1. Introduction
Primary ettringite is produced from the reaction of aluminates and sulfate ions from clinker (C3A; C4AF; SO3) during the hydration process. However, this compound becomes unstable when concrete temperatures exceed 60-65ºC during the first hours after casting. This situation can occur due to excessive hydration heat generated by cement hydration or even by thermal curing processes that are usually adopted for pre-cast elements in the industry. Thus, after cooling, DEF can occur (Taylor, 1997; Bauer, 2006; Ifsttar, 2018).
Several laboratory studies are presented in the literature, although different parameters impair accurate comparisons. Aside from sulfate ions and temperature, the main conditional factors are the presence of aluminates and high moisture (Mehta; Monteiro, 2014; Neville, 2016; Kchakech et.al., 2016; Thiebaut et.al., 2018; Ramu et.al., 2021).
Other influential factors can activate DEF more quickly. Among them are the types of materials used for concrete and exposure conditions (Fu et.al., 1997; Leklou et.al., 2013). In relation to concrete expositions, moisture is necessary to promote DEF, besides temperature rise at the first hydration stages of cement (Godart, 2017). In addition to the level of temperature, the dwell time of the peak of temperature also influences the rate of ettringite crystallization (Kchakech et al., 2016; Giannini et al., 2018). Some researchers evaluated the effect of pozzolanic admixtures (Al Shamaa et.al., 2016; Dayarathne et.al., 2013; Ramlochan et.al., 2013; Amine et.al., 2017; Asamoto et.al., 2017; Leklou et.al., 2016; Rashidi et.al., 2017), even though there has been no clear agreement on these incorporations to date. Some researches point to pozzolan admixtures as a mitigative measure to reduce temperature rise of concrete during the cement hydration (Ramlochan et al. 2003; Mehta; Monteiro, 2014; Amine et al., 2017). Mineral admixtures have been studied in relation to their interaction along cement hydration (Dayarathne et.al., 2013; Amine et.al., 2017).
Some researchers indicate a beneficial effect of fly-ash (Ramlochan et.al., 2003; Dayarathne et.al., 2013; Amine et.al., 2017; Asamoto et.al., 2017; Leklou et.al., 2017), but others suggest this admixture just delays the neoformations and the expansive process of DEF (Schovanz, 2019; Bronholo, 2020; Schovanz et.al., 2021).
The content of pozzolans varies between studies aimed at mitigating DEF occurrence. Some researchers tested a range of 15% as suitable for prevention (Amine et.al., 2017), whereas others indicate that 30% of fly-ash is required (Leklou et.al., 2017). These differences are usually related to the type and composition of the mineral admixtures (Ramlochan et.al., 2003).
The concentrations of some components can interfere with the chemical process. Taylor et al. (2001) points out the relation of SO3/Al2O3 in DEF occurrence. In the case of cement replacement by fly-ash, this relation tends to reduce and minimize DEF expansions (Ramlochan et.al., 2013; Leklou et.al., 2016).
The manufacture of cement involves incorporating different mineral admixtures and contents, depending on the local availability of supplies. This is a global practice; aside from reducing energetic consumption in the clinker production, there exists an optimal usage of residues to offset one’s carbon footprint. Furthermore, this practice can also improve concrete performance and resistance to chemical attacks. However, little is known about blended cements. The performance of high early-strength cement is known to bring severe damages to concrete (Schovanz et.al., 2021) and is responsible for several cases of DEF diagnosed in some elements and structures in Brazil (Hasparyk et.al., 2016; Godart, 2017; Hasparyk and Kuperman, 2019). High contents of cement and specific physical-chemical characteristics can interfere in heat liberation during hydration heat (Melo et.al., 2011; Godart, 2017).
Some studies at laboratories with mortars can be observed in the literature to favor DEF (Adamopoulou et.al., 2011; Dayarathne et.al., 2013; Leklou et.al., 2016). The same practice was previously used for other types of pathologies (like alkali-aggregate reactions) in order to simplify test methods and include them in the standards.
