distance calculations. The percentage of genetic divergence was computed as genetic distance x 100.
To clarify the systematics of E. f. miradorensis, we provided a list of synonyms and examined the holotype of Eptesicus fuscus pelliceus (BMNH 98.7.1.28), considered a junior synonym of E. f. miradorensis.
Morphology and morphometry. To obtain morphometric and morphological data of E. f. miradorensis, we reviewed specimens of the genus Eptesicus deposited in the following institutions: Australia: The Queensland Museum (QM); United States: the American Museum of Natural History (AMNH); United Kingdom: the British Museum of Natural History (BMNH); Colombia: the Colección Zoológica, Universidad del Tolima (CZUT), Instituto Alexander von Humboldt (IAvH); Instituto de Ciencias Naturales, Universidad Nacional de Colombia (ICN); Museo de Historia Natural Universidad de La Salle (MLS); Museo de Historia Natural Universidad del Cauca (MHNUC); Museo de Historia Natural de la Universidad de Caldas (MHN-UCa); Museo de la Universidad de Antioquia (MUA, currently Colección Teriológica Universidad de Antioquia CTUA), and Universidad del Valle (UV); México: Colección Nacional de Mamíferos, Universidad Nacional Autónoma de México (CNMA). The list of revised specimens is presented in the Supplementary material.
Of each specimen, 14 skull and external measurements were taken using digital calipers to the nearest 0.1 mm: hind foot length (HF), forearm length (FA), third metacarpal (IIIMT), fourth metacarpal (IVMT), fifth metacarpal (VMT), the greatest length of the skull excluding incisors (GLS), condyle-basal length (CBL), zygomatic breadth (ZB), mastoid breadth (BM), braincase breadth (BBC), breadth across postorbital constriction (POC), length of maxillary toothrow (CM3), breadth across molars (M3M3), and length of the mandible (ML). Total body length (TL), tail length (TV), ear length (EAR), and weight (W) were taken from the labels.
We illustrated the skeleton anatomy of E. miradorensis based on micro-Computed Tomography CT-scans of one specimen from México (QM JM 6365) and the direct inspection of three skeletons of specimens from Colombia (MHN-UCa 2022, 3204, 3208). We also described the baculum of one adult specimen from México (QM JM 6365) using CT-scans and obtained three measurements: baculum length, base width, and shaft width. We also compared the bacular morphology with information available in the literature for Eptesicus taxa (Hamilton 1949; Brown et al. 1971).
All the specimens were identified following the characters proposed in Davis and Gardner (2008): large size (forearm length > 48 mm or longer; greatest length of the skull generally > 19 mm, and maxillary toothrow length 7 mm or longer). We present descriptive statistics of the skull and external measurements for males and females separately for each country. Due to the number of available specimens, sexual dimorphism in wing and skull characters of populations from South America (Colombia-Venezuela) and México was explored using a Mann-Whitney U-test. We also performed a Principal component analysis (PCA) of combined male and female specimens to explore the morphological variation gap and to test the possible directions of the variance associated with wing and skull size from México south to Central and South America. For these analyses, we used the statistical software PAST version 4 (Hammer et al. 2001).
Distribution. To update the distribution, we obtained geographic information of the specimens reviewed and included records from other sources such as literature (Davis and Gardner 2008; Turmelle et al. 2011; Arguero and Albuja 2012) and online databases such as the Global Biodiversity Information Facility (GBIF). The list of the used records is presented in the Supplementary material. We reviewed and curated the database by applying verification procedures and removing duplicate records, records without complete geographical information (geographic region, latitude, longitude, locality, voucher number), or taxonomic uncertainty (Chapman 2005). Spatial filtering of at least a 10 km distance was applied to reduce spatial bias and auto-correlation (Boria et al. 2014) using the R package spThin (Aiello-Lammens et al. 2015; 1,000 repetitions). With these records, we also performed a Species Distribution Model (SDM) using Maxent version 3.4.0 (Phillips et al. 2017) to create a prediction of suitability across the study area (Phillips et al. 2006, 2017; Phillips and Dudík 2008) and to explore barriers that may be constrained the distributional potential of the species. We followed the proposal of Soberón (2010) to define our study area by selecting ecoregions (Olson et al. 2001) in which the species have been documented. We added a 10 km buffer around the ecoregions polygon to ensure that Maxent selects the bioclimatic data from ‘background’ pixels from a region in which known records are more likely to form a representative sample of the climatic conditions suitable for the species and within which absences are meaningful (Barve et al. 2011). Initially, we considered 55 environmental predictors, of which 19 represent annual trends (e. g., mean annual temperature, annual precipitation), seasonality (e. g., annual range in temperature and precipitation), and extreme or limiting environmental factors (e. g., temperature of the coldest and warmest month, and precipitation of the wet and dry quarters), two monthly precipitation (mm), 12 solar radiation (kJ m-2 day-1), and 12 wind speed (m s-1) at 30 s (~ 1 km2) resolution from WorldClim Version2 (Fick and Hijmans 2017). These variables reflect the information of temperature, rainfall, heat and light, and other radiation given off by the sun and air moving. We consider these climatic predictors because some impose physiological constraints on bats (Velazco et al. 2018). We remove highly correlated and redundant variables considering a VIF < 10 (Variance Inflation Factor; Montgomery and Peck 1992). We used ENMeval 2.0 package (Kass et al. 2021) to automate the model analysis for the species considering three feature class combinations (lq, lqh, h) and five regularization multipliers settings (one by one to 5). Candidate model performance was evaluated based on the significance of the partial receiver operating characteristic (partial ROC), AUC difference (low value), and omission rate at ten percentiles (OR 10p; Cobos et al. 2019; Kass et al. 2021). The Cloglog output format was used to describe the environmental suitability of the species (Phillips and Dudík 2008), and it was reclassified to obtain a binary map using the 10-percentile threshold value. We evaluated the correlation between altitude and suitability using a Pearson test to assess if the species has some altitudinal preferences. Finally, we represented the latitude, longitude, suitability values, and altitude in a plot using the ggplot 2.0 package (Wickham 2020) to show the spatial tendencies of the altitude and suitability. The statistical analysis was performed in the R program (R Core Team 2022).
