1. Introduction
The restoration and rehabilitation projects of social works in the Historic Center of Havana must guarantee a safe, durable, comfortable property with functional amenities for the building`s tenants. During the construction phase, it is necessary to carry out quality controls on the reinforced concrete elements to evaluate resistance, hardness, or possible pathologies such as plastic deformations and cracks.
Various techniques such as resistance to penetration, pull-out and pull-off, extraction of concrete probes, resonance, and permeability, allow to evaluate the constructive quality of a property, however, they have the disadvantages of extensive research time, high costs and/or physical aggression to the structure. This problem has led to the need to develop non-destructive testing (NDT) methods that allow, in an economical, fast, and effective way, to estimate the resistance, state of conservation and durability of a building.
Among the most important factors that support the use of NDT are the possibility of evaluating the vulnerabilities of the building based on the detection of areas with damage, estimation of the compressive strength (fc) and variations in quality according to the project requirements (Breysse, et al., 2017, Chandak and Kumavat, 2020). The purpose is to determine the quality and integrity of the parts and components of a structure without affecting their performance or functions for which they were designed (Helal, et al., 2015, Hussein and Abdi, 2021).
The quality of concrete is generally expressed in terms of resistance since buildings must be designed to support different types of loads. Concrete is a material that is made up of cement, fine and coarse aggregates, water, and additives, and once it sets it is necessary to know the resistance value acquired, mainly after 28 days. For this purpose, numerous calculation equations have been developed that allow estimating the concrete's fc from the values of the Rebound Hammer (RN) (Lima and Silva, 2000), ultrasonic pulse velocity (UPV) (Hannachi and Guetteche, 2012, Popovics, 1991) or the measurement of the apparent resistivity (ρ) of the concrete (Araújo and Meira, 2021).
Another widely applied methodology is the SonReb method, which combines the results of the RN and the UPV. Since its implementation in the 60s of the last century by Facaoaru (Facaoaru, 1969), This has become the most widely used NDT to estimate the fc of concrete.
Due to the fact that it is a non-destructive technique, the veracity and precision of the results may be affected by errors in the execution of concreting, age of the structure, presence of reinforcements, mix design, carbonation, porosity, cracking, characteristics of the aggregates and environmental parameters such as temperature and relative humidity (Cristofaro, et al., 2020, Hussain and Akhtar, 2017).
Cuban construction regulations do not have the SonReb method implemented as a valid NDT procedure for fc estimation. In this sense, they only propose the use of Schmidt rebound hammer test (NC 246, 2003), and the UPV UPV (NC 231, 2002) independently as methodologies to estimate the resistance, quality and durability of the work. In the bibliographical research carried out, only two previous investigations in Cuba based on the SonReb method were identified (Navarro, et al., 2019, Ricardo, 2018).
The objective of this study is to test the feasibility of using the SonReb model in real structures where national materials are used, based on the analysis of the results obtained. For this, eight models were correlated, and the calculated fc were compared with the reference value by breaking the specimen. The study was carried out in a work under restoration in the Historic Center of Havana. The rehabilitation conceived the construction of columns and beams, of which nine columns were chosen to carry out the study. The concrete used was designed from aggregates extracted from Cuban quarries. The reference specimens were taken in-situ, from the same concrete mixture with which the reinforced concrete columns were formed.
2. Materials and methods
2.1 Mix design
For the manufacture of the concrete, ordinary Portland cement was used, which corresponds to the denomination P-35 (Type I) from the Cementos Curasao Factory, Mariel municipality, Artemisa province. Table 1 shows its main characteristics, in accordance with the provisions of the Cuban Standard (NC 1340, 2021).
Table 1 Physical properties of OPC (P-35)
Properties | Values | Acceptance |
---|---|---|
Specific surface (Blaine) (cm2/g) | 3317 | min. 2800 |
Fineness (%) | 1,7 | max. 10 |
Initial setting time (min) | 102:00:00 | min. 45 |
Final setting time (h) | 03:02:00 | max. 10 |
Volumetric weight (kg/m3) | 1168 | - |
Density (g/cm3) | 3,15 | - |
Normal consistency (%) | 24,5 | - |
The fine and coarse aggregates used in the preparation of the concrete mix are of natural origin, extracted from the Dragon Camoa quarry located in the Mayabeque province, and from the Alacranes quarry located in the Matanzas province. All the tests were carried out according to the corresponding Cuban Standards (NC 177, 2002, NC 181, 2002, NC 182, 2002, NC 186, 2002). Tables 2 and 3 details the physical properties of the aggregates and their granulometric distribution.