Evidence of the relation between C3A and the intensity of formed ettringite can be observed in the studies involving mortars by Katsioti et al. (2011). Furthermore, researchers like Asamoto et al. (2017) had determined at the laboratory that DEF will occur just in the presence of contents of SO3 above 3%. In addition, Adamopoulou et al. (2011) identified DEF prematurely (3 months) in mortars that were thermally cured at 50ºC, even though the majority of studies state that maximum limit temperatures should not exceed 65ºC (Al Shamaa et.al., 2016; Godart, 2017). Bronholo (2020) did not detect expressive expansions in mortars with fly-ash cement when mortars were exposed to coupled attacks of DEF and ASR over one year. However, DEF was discovered to be present in the cement matrixes of the mortars through microstructural analyses.
This study aimed to evaluate the influence of different types of cement on DEF occurrence using laboratory tests and analyses performed in cement composites.
2. Experimental program
2.1 Materials
The experimental program included four different types of Portland cement as follows: High Early Strength Cement (CP V); Blended Cement with Fly-Ash (about 9% of pozzolan - CP II-Z); Blended Cement with Limestone Filler (about 6% of filler - CP II-F); Pozzolanic Cement with Fly-Ash (about 24% - CP IV). Table 1 presents chemical characteristics of cements obtained by X ray fluorescence and also their fineness by Blaine.
Table 1 Main characteristics of cements
Parameter (in %) | CP V | CP II-F | CP II-Z | CP IV |
---|---|---|---|---|
CaO | 0.93 | 0.74 | 1.02 | 0.85 |
SiO2 | 19.56 | 20.41 | 22.69 | 30.73 |
Al2O3 | 5.26 | 4.55 | 5.82 | 8.60 |
Fe2O3 | 2.87 | 2.12 | 3.07 | 3.83 |
Na2Oeq | 0.54 | 0.47 | 0.41 | 1.02 |
MgO | 1.03 | 1.35 | 1.10 | 1.57 |
SO3 | 2.93 | 2.52 | 1.85 | 0.05 |
SO3/Al2O3 | 0.56 | 0.55 | 0.32 | 0.01 |
Insoluble residue | 0.55 | 5.74 | 8.72 | 24.48 |
Loss on ignition | 4.28 | 8.71 | 6.08 | 5.13 |
Blaine Fineness | ||||
(in cm2/g) | 4,250 | 4,450 | 5,110 | 4,050 |
*Na2Oeq = 0.658 K2O + Na2O.
The fine aggregate used in the experiments has 4.8 mm of maximum size and is alkali silica reaction (ASR) innocuous, according to the previous accelerated mortar bar test (Brazilian Standard NBR 15577, 2018).
2.2 Procedures of casting and curing
The mix of mortars presented a proportion of 1:2.275 of cement: fine aggregate and the water-cement ratio was equal to 0,485, according to ASTM C 1012 (2018).
The procedure and thermal cycle adopted to induce DEF was previously proposed by Schovanz (2019) and Hasparyk et al. (2020). Specimens were cast and maintained for 6 hours in a moist room until the beginning of the thermal cycle (pre-curing period). In the sequence, specimens were submerged in water with a gradual increase of temperature from 25ºC up to 85ºC, remaining in this condition for 12 hours, followed by cooling up to 38ºC. Both the heating and cooling rate was 10ºC per hour over time and up to one year (Figure 1).
2.3 Laboratory investigations
Physical, mechanical and microstructural analyses were performed to evaluate DEF. Expansions and mass variations were monitored periodically with 3 mortar prisms for each cement (25x25x285 mm) over 365 days. Mechanical properties such as compressive strength and diametral tensile strength were also determined in 4 cylindrical specimens for each age and cement (50x100 mm) over time by NBR 7215 (ABNT, 2019) and NBR 7222 (ABNT, 2011), respectively. The microstructural analyses were performed through a scanning electron microscope with EDS. Fracture samples collected from the internal part of the specimens after mechanical tests were investigated by a secondary electron detector (SE).