Results
Molecular analyses and systematics. The alignments of both genes were unequivocal and without internal stop codons. The Cyt-b alignment consisted of 1,016 bp, of which 190 bp were parsimony-informative sites, and 678 were invariable sites. The COI alignment comprised 657 bp, 151 bp were parsimony-informative sites, and 441 were invariable sites.
The trees of the two mitochondrial genes (Cyt-b and COI) recovered E. f. miradorensis as a monophyletic group, including sequences from a comprehensive geographic coverage of the species. The COI gene recovered E. f. miradorensis as a monophyletic clade, including sequences from Colombia, México, and Venezuela, with strong support in the Bayesian analysis (PP = 1) and non-parametric branch support of the maximum likelihood analysis (SH-aLRT = 85) but not supported in ultrafast bootstrap (UFBoot = 89). This clade is sister to a well-supported clade of E. fuscus pallidus from southeast USA (PP = 1; SH-aLRT = 93; UFBoot = 96), forming a pair of strongly supported sister clades (PP = 1; SH-aLRT = 99; UFBoot = 95). Other subspecies such as the nominal E. f. fuscus from central and west Canada and the west USA, and E. f. hispaniolae from the Dominican Republic, formed an unresolved group with E. guadeloupensis. Finally, E. f. bernardinus from western Canada and the north-western USA appeared as a sister group to the other subspecies of E. fuscus included and E. guadeloupensis (Figure 1). Similarly, the Cyt-b tree recovered E. f. miradorensis as a monophyletic clade with high support in Bayesian inferences (PP = 1) and non-parametric branch support of the maximum likelihood analysis (SH-aLRT = 97) but not supported in ultrafast bootstrap (UFBoot = 91); additionally, its early spit from the remain taxa (Eptesicus guadeloupensis, E. f. fuscus, and E. f. bernardinus) is not well supported (PP = 0.8, SH-aLRT = 66, UFBoot = 62; Figure 1).
The genetic distances are considerably greater comparing E. f. miradorensis with other subspecies of E. fuscus. For the COI gene, the genetic distances varied between 3.75 % (E. f. miradorensis and E. f. pallidus) and 10.27 % (E. f. miradorensis and E. f. hispaniolae), while the distance within sequences of E. f. miradorensis was 0.90 %. For the Cyt-b gene, the genetic distances varied between 6.82 % (E. f. miradorensis and E. f. bernardinus) and 9.81 % (E. f. miradorensis and E. guadeloupensis), while the distance within sequences of E. f. miradorensis was 2.67 % (Table 1).
Table 1 Uncorrected p-distances in % for the Cyt-b (above diagonal) and COI (below diagonal) genes. Bold values in the diagonal represent the distances within taxa where the first values are from the Cyt-b gene and the second values correspond to the COI gene.
1 | 2 | 3 | 4 | 5 | 6 | |
1. E. guadeloupensis | NA/NA | 9.81 | 6.66 | 7.86 | ||
2. E. miradorensis | 8.66 | 2.67/0.90 | 6.83 | 6.82 | ||
3. E. f. fuscus | 6.39 | 9.21 | 0.39/0.30 | 6.82 | ||
4. E. f. pallidus | 8.27 | 3.75 | 9.05 | NA/0.26 | ||
5. E. f. bernardinus | 8.29 | 7.32 | 7.09 | 7.92 | NA/0.63 | |
6. E. f. hispaniolae | 6.59 | 10.27 | 6.93 | 9.24 | 8.32 | NA/0.78 |
Taxonomic remarks. Based on the information that recovered E. f. miradorensis as monophyletic groups in two mitochondrial markers, and considering the high genetic distances compared with other subspecies that are over the values proposed in the genetic concept of species (> 3 %, Bradley and Baker 2001) we suggest that E. f. miradorensis should be elevated to species level:
Systematics
Order Chiroptera
Family Vespertilionidae
Genus EptesicusRafinesque, 1820
Eptesicus miradorensis (H. Allen, 1866)
S[cotophilus]. miradorensis H. Allen, 1866:287; type locality “Mirador,” Veracruz, México.
[Vespertilio (Eptesicus) fuscus] miradorensis: Trouessart, 1904:77; name combination.
Eptesicus fuscus miradorensis: Miller, 1912:62; name combination.
Eptesicus fuscus pelliceus O. Thomas, 1920b:361; type locality “La Culata,” Merida, Venezuela.
E[ptesicus]. s[erotinus]. miradorensis: Koopman, 1994:120; name combination
Eptesicus brasiliensis: Niceforo Maria [2004]:225; in part.
LSID: urn:lsid:zoobank.org:act:39C7676F-8E25-4F4C-9B92-491AB516E427.