Table 2 Physical properties of fine and coarse aggregates
Properties | Sand | Coarse |
---|---|---|
Current specific gravity (g/m3) | 2.65 | 2.62 |
Saturated specific gravity (g/m3) | 2.70 | 2.66 |
Apparent specific weight (g/m3) | 2.75 | 2.70 |
Percentage absorption (%) | 0.60 | 1.50 |
Loose volumetric mass (kg/m3) | 1443 | 1309 |
Compacted volumetric mass (kg/m3) | 1616 | 1462 |
Percent of gaps (%) | 39.60 | 44.80 |
Finest Material-Sieve 200 (%) | 3.00 | 1.20 |
Table 3 Granulometric distribution of the aggregates used
Sieves (mm) | |||||||||
---|---|---|---|---|---|---|---|---|---|
Aggregates | 19.1 | 12.7 | 9.52 | 4.76 | 2.38 | 1.19 | 0.59 | 0.295 | 0.149 |
Fine | 100 | 100 | 95 | 90 | 74 | 45 | 28 | 14 | 5 |
Coarse | 100 | 98 | 63 | 6 | 2.5 | 0.0 | 0.0 | 0.0 | 0.0 |
A ratio of fine and coarse aggregate of 55/45 respectively was used. The water/cement ratio used was 0.45. The estimated compressive strength (fc) is 30.0 MPa. To improve the workability of the fresh concrete, the additive MAPEI Dynamon SRC-20 was used. This is an acrylic-based super-fluidizing additive (advanced second generation) modified for pre-mixed concretes characterized by a low water/cement ratio, high mechanical resistance and long maintenance of workability. The settlement measured by the Abrams cone was between 14 and 16 cm. The amount of materials used for a cubic meter of concrete is shown in Table 4.
2.2 Experimental Methodology
The taking of samples of fresh concrete for the preparation of the concrete probes was carried out directly in the construction site in accordance with the Cuban Standard. (NC 167, 2002). The concrete probes were kept under curing in a pool for 28 days and later tested by breaking in a concrete press. The average fc result issued by the laboratory was 27.0 MPa. This will be the reference value for comparison with the results obtained by the different SonReb methods used.
For the execution of the research project, nine columns were selected (C2, C4, C5, D2, D4, D5, E2, E4, E5), located on the second level of the building. They have a cross section of 300 x 300 mm, and a length of 4500 mm. In each column, two test points were prepared from the base: the first located at 1680 mm and the second at 2680 mm, with the purpose of evaluating them in the lower and upper part of each column. This makes it possible to identify significant differences between the mass of the concrete because of execution errors, such as pouring, curing and compaction. In each test zone, 10 measurements were made, for a total of 20 results for each column. All measurements were made between 28 and 56 days after the columns were cast.
For the selection of the SonReb methods, a bibliographic research of the empirical equations developed was carried out. A sample of eight was selected, ranging from its beginnings in 1979 to the present in 2020. Table 5 shows the equations used.
Table 5 SonReb equations applied for estimation of compressive strength
Year | Author | Equation | Units (fc, UPV) | Correlation type |
---|---|---|---|---|
1979 | Bellander (Bellander, 1979) |
|
MPa, km/s | Polynomial |
1979 | Meynink et al. (Meynink and Samarin, 1979) |
|
MPa, km/s | Polynomial |
1993 | RILEM (RILEM NDT 4, 1993) |
|
MPa, m/s | Power |
1994 | Tanigawa et al. (Faella, et al., 2011) |
|
MPa, m/s | Linear |
1996 | Arioglu & Köylüoglu (Arioglu and Köylüoglu, 1996) |
|
MPa, km/s | Power |
1996 | Ramyar et al. (Ramyar and Kol, 1996) |
|
MPa, km/s | Linear |
1999 | Khedar et al. (Khedar, 1999) |
|
MPa, m/s | Power |
2020 | Chandak & Kumavat (Chandak and Kumavat, 2020) |
|
MPa, m/s | Power |
2.3 Equipment used
As non-destructive techniques for estimation by SonReb, a Proceq digital Schmidt Hammer was used. The surface was prepared according to current regulations (ASTM C805, 1997, NC 246, 2003). The program used to download the data was Proceq Hammerlink®. A Proceq Pundit Lab+ ultrasound with 54 KHz transducers was used. The data was downloaded by the software Proceq PunditLink®. The measurement technique used was "Direct Transmission". To locate the reinforcing bars embedded in the columns, a rebar locator Proceq Profoscope was used (Figures 1-2).