3. Results and discussions
According to Figure 2, values of expansion for mortars containing cement with no admixture were much higher than those in the presence of some admixture. The first major increase began after 150 days and lasted up to 200 days; after that, a dormancy period was observed for up to 260 days, followed by another increase in expansion for up to about 300 days. Then, the growing rate of expansion was intensified up to 365 days (the maximum expansion was 1.8%). Although the fly-ash cements (both, CP II-Z and CP IV) produced reduced expansions (below 0.10% at 365 days), a higher dispersion for expansions was observed over time. Cement with limestone filler (CP II-F type) performed entirely differently from the others. Over time and up to about 300 days, there were minor expansions below 0.06%. After this period, a significant growth rate was perceived for up to 365 days, when mortar achieved an average expansion of 0.45%.
It can be mentioned that fly-ash influences the contents of Al2O3 and SO3 (Table 1) of cement, with a significant reduction in the SO3/Al2O3 relation followed by reduced DEF expansions.
According to the literature (Ramlochan et.al., 2013; Leklou et.al., 2016), those parameters can support a better understanding of the performance of cement with fly-ash (CP II-Z and CP IV) in relation to the other. CP V and CP II-F do not contain pozzolans, and the main difference is related to the fineness (CP V - 4.250 cm²/g; CP II-F - 4.450 cm²/g) and the presence of limestone filler (5.74%). Cement fineness accelerates hydration reactions and, thus, the heat of hydration. Shamaa et al. (2016) had previously presented the effect of filler in the kinetic of DEF reaction; however, it is not capable of mitigating DEF. According to the authors, expansions can increase and the latency period can be reduced with the increase of particles of limestone filler in the mixes. The reaction of dissolved carbonate ions from the limestone filler with the aluminate phases of Portland cement leads to the formation of carboaluminates as opposed to sulfoaluminates, and stabilizes the ettringite that is produced at early ages.
Mechanical properties were coherent among tested cements and were determined by comparing expansions. The compressive strength of mortars increased from one month to six months for all tested cements (Figure 3). On the other hand, at 12 months there was a decrease. The drop of this property in comparison to 1 month was much more critical for the cement with no admixture (CP V) and the one with limestone filler (CP II-F), 63% and 54%, for expansion levels of 1.79 and 0.45, respectively. Even though the level of expansions for CP V was higher than the one for CP II-F, since CP V is a high early strength cement, mortar achieved higher strengths at the first ages compared to the CP II-F concrete. Furthermore, expansion stages above 0.40% are too high to impact strengths and damage cement composites, as expected (Schovanz, 2019; Bronholo, 2020).
In the presence of fly-ash, and especially for the CP II-Z, a decrease was not observed at one year, considering low expansions (less than 0.10%), but in relation to 6 months, there was a reduction of just about 2% in the presence of this cement, meanwhile pozzolanic cement (CP IV) suffered a decrease of about 25% for the same period (Figure 3 and Table 2).
Even though compressive strength is not the main property influenced by the most expansive reactions, it is not valid in the case of DEF. Microcracking generated by DEF starts in the interfacial transition zone (TZ) between cement and aggregate, leading to negative influence in this property, and especially in mixes with cement pozzolanic admixtures (Taylor et.al., 2001). Self-healing of cracking in cement composites can be achieved through the use of pozzolan admixtures (Van Tittelboom and De Belie, 2013). This phenomenon can explain the better performance of composites with fly-ash in relation to the compressive strength (Termkhajornkit et.al., 2009).