Holotype: Not designated. The description was based on a fluid-preserved female (National Museum of Natural History - USNM 5411) collected by Dr. C. Sartorius in Mirador, Veracruz, México (Davis 1966). According to Lyon and Osgood (1909), the specimen could not be located.
Emended diagnosis: Eptesicus miradorensis is the largest species of the genus with continental distribution in America (forearm: 48.54 to 51.13 mm). The skull is robust (greatest length of the skull: 19.79 to 20.59 mm; condyle-canine length: 17.7 to 18.47 mm) and presents well developed sagittal and lambdoidal crests, with a triangular appearance in caudal view. The dorsal fur is long (9.0 to 11.0 mm), lustrous, smooth, and shiny, with a brown color. The dorsal coloration is brown to golden yellow, dark to light, and bright, with two bands, the lower band being darker. The ventral coloration is lighter than the dorsal, with two bands, the lower band being darker. Both ventral coloration and dorsal have a mottled appearance. The wing and uropatagium membranes are dark. The ears are large (mean 17.5 mm) with the same coloring of the membranes. The tragus is elongated, reaching about 40 % of the length of the ears. The face is bare, and the skull is not flared and domed (Figure 2).
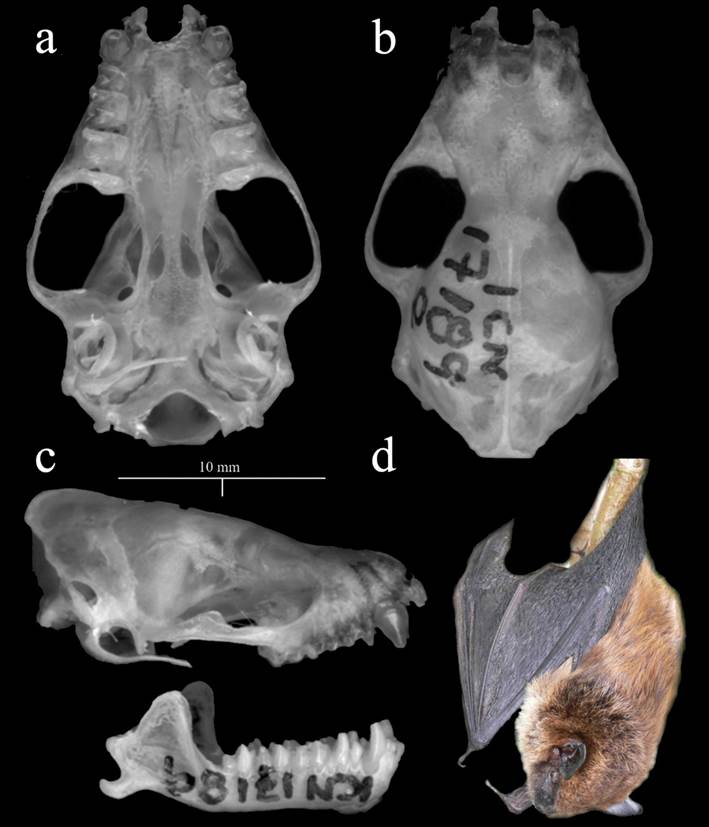
Figure 2 Details of the skull (ICN 17189; female from Department of Santander, Colombia) of E. miradorensis. a. Ventral view. b. Dorsal view. c. Lateral view. d. Alive specimen from Serranía del Perijá, Colombia (ICN uncatalogued) shows long brownish hair and a dark, naked face.
Skeleton and baculum morphology. All specimens analyzed have seven cervical vertebrae, 11 thoracic, six lumbar, the sacral vertebrae fused into a single bone (the sacrum), and eight caudal vertebrae. The number of ribs is 10 (Figure 3). The baculum of one specimen from México (QM JM 6365) is small (length 0.83 mm, base width 0.54 mm, shaft width 0.34 mm), slightly convex dorsally, and slightly concave ventrally (Figure 3). The baculum morphology of E. fuscus from North America (without specific locality but likely from the USA; see Hamilton, 1949) is similar in size (0.8 mm length), and general morphology to the one observed in E. miradorensis described here.
Assessment of morphometric variation of E. miradorensis. We reviewed 85 specimens from Colombia, Guatemala, México, Panamá, and Venezuela. All individuals match the diagnostic characters of E. miradorensis: large size (FA 48.0 to 54.0 mm, GLS ~ 18.5 to 21.2 mm, weight 10 to 19.6 g), and long brownish hair (8 to 12 mm). Dorsal fur presents lighter tips. Ventral hair coloration is lighter than dorsal coloration. The membranes, ear, and rostrum are dark. The rostrum is naked (Figure 2).