3. Results and discussion
Tables 6-7 show the results obtained from the UPV and RN measurements performed on the columns. The statistical analysis reflects that, in all the elements studied, the CV did not exceed 10% with a normal distribution of the data. The total number of values analyzed (n) for the application of the models was 162 results. For mathematical development, the UPV was established as an independent variable.
The average UPV obtained in all cases are higher than 3500 m/s, which responds to high-quality concrete (NC 231, 2002). In the same way, the registered rebound indices show the value Q>40 for an estimated resistance greater than 30 MPa (NC 246, 2003).
The results are shown in Table 8. In the calculation of the fc of the proposed models (Table 9), a greater dispersion is observed in the CV of the results, which is due to the design of each equation. Based on the results obtained, the models with the best approximation to the reference value are RILEM and Tanigawa et al. with 26.29 MPa and 26.07 MPa respectively (Figure 3), with an error of less than 4% for both cases (Figure 4). Figure 5 shows how some models present a linear behavior, while others reflect a greater dispersion in the results. This variability is determined by the design of each equation (linear, polynomial and power) for the estimation of the fc, which is dependent on the values of RN and UPV obtained.
To determine the relationship of these variables on the fc, the results were independently correlated by combining the RN and the UPV obtained with the calculated fc. The results are shown in Figures 4, 5, 6, 7, 8, 9, 10, 11. For the construction of the graphs, the data was organized from the statistical processing, so that all the models have the same population of data.
The analysis of the behavior of the RN and UPV variables with respect to the calculated fc shows that in the models where there is a coherent correlation for both pairs of variables (RN vs fc, UPV vs fc) (Figures 6, 9), the calculated fc tends to get closer to the reference value. For the cases of the models proposed by Bellander, Meynink et al., Raymar et al. and Kheder (Figures 7, 8, 10, 12 respectively), although they show a very good correlation between the RN vs fc, with respect to the UPV there seems to be no influence of this variable on the fc, so the result obtained differs far from the reference value of 27.0 MPa. Perhaps this is the reason why the differences from the reference were greater than 20% for all cases. An interesting result was obtained from the Chandak & Kumavat model (Figure 13), where the RN and UPV relationships are opposite with respect to the fc. That the error obtained is less than 15% may be associated with the fact that, although the correlations are contrary, there is a linear trend of the RN and the UPV on the calculated fc.