Tensile strength featured a slightly higher sensitivity due to the higher expansions of CP V (1,79%) and CP II-F (above 0.45%). Soon, at six months there was already a drop of this property followed by a new drop at 12 months. At six months, tensile strength had a reduction of about 9% for both types of cement. At the last age, mortars with CP V and CP II-F suffered a decrease of 68% and 57%, respectively (Table 2 and Figure 4). Some researchers have shown that expansions of the order of 0.2-0.3% are able to affect tensile strength meanwhile compressive strengths are influenced at higher levels of expansions (Giannini et al., 2018; Leklou et al., 2016).
Mortars with fly-ash performed differently due to an increase of tensile strength up to the first six months, somewhat following the behavior of compressive strength, but over time and at 12 months, there was a drop of 19% and 12% for CP II-Z and CP IV, respectively (Figure 4). The initial gain in the tensile strength occurs due to the pozzolanic reactions involving the mineral admixtures that are present in these cements.
Table 2 Behavior of mortar properties in relation to expansions at one year
Cement Type | % Reduction in Compressive Strength | % Reduction in Tensile Strength | Expansion (%) |
---|---|---|---|
CP V | -63 | -68 | 1.79 |
CP II-F | -54 | -57 | 0.45 |
CP II-Z | 93 | 9 | 0.08 |
CP IV | 1 | -9 | 0.10 |
According to Table 2, it is evident that for expansions above 0.45%, the damage to mechanical properties is very expressive, with reductions above the order of 50%. Furthermore, for expansions measured in mortars of about 0.10%, there are already clear signs of a beginning of DEF deterioration since tensile strength appears with a significant reduction of about 10% at one year. This negative reflex raises concern about the performance of concretes cast with this type of pozzolanic cement in relation to DEF. The level of expansion does not seem as high as expected, but mechanical consequences are detected, and DEF was observed through microstructural analyses. Thus, for DEF studies in mortars, a threshold at one year should be less than this value. Further research is necessary to define a completely reliable test method and limit of expansions.
A correlation between both the strength and the level of expansions is presented in the Figure 5 and Figure 6. As expansions increase, the strengths reduce for the cement types CP V and CP II-F; on the other hand, it is not possible to stablish a correlation in the presence of those cements with fly-ash (CP II-Z and CP IV). In order to corroborate this statement, microstructural analyses were performed and clearly indicate formations of ettringite crystals in the mortars containing fly-ash. Mortar samples from the other cements were also analyzed and DEF detected. In Figures 7 - 10, DEF occurrence can be confirmed, and some EDS spectra are presented confirming the chemical feature of ettringite formations.

Figure 7 Micrographs of mortars with CP V at one year: a) Voids filled and intense microcracking; b) Fragile paste; with ettringite formation; c) TZ weakened and massive ettringite in the cement paste; d) EDS spectrum of ettringite crystals indicated in the Fig. 7a.

Figure 8 Micrographs of mortars with CP II-F at one year: a) DEF filling a void; b) Compressed Ettringite crystals in the cement paste; c) Loss of adherence between cement paste and aggregate and neoformations in the surface of aggregate; d) EDS spectrum of massive ettringite indicated in Fig. 8a.
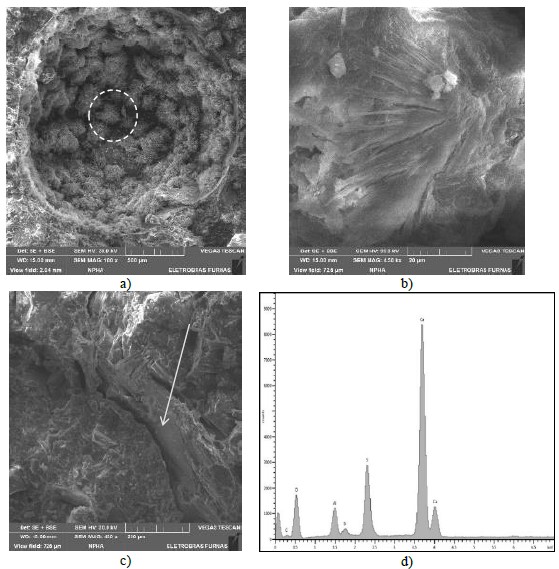
Figure 9 Micrographs of mortars with CP II-Z at one year: a) Agglomeration of ettringite in a void; b) Massive formations in the cement paste; c) Detachment between paste and aggregate; d) EDS spectrum of ettringite agglomerations indicated in Fig. 9a.