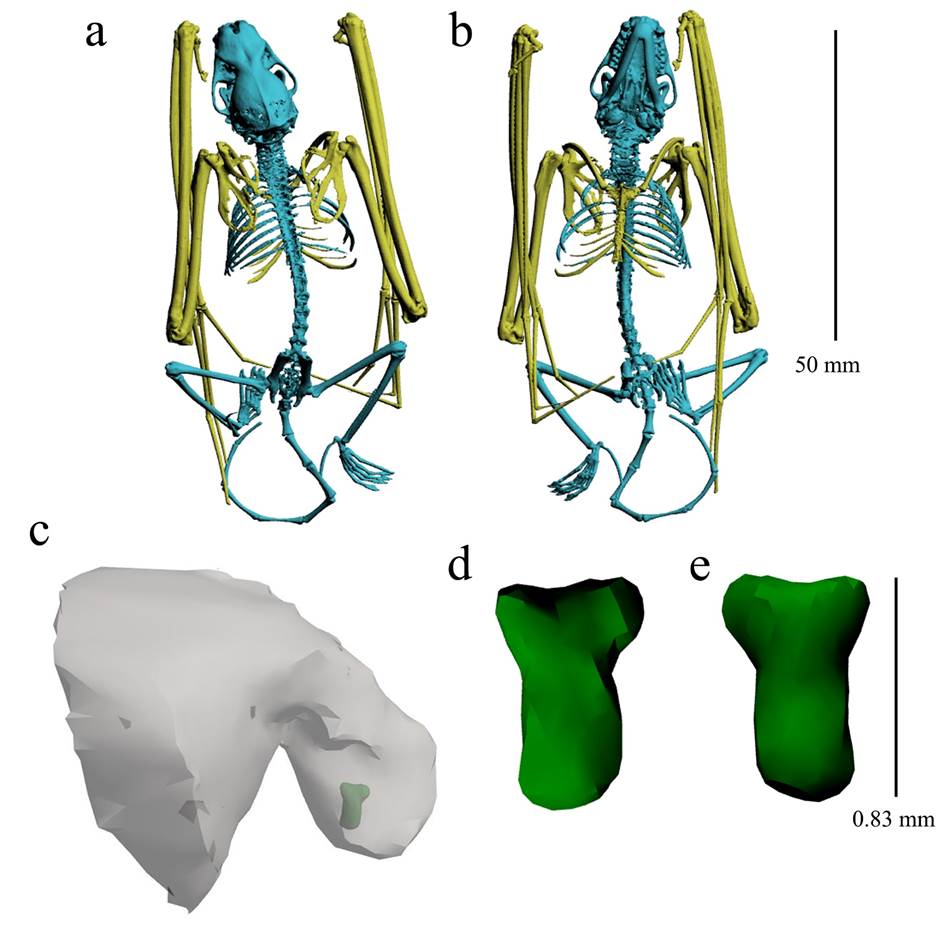
Figure 3 Details of the skeleton (a-b) and baculum (c-e) of Eptesicus miradorensis (QM JM 6365) from México. a. Dorsal view. b. Ventral view. c. Baculum shape and position (green) embedded in the penis (grey structure). d: Dorsal view of the baculum. e. Ventral view of the baculum.
Specimens from the northern distribution range (México) are similar in size to those from South America (Table 2). Colombia-Venezuela and Mexican populations are similar in two wing measurements (FA, IIIMT) between sexes; however, females have larger VMT (Mann - Whitney U - test, Z = - 2.031, p = 0.0423, n = 9) in South American populations, and IVMT (Z = - 1.979, p = 0.04783, n = 16) in Mexican populations. Similarly, both populations found no significant differences in the skull measurements (GLS, CBL, POC, BBC, BM, ZB, M3M3, CM3, ML).
Table 2 External and cranial measurements (Meas) of E. miradorensis along its distribution range. Values indicate: Mean (interval) n.
South America | Central and North America | ||||||||||
---|---|---|---|---|---|---|---|---|---|---|---|
Colombia-Venezuela | Ecuador (from literature) | Panama | Guatemala | México | |||||||
Meas | Male | Female | Male | Female | Male | Female | Female | Male | Female | ||
TL | 114.10 (101.9-129.5) 11 | 106.88 (103-130) 11 | 111 | 121 (120-122) 2 | - | - | 114.33 (109-119) 3 | 113.31 (96-125) 17 | 118.53 (110-128) 15 | ||
TV | 48.93 (43.0-53.4) 11 | 49.69 (43.0-59.2) 11 | 51 | 53 (56-50) 2 | - | - | 49.33 (47-52) 3 | 42.74 (40.0-44.4) 17 | 48.17 (40-55) 12 | ||
EAR | 16.48 (12.8-21.0) 12 | 14.33 (10.8-19.0) 11 | 18 | 22.5 (21-24) 2 | - | - | 19.5 (19-20) 3 | 14.93 (13-16.81) 17 | 17.62 (11-20) 16 | ||
W | 13.68 (10-17) 7 | 13.33 (13.5-17.0) 6 | 14.5 | 16 (15-17) 2 | - | - | 17.8 (16.3-19.6) 3 | 15.93 (14-18) 10 | 17.14 (12-21.5) 10 | ||
FA | 49.90 (48.02-51.38) 14 | 50.56 (47.65-53.53) 13 | 50.2 (49.4-51) 2 | 52.9 (52.0-53.8) 2 | 50.76 | 49.51 | 50.27 (49.42-50.99) 3 | 48.76 (46.12-52.97) 17 | 50 (47.07-53.04) 16 | ||
IIIMT | 46.02 (43.69-48.57) 14 | 47.19 (44.90-50.87) 12 | 48.3 (47.6-49) 2 | 51.25 (50.8-51.7) 2 | 48.25 | 47.35 | 46.86 (46.08-47.9) 3 | 46.25 (43.04-50.83) 16 | 47.68 (44.02-49.93) 16 | ||
IVMT | 45.24 (43.00-48.63) 14 | 46.13 (43.95-48.74) 12 | - | - | 46.27 | 46.41 | 46.18 (45.82-46.59) 3 | 45.37 (42.33-48.12) 15 | 46.93 (43.29-48.89) 16 | ||
VMT | 43.34 (40.90-46.59) 14 | 44.77 (42.62-48.27) 12 | - | - | 43.44 | 44.2 | 43.77 (43.33-44.51) 3 | 43.88 (39.70-48.11) 15 | 44.77 (42.10-47.12) 15 | ||