Table 6 Results of measurements and statistical parameters
Columns | ||||||||
---|---|---|---|---|---|---|---|---|
C2 | C4 | C5 | D2 | |||||
UPV (m/s) | RN | UPV (m/s) | RN | UPV (m/s) | RN | UPV (m/s) | RN | |
3597.1 | 42 | 4032.3 | 40.5 | 3512.9 | 42.5 | 3754.7 | 42.5 | |
3597.1 | 40 | 4087.2 | 33.5 | 3512.9 | 38 | 3731.3 | 37.5 | |
3575.7 | 41 | 4059.5 | 43.5 | 3533.6 | 41.5 | 3778.3 | 44.5 | |
3597.1 | 40.5 | 4087.2 | 38.5 | 3492.4 | 42.5 | 3754.7 | 39.5 | |
3575.7 | 41 | 4115.2 | 38 | 3533.6 | 42.5 | 3778.3 | 40.5 | |
3597.1 | 40 | 4115.2 | 37.5 | 3492.4 | 40 | 3754.7 | 37.5 | |
3597.1 | 40 | 4087.2 | 40 | 3492.4 | 48 | 3778.3 | 41.5 | |
3597.1 | 37.5 | 4115.2 | 36 | 3492.4 | 44.5 | 3778.3 | 35.5 | |
3618.8 | 41.5 | 4115.2 | 41.5 | 3492.4 | 47.5 | 3802.3 | 42 | |
3952.6 | 42 | 4115.2 | 40 | 3492.4 | 41.5 | 3802.3 | 41 | |
3952.6 | 41.5 | 3708.3 | 44 | 3802.3 | 43 | 3851.1 | 45.5 | |
3952.6 | 41 | 3708.3 | 40.5 | 3802.3 | 41 | 3876 | 41.5 | |
3952.6 | 41.5 | 3708.3 | 42.5 | 3826.5 | 44.5 | 3901.2 | 44 | |
3952.6 | 39 | 3731.3 | 39.5 | 3802.3 | 45 | 3851.1 | 45.5 | |
3952.6 | 39 | 3731.3 | 40 | 3826.5 | 46.5 | 3876 | 42 | |
3952.6 | 41.5 | 3731.3 | 41.5 | 3802.3 | 42 | 3851.1 | 44.5 | |
3952.6 | 40 | 3708.3 | 40.5 | 3826.5 | 45.5 | 3876 | 39.5 | |
3952.6 | 40 | 3731.3 | 45 | 3826.5 | 47.5 | 3876 | 45 | |
Mean | 3773.6 | 40.5 | 3927.1 | 40.1 | 3642.3 | 43.5 | 3815.1 | 41.6 |
SD | 184.32 | 1.19 | 192.09 | 2.82 | 159.05 | 2.78 | 54.15 | 2.94 |
CV (%) | 4.88 | 2.93 | 4.89 | 7.03 | 4.37 | 6.4 | 1.42 | 7.06 |
Table 7 Results of measurements and statistical parameters
Columns | ||||||||||
---|---|---|---|---|---|---|---|---|---|---|
D4 | D5 | E2 | E4 | E5 | ||||||
UPV (m/s) | RN | UPV (m/s) | RN | UPV (m/s) | RN | UPV (m/s) | RN | UPV (m/s) | RN | |
3512.9 | 38.5 | 3640.8 | 42 | 3640.8 | 43.5 | 3826.5 | 43 | 3618.8 | 42 | |
3452.2 | 41 | 3663 | 40.5 | 3640.8 | 41.5 | 3826.5 | 41.5 | 3640.8 | 38.5 | |
3472.2 | 37 | 3663 | 41.5 | 3663 | 42 | 3826.5 | 43.5 | 3640.8 | 38.5 | |
3575.7 | 37.5 | 3708.3 | 45.5 | 3640.8 | 48.5 | 3802.3 | 42 | 3640.8 | 43 | |
3554.5 | 35.5 | 3708.3 | 41.5 | 3640.8 | 43 | 3826.5 | 47.5 | 3663 | 42.5 | |
3452.2 | 37 | 3754.7 | 43.5 | 3663 | 42 | 3826.5 | 40.5 | 3685.5 | 43 | |
3492.4 | 40.5 | 3685.5 | 42 | 3663 | 43.5 | 3802.3 | 38.5 | 3663 | 40 | |
3472.2 | 38.5 | 3731.3 | 44.5 | 3663 | 38.5 | 3826.5 | 38 | 3663 | 39 | |
3512.9 | 37 | 3708.3 | 42.5 | 3663 | 43 | 3826.5 | 45.5 | 3663 | 39.5 | |
3472.2 | 37.5 | 3731.3 | 40.5 | 3663 | 43.5 | 3851.1 | 44 | 3685.5 | 38 | |
3533.6 | 43.5 | 3685.5 | 44.5 | 3778.3 | 42.5 | 3731.3 | 40.5 | 3731.3 | 43.5 | |