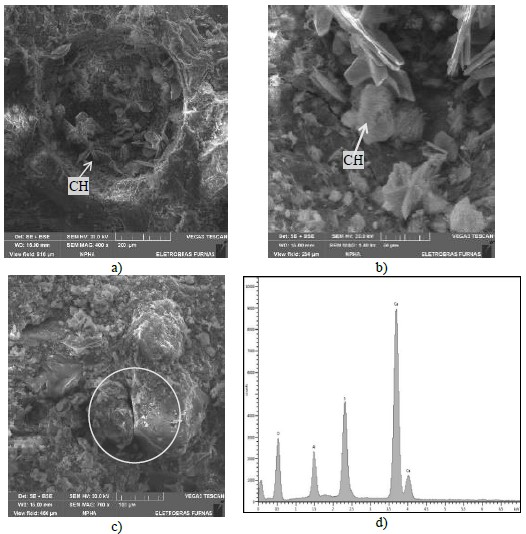
Figure 10 Micrographs of mortars with CP IV at one year: a) CH crystals inside a void with a few Ettringite formations; b) Detail of a few ettringite crystals and CH; c) Some grains of aggregate disconnected in the mortar; d) EDS spectrum of crystals indicated in Fig. 10b.
Analyses performed at one year by SEM and EDS had evidenced the presence of delayed ettringite in some conditions. It is possible to compare differences from each cement used and also the magnitude of neoformations. Expansions, as well as mechanical behavior, can be explained by microstructure. DEF damages for tensile strength followed this descending order: CP V; CP II-F; CP II-Z; CP IV. From a microstructural point of view, ettringite neoformation incidences in the voids and cement paste and fragility of paste were observed in the same order. However, some loss of adherence between paste and aggregate was detected in all the samples, independent of the cement type. Furthermore, for mortars cast without fly-ash pozzolan (CP V e CP II-F), the presence of ettringite neoformations was more significant. Compressive strength behavior was similar to tensile strength, even though cement CP II-Z did not promote an abrupt decrease when compared to cement CP IV at one year. In relation to DEF expansion levels, major values were achieved for cement with no admixture (CP V), followed by the CP II-F cement. In addition, major damages for cement pastes and TZ were demonstrated by SEM in the presence of DEF.
4. Conclusions
The present study evaluated DEF in mortars with different cement types. The results from the experimental program led to the following conclusions:
- Cements without fly-ash are more prone to DEF, with major expansions and negative influences in mechanical properties;
- The presence of limestone filler promotes a modification in the kinetics of the reaction, delaying the expansive process from DEF, but with no mitigation;
- Mixes cast with fly-ash cements had performed better, with minor negative consequences in the mechanical properties as well as fewer levels of expansion due to the self-healing properties of fly-ash in the presence of water;
- The procedure at the laboratory was able to induce DEF and promoted influences in cement paste integrity, including the fragility of the paste and transition zones and the mechanical properties. Anyway, lab procedures must be carefully adopted for mortars as different expansive behaviors are observed between tested cement. Cement with no admixture of high early strength could be evaluated at five months, but the same did not occur for the other types, appointing the necessity of later ages of evaluation, including after one year;
- Damages from induced DEF to mechanical properties of mortars were very expressive. For expansions above 0.45%, reductions of the order of 50% occurred. Furthermore, for expansions of about 0.10%, clear signs of DEF deterioration were observed in the mortar’s microstructure and tensile strength at one year, even in the presence of fly-ash, flagging the risk of damage evolution.