HF | 11.43 (10.03-12.17) 14 | 11.83 (10.77-13.31) 13 | 9 | 10.5 (10-11) 2 | 10.82 | 11.44 | 10.78 (10.09-11.53) 3 | 10.78 (8-13) 17 | 10.37 (7-12) 16 | ||
GLS | 19.14 (18.55-20.02) 9 | 19.34 (18.70-20.12) 11 | 19.85 (19.5-20.2) 2 | 20.90 (20.90-20.90) 2 | 19.49 | 19.84 | 19.85 (19.61-20.09)3 | 19.34 (17.63-21.28) 17 | 18.22 (18.42-20.17) 15 | ||
CBL | 18.16 (17.01-18.98) 10 | 18.12 (17.60-18.80) 11 | - | - | 17.92 | 18.3 | 18.21 (17.77-18.20) 3 | 18.48 (17.92-19.42) 17 | 18.42 (16.93-19.4) 15 | ||
POC | 4.28 (4.02-4.47) 11 | 4.37 (4.12-4.63) 13 | 4.3 | - | 4.1 | 4.16 | 4.17 (3.93-4.43) 3 | 4.36 (3.77-4.78) 17 | 4.26 (3.94-4.60) 15 | ||
BBC | 8.86 (8.65-9.18) 11 | 8.81 (8.62-9.00) 11 | - | - | 8.42 | 8.67 | 8.48 (8.20-8.73) 3 | 8.73 (8.20-9.52) 17 | 8.73 (8.22-9.24) 15 | ||
BM | 10.04 (9.58-10.22) 11 | 10.15 (9.82-10.46) 11 | - | - | 9.67 | 9.9 | 10.09 (9.98-10.22) 3 | 10.05 (9.63-10.73) 17 | 10.21 (9.68-10.75) 15 | ||
ZB | 13.00 (12.54-13.23) 8 | 13.10 (12.63-13.77) 10 | 13 | - | 12.82 | 12.91 | 12.95 (12.75-13.25) 3 | 13.05 (12.17-14.14) 17 | 13.06 (12.47-13.51) 15 | ||
M3-M3 | 8.19 (7.46-8.83) 11 | 8.24 (7.76-8.93) 13 | - | 8.46 | 8.35 | 8.23 (8.21-8.25) 3 | 8.21 (7.72-8.77) 17 | 8.17 (7.67-8.47) 15 | |||
CM3 | 7.34 (7.06-7.68) 11 | 7.36 (7.11-7.62) 13 | 7.5 (7.3-7.7) 2 | 7.65 (7.60-7.70) 2 | 7.56 | 7.57 | 7.53 (7.23-7.78) 3 | 7.38 (6.92-7.91) 17 | 7.32 (6.89-7.62) 15 | ||
ML | 15.19 (14.54-16.03) 11 | 15.18 (14.53-15.83) 12 | - | - | 14.8 | 14.89 | 15.11 (14.77-15.51) 3 | 14.49 (13.38-16.10) 17 | 14.65 (14.12-15.39) 14 |
The PCA analysis showed no clear distinction between North/Central and South American populations (Figure 4), with specimens from México and Colombia overlapping the first component's negative and positive axis. Specimens from Central America (Guatemala-Panamá) were more centrally distributed along the PC1. The PCA of wing measurements shows that the first principal component accounted for most of the wing size variation (88 %, eigenvalue > 1; n = 30). For the cranial characters, the first two PC accounted for the largest variation (76.04 %: PC1: 49.42, PC2: 26.62, eigenvalues < 1; n = 18). The variables that contributed most strongly to PC1 were GLS (0.6847) and ML (0.4946).
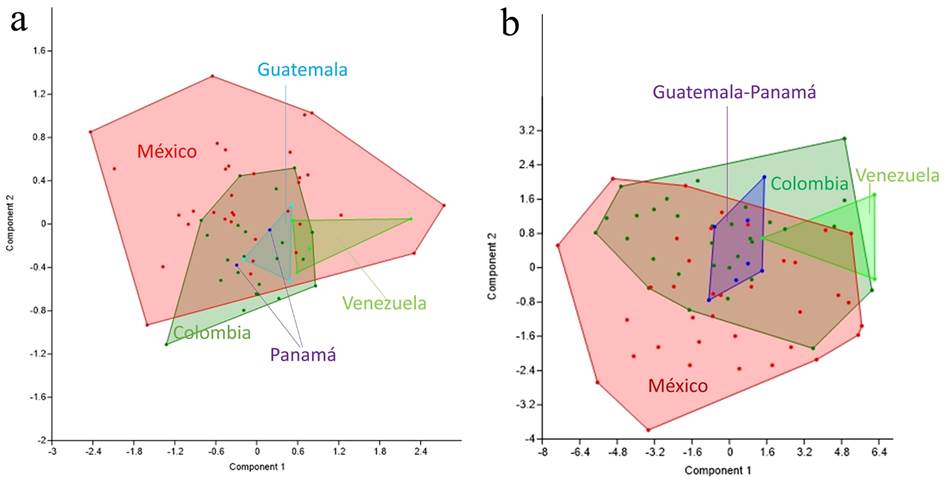
Figure 4 PCA plots of (a) cranial and (b) external measurements of Eptesicus miradorensis from different localities along its distribution range.