3533.6 | 41 | 3685.5 | 46.5 | 3778.3 | 39.5 | 3731.3 | 36.5 | 3754.7 | 45 | |
3533.6 | 43 | 3685.5 | 42 | 3708.3 | 42 | 3731.3 | 39 | 3754.7 | 45.5 | |
3554.5 | 43 | 3685.5 | 45.5 | 3754.7 | 41 | 3731.3 | 41.5 | 3754.7 | 42.5 | |
3533.6 | 42 | 3685.5 | 43 | 3778.3 | 36.5 | 3731.3 | 45 | 3778.3 | 39.5 | |
3554.5 | 42 | 3708.3 | 45 | 3640.8 | 42 | 3731.3 | 47 | 3754.7 | 43 | |
3554.5 | 45 | 3685.5 | 44.5 | 3754.7 | 38.5 | 3754.7 | 43 | 3778.3 | 38.5 | |
3554.5 | 45.5 | 3685.5 | 45.5 | 3731.3 | 43.5 | 3731.3 | 46 | 3754.7 | 43.5 | |
Mean | 3517.8 | 40.2 | 3694.5 | 43.3 | 3692.5 | 41.9 | 3784.1 | 42.3 | 3701.4 | 41.3 |
SD | 39.57 | 3.05 | 27.24 | 1.88 | 54.32 | 2.61 | 47.35 | 3.17 | 54.86 | 2.43 |
CV (%) | 1.12 | 7.58 | 0.74 | 4.33 | 1.47 | 6.22 | 1.25 | 7.48 | 1.48 | 5.88 |
Table 8 Average results of UPV and RN measurements
Column No. | ID | UPV average (m/s) | RN average |
---|---|---|---|
1 | C2 | 3773.68 | 40.50 |
2 | C4 | 3927.10 | 40.14 |
3 | C5 | 3642.37 | 43.53 |
4 | D2 | 3815.09 | 41.64 |
5 | D4 | 3517.88 | 40.28 |
6 | D5 | 3694.52 | 43.36 |
7 | E2 | 3692.55 | 41.92 |
8 | E4 | 3784.17 | 42.36 |
9 | E5 | 3701.42 | 41.39 |
Table 9 Results of the statistical analysis of the values obtained
Author | ID | n total | fc Mean | Standard Deviation | Coefficient of Variation (%) |
---|---|---|---|---|---|
RILEM | fc1 | 162 | 26.29 | 3.12 | 11.88 |
Bellander | fc2 | 53.30 | 9.12 | 17.11 | |
Meynink et al. | fc3 | 41.00 | 3.82 | 9.33 | |
Tanigawa et al. | fc4 | 26.07 | 3.50 | 13.60 | |
Raymar et al. | fc5 | 43.62 | 4.12 | 9.46 | |
Arioglu & Köylüoglu | fc6 | 36.40 | 4.89 | 13.45 | |
Kheder | fc7 | 17.99 | 1.29 | 7.18 | |
Chandak & Kumavat | fc8 | 23.67 | 1.40 | 5.91 |
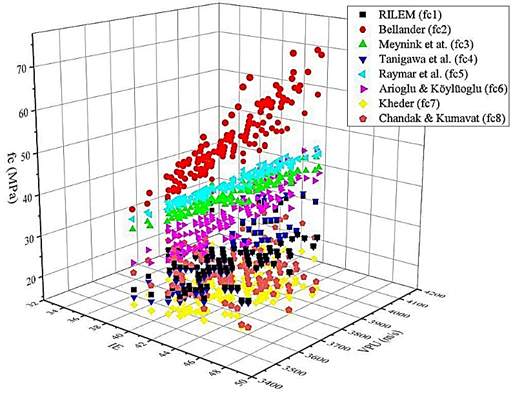
Figure 5 General distribution of the results obtained from the application of selected SonReb models
4. Conclusions
Based on the results obtained through the application of the different SonReb methods studied, for the estimation of the compressive strength of concrete produced with ordinary Cuban Portland Cement P-35 (Type 1) and domestic aggregates, it was shown that the models proposed by RILEM and Tanigawa et al can be applied to estimate, with an error of less than 4%, the fc of concrete by correlating Schmidt rebound hammer and ultrasonic pulse velocity tests.
These results can be used as a research basis for a future development of Cuban regulations that consider the use of SonReb models valid, for non-destructive evaluations of the quality of national concrete in newly built buildings, or in restoration works.