Distribution. We compiled 964 records from 1,098 localities in México, Costa Rica, Guatemala, Honduras, Panamá, Colombia, Venezuela, and Ecuador (Figure 5). The localities cover an altitudinal range between 35 and 4,035 m, with an average greater than 1,951 m (Table 3). The number of localities for each country and the elevation intervals are presented in Table 3. The evaluation metrics showed a model with acceptable complexity (Features classes: H, hinge; regularization multiplier: 3; AUCtrain: 0.87; AUC difference = 5 %, Partial ROC: 0; Omission rate at 10P: 0.1; Number of parameters: 55). Seventeen predictors have a low correlation according with VIF, however, five of them had low impact on the Maxent model, and thus 12 variables were used (Bio2: Mean Diurnal Range, Bio3: Isothermality, Bio8: Mean Temperature of Wettest Quarter, Bio15: Precipitation Seasonality, Bio19: Precipitation of Coldest Quarter, Prec05: Precipitation May, Prec07: Precipitation July, Prec09: Precipitation September, Prec12: Precipitation December, Srad03: Solar Radiation March, Srad09: Solar Radiation September, Wind07: Wind Speed July). The maps of suitability and distribution (Figure 5) showed that E. miradorensis has a potential distribution along the Andes in Ecuador to northern México. The higher suitability values are present in México, Guatemala, north Colombia, and Venezuela. The elevation and species suitability were positively correlated (r = 0.64, p-value < 0.001), indicating low suitability values in the northern (México) and southern (Ecuador; Supplementary material). In the Mexican Transition Zone and Colombia Andes, the species had higher suitability values at medium and high altitudes (~2000 m MSL; Supplementary material), while in the records located at low altitudes such as in northern México, the species had low suitability. Interestingly, maps (Figure 5; Supplementary material) showed that lowlands such as the Isthmus of Tehuantepec or the Nicaraguan depression and some Ecuadorian localities where the species has been recorded have inadequate climatic characteristics.
Table 3 Number of localities collected for E. miradorensis along its distribution range by country and reporting the elevation interval (Min: minimum, Max: maximum).
Elevation (m MSL) | ||||
---|---|---|---|---|
Countries | # Records | Min | Max | |
Colombia | 42 | 608 | 4,160 | |
Costa Rica | 17 | 1,083 | 1,598 | |
Ecuador | 2 | 2,415 | 2,609 | |
Guatemala | 15 | 1,653 | 1,946 | |
Honduras | 2 | 964 | 1,009 | |
México | 992 | 35 | 4,035 | |
Panama | 2 | 1,062 | 1,102 | |
Venezuela | 26 | 878 | 4,105 |
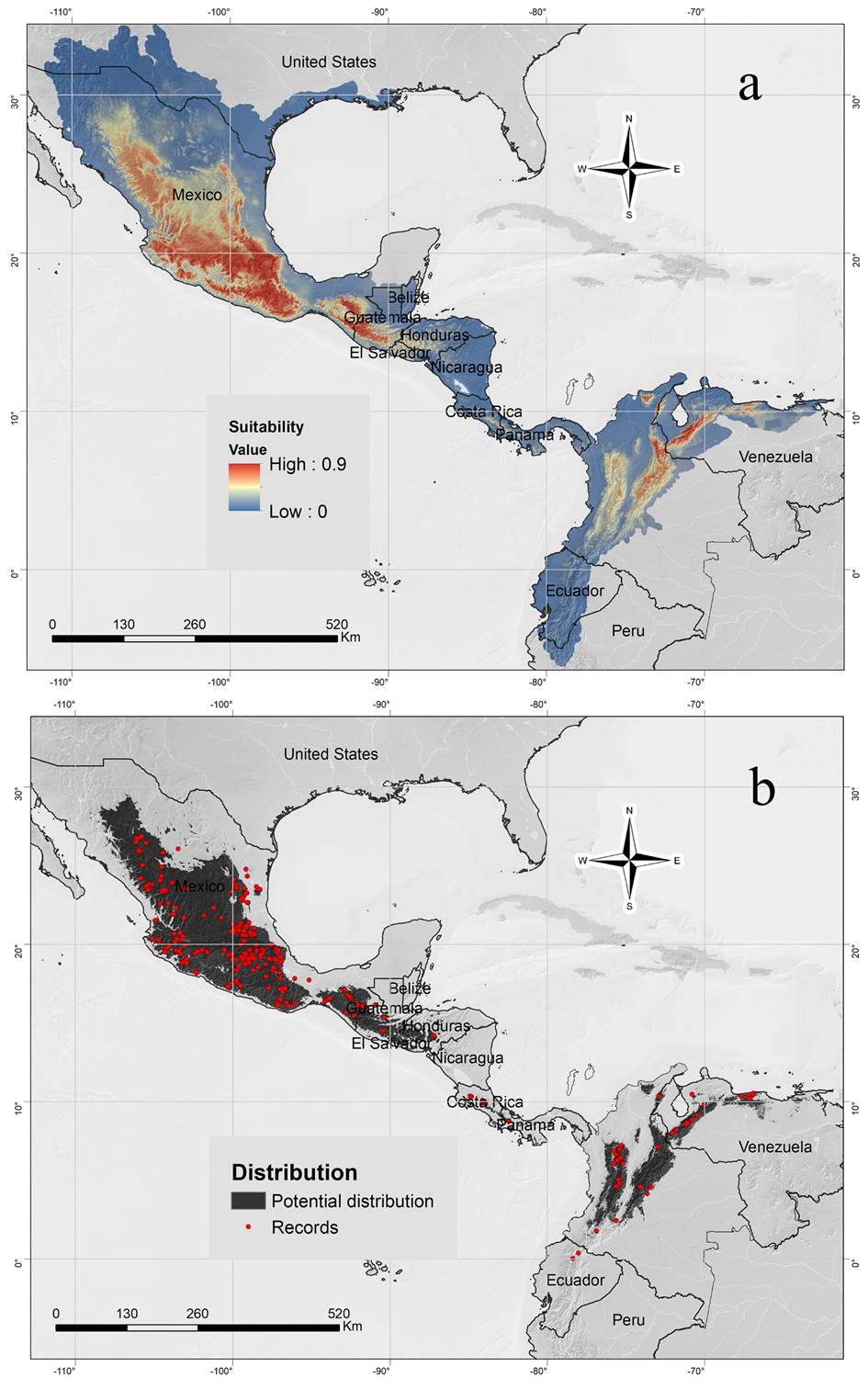
Figure 5 a. Suitability map of Eptesicus miradorensis in America using the Maxent algorithm. The higher suitability values are present in México, Guatemala, north Colombia, and Venezuela. b. Binary distribution map of Eptesicus miradorensis using the 10-percentile threshold value. Red points represent the species records.
Discussion
Neotropical Eptesicus are among the least studied bats, perhaps due to their complex systematics and taxonomy. Despite that, in the last 20 years, at least four new species of the genus have been named in South America (Miranda et al. 2006; Sánchez et al. 2019; Acosta et al. 2021; Ramírez-Chaves et al. 2021), and the presence of cryptic diversity has been highlighted (Turmelle et al. 2011; Ramírez-Chaves et al. 2021). The lack of genetic data for E. miradorensis in previous phylogenetic analyses or species descriptions (Giménez et al. 2019; Sánchez et al. 2019) limited the assessment of the morphological and phylogenetic comparisons within the E. fuscus’ subspecies and Neotropical Eptesicus in general. We highlight the importance of using different approaches to understand the real diversity and environmental ranges of cryptic taxa in Eptesicus, including assessing the specific status of the subspecies of E. fuscus and species groups such as E. chiriquinus, E. furinalis, and E. brasiliensis.
Systematics.Allen (1866) named Scotophilus miradorensis (= E. miradorensis) as a distinct species based mainly on the lustrous yellowish-brown color with a lighter base from specimens from Mirador, Veracruz, México. Thomas (1920) described Eptesicus fuscus pelliceus, supported by an adult female (BMNH 98.7.1.28) collected in “La Culata” near Mérida, Venezuela. In the description given by Thomas (1920), he mentioned that this subspecies is "very similar" to E. f. miradorensis.Cabrera (1957) and Davis (1966) synonymized both subspecies with the name E. fuscus miradorensis having priority. After revising the holotype of E. fuscus pelliceus, and specimens captured in the Serranía del Perijá near the type locality of E. fuscus pelliceus, we agree with the conclusions of Cabrera (1957) and Davis (1966).
Despite the abundance of E. fuscus in North America, only some works have assessed the taxonomic status of its subspecies (e. g., E. hispaniolae). Recent works of Yi and Latch (2021a, b) considered E. f. miradorensis as part of the same clade as E. f. bernardinus, E. f. pallidus, and E. f. peninsulae sustaining that subspecies are a product of climatic changes and insolation, these phenomena caused population divergence without speciation with secondary gene flow (Yi and Latch 2022a). However, we support E. f. miradorensis as valid species for several reasons. Firstly, some of the taxonomic conclusions of Yi and Latch (2022b) are biased for the putative identification of specimen’s tags and long branch attractions; they showed old taxonomic names (e. g., Histiotus macrotus laephotis = Histiotus laephotis) and multiple paraphyletic clades for several species, some of them demonstrated corresponding to valid species (e. g., E. diminutus [Venezuela] = E. orinocensis; Ramírez-Chaves et al. 2021). Secondly, all continental sequences from México to Venezuela represent a monophyletic linage in the tree presented by Yi and Latch (2021b); these sequences putatively form the E. miradorensis clade that differs from the clades corresponding to E. f. bernardinus, E. f. pallidus, E. f. fuscus, and the Caribbean E. “fuscus” (see Yi and Latch 2022b).
Finally, secondary gene flow could not be exclusive to sub-specific populations. For instance, Yi and Latch (2022b) recognize that the Caribbean populations of E. fuscus correspond to a valid species. Notwithstanding, they also considered the same Caribbean populations as subspecies (Yi and Latch 2022a). The phylogenetic position of the Caribbean clade in nuclear and mitochondrial analyses is nested between the east and the west clades of E. fuscus, showing that the E. fuscus is paraphyletic. Considering the Caribbean population as one (or various) specific entities reinforces the hypothesis that E. fuscus comprises several species with gene flow, including E. miradorensis.
Morphology and morphometry. We did not detect sexual dimorphism in the forearm length in South American and Mexican populations despite previous claims that females are slightly larger than males (Burnett 1983a, Kurta and Baker 1990). Similarly, we failed to find a clear pattern differentiation in the forearm and skull size between North/Central American and South American populations, even though it was suggested that wing and skull size increase from México to Honduras and decrease in Costa Rican/Panama populations, while wing size decrease and skull size increase towards South America (Burnett 1983a). Previous conclusions might be biased by sample size and the inclusion of specimens from several subspecies. Since subspecies such as E. f. dutertreus from Cuba exhibit a high degree of intra-sexual variation and are dimorphic in skull traits (Ariosa Olea and Mancina 2018), a larger sample should exist be evaluated to explore sexual dimorphism in E. miradorensis and the intermediate size of Central American populations.
The information on the baculum morphology of Neotropical Eptesicus and other Neotropical vespertilionids is scarce (Brown et al. 1971). Hamilton (1949) described the baculum morphology of E. fuscus from North America, which is similar in size (0.8 mm length) and general morphology to the one observed in E. miradorensis described here. The slight differences between the specimen illustrated here (QM JM 6365) and E. fuscus (in Hamilton 1949) are the usually sharp and well-marked distal and proximal points in E. fuscus (more rounded in QM JM 6365). Additionally, there are major differences between the baculum of E. fuscus and E. miradorensis compared with the small-sized E. furinalis. The baculum of E. furinalis from Nicaragua (Brown et al. 1971) is very large (0.9 mm length, 0.6 mm base width) contrasted with E. fuscus and E. miradorensis (0.8 mm length, 0.5 mm base width) considering the differences in body size among these species. The bacular morphology has been used as a taxonomic trait in several vespertilionid genera in other continents (e. g., Taylor et al. 2018; Srinivasulu et al. 2019), and often allows differentiation among cryptic taxa (Herdina et al. 2014). However, these characters have seldom been used in the taxonomy of neotropical Eptesicus. Therefore, variation in this structure should be tested for differentiation in Neotropical Eptesicus and other American vespertilionids.
Distribution. Although recent records have corroborated the current presence of E. miradorensis (as E. fuscus) in Ecuador (Arguero and Albuja 2012), one overlooked historical record from this country (Lönnberg 1921) constitutes the southernmost record of this species. Lönnberg (1921) recorded a female specimen (as E. fuscus pelliceus) collected in 1917 above Quito (3,352 m elevation) deposited in the collections of the Naturhistoriska riksmuseet (NRM), Stockholm. Lönnberg (1921) mentioned that the specimen closely resembled E. f. miradorensis and E. f. pelliceus and was assigned to the latter because the fur is basally blackish. The specimen was described as having long and fluffy hair (9 mm), large FA (51.9 mm), and GLS (19.5 mm). Lönnberg (1921) match the diagnostic characters of E. miradorensis. The specimen reported by Lönnberg (1921) was not found in one visit to the NRM collections and is apparently lost (Daniela Kalthoff 2014, comm, pers.); however, the remaining information associated with the specimen cast no doubts on its identification. Closer localities from Colombia to the Ecuadorian records are Farallones, Department of Valle del Cauca (Western Andes; Alberico 1994), and the Macizo Colombiano (Central and Western Andes; Ramírez-Chaves 2008; Ramírez-Chaves et al. 2010), in the Department of Cauca, not Medellín, Colombia as stated by Arguero and Albuja (2012).
It is essential to highlight the strong association of E. miradorensis to mountain ecosystems, especially in elevations over 1,500 m in México and Colombia (Davis and Gardner 2008, Ramírez-Chaves 2008). Elevation and additional environmental variables such as elevation, precipitation, and slope have been suggested to play an important role in the distribution of this species (Pérez 2011). In addition, elevation explains significant amounts of morphological differentiation in E. fuscus (including E. miradorensis as subspecies of E. fuscus; Burnett 1983b). The results of the Maxent model indicated that specific environmental variables could limit the regional distribution of the species (Precipitation, Solar Radiation, Wind Speed, altitude, and latitude). It is proposed that biotic interactions, such as exposure to physical stress limit distribution at lower latitudes, but, in contrast, abiotic factors such as predation and interspecific competition restricts the distribution at higher latitudes (Brown et al. 1996). Results of the MaxEnt that showed low suitability values in northern México and Ecuador would indicate that the distribution boundaries of E. miradorensis are related to the effects of the latitude. Furthermore, the southern limits of the species in South America remain unclear, and the role of the Amotape Huancabamba Zone as a possible barrier needs to be assessed, as suggested for other taxa (Quintana et al. 2017). Although in South America E. miradorensis inhabits predominantly Andean forests at medium to high elevations, some records below the 1,000 m of altitude in Colombia captured by us come from dry forests within forested areas adjacent to dry shrubs. In these dry forests, the species was reported lactating in January 2016. According to recent captures in urban and peri-urban areas in Central Cordillera, the species supports some ecosystem transformation. Specimens have been captured leaving from house roofs in which other species such as Molossus molossus inhabit.
With the elevation of E. miradorensis to the species level, currently, there are 12 recognized species of short-eared Neotropical Eptesicus (Sánchez et al. 2019; Acosta et al. 2021; Ramírez-Chaves et al. 2021). In addition, the systematics of Eptesicus remains controversial because some authors (e. g., Giménez et al. 2019) include the long-eared bats of the genus Histiotus as a subgenus of Eptesicus. Therefore, the information we provided here can be helpful for additional integrative analyses at a continental scale. If Histiotus is considered part of Eptesicus, the richness of this genus could reach more than 37 species globally, being one of the family's most diverse genera of insectivore bats Vespertilionidae after Myotis, Murina, and Pipistrellus (Moratelli et al. 2019; Burgin et al. 2020).