Servicios Personalizados
Revista
Articulo
Indicadores
-
Citado por SciELO
-
Accesos
Links relacionados
-
Similares en SciELO
Compartir
Revista mexicana de fitopatología
versión On-line ISSN 2007-8080versión impresa ISSN 0185-3309
Rev. mex. fitopatol vol.42 no.1 Texcoco ene. 2024 Epub 27-Ene-2025
https://doi.org/10.18781/r.mex.fit.2309-3
Review Articles
Current situation of viticulture in Costa Rica and management strategies for downy mildew (Plasmopara viticola)
1 Centro de Investigación en Estructuras Microscópicas. Vicerrectoría de Investigación. Universidad de Costa Rica. Ciudad de la Investigación, Finca 2. Sede Rodrigo Facio. Código Postal 2060 Costa Rica
2 Consultoría Agronómica Jiménez y Quesada. S.A. San Marcos de Tarrazú, Costa Rica
3 Estación Experimental Agrícola Fabio Baudrit Moreno. Escuela de Agronomía. Universidad de Costa Rica. 2 km oeste de la iglesia católica en Barrio San José, La Garita, Alajuela. Código Postal 2060. Costa Rica
Viticulture is one of the oldest agricultural activities, and its exploitation has traditionally been limited to temperate climate zones, where the european grapevine (Vitis vinifera) and wine originate. Given the effects of climate change, more areas lose the capacity to grow this crop, and the tropics are presented as potential regions for this market. In Costa Rica, viticultural activity has been reported since the mid- 20th century, however, technical information on the crop is scarce. Downy mildew, caused by the oomycete Plasmopara viticola, represents one of the diseases with the greatest economic impact for viticulture worldwide, as well as the most limiting phytosanitary problem in Costa Rica. Under high humidity conditions, the development of the pathogen is accelerated, and the host remains susceptible throughout the crop cycle, which makes proper management of epidemics difficult. Chemical control is the most common management strategy around the world, however, the appearance of P. viticola populations with resistance to fungicides has been observed in most grape vine-growing areas, hence the search for more ecological alternatives is a necessity. Currently, Costa Rica does not have integrated management strategies that allow sustainable production, and there is only one registered product for protection against this pathogen. This situation justifies paying more attention to the investigation of this pathosystem.
Keywords: Vitis vinifera; tropical viticulture; oomycete; disease management.
La viticultura es una de las actividades agrícolas de mayor antigüedad, y su ex- plotación tradicionalmente se ha limitado a zonas de clima templado, de donde la vid (Vitis vinifera) y el vino son originarios. Ante los efectos del cambio climático, cada vez más áreas pierden capacidad para el desarrollo de este cultivo, y los tró- picos se presentan como regiones potenciales para este mercado. En Costa Rica, la actividad vitícola se ha reportado desde mediados del siglo XX, sin embargo, la información técnica sobre el cultivo es escasa. El mildiú velloso, causado por el oomicete Plasmopara viticola, representa una de las enfermedades de mayor impacto económico para la viticultura a nivel global, así como el problema fitosa- nitario más limitante en Costa Rica. En condiciones de alta humedad, el desarrollo del patógeno es acelerado, y el hospedante se mantiene susceptible durante todo el ciclo de cultivo, lo cual dificulta el manejo adecuado de las epidemias. Global- mente, el combate químico es la estrategia de manejo más común; sin embargo, la aparición de poblaciones de P. viticola con resistencia a fungicidas se ha observado en la mayoría de las zonas productoras, por lo que la búsqueda de alternativas más ecológicas constituye una necesidad. En la actualidad, Costa Rica no cuenta con estrategias de manejo integrado que permitan la producción sostenible, y solo exis- te un producto registrado para la protección contra este patógeno. Esta situación justifica prestar mayor atención al estudio de este patosistema.
Palabras clave: Vitis vinifera; viticultura tropical; oomicete; manejo de enfermedades
Introduction
Vitis vinifera is the grapevine species with the greatest economic importance for viticulture worldwide, and the only one used extensively in the production of wine (This et al., 2006; Sargolzaei et al., 2021). Currently, the grape market generates roughly USD $34 billon (Sacchi, 2021). In 2018, the approximate global extension reached 7.4 million hectares, with an estimated productivity of 77.8 million tons, out of which 57 % went to wine production, 36 % for fresh consumption, and 7% for raisins (International Organization of Vine and Wine - OIV, 2019). Despite the existence of over 10 000 varieties, only 33 cover more than 50 % of the total cultivated area (IOV, 2017).
Against the variations in water and temperature regimes due to climate change, Lallanilla (2013) and Santos et al. (2020a) warn that the geographic distribution of viticulture will transform in the next 50 years. Despite the plasticity displayed by V. vinifera in its acclimatization to various weather conditions, some areas that are now considered suitable for cultivation may lose this ability, which would make the establishment and maintenance of vineyards difficult (This et al., 2006; Jacinto et al., 2023).
According to claims by Mozell and Thach (2014), it is possible for this scenario to cause a migration of grape production in North America, Europe and South America to higher and colder regions, as well as a reduction in Australia and South Africa, as a consequence of high temperatures, whereas, by contrast, China stands out as the only country with a potential for growth in this industry. This projection is a challenge for wine production, since some wine-quality criteria are related to characteristics determined by the region of origin (Mackenzie and Christy, 2005; Moriondo et al., 2013).
Vitis vinifera was introduced into Latin America in the 16th Century (Camargo et al., 2008), and viticulture has been documented for over 60 years in tropical countries. However, production in the tropics has been characterized by lower quality for fermentation, as the adaptation of varieties from temperate to tropical climates is difficult, affecting the development of the crop (Carbonneau, 2011; Camargo et al., 2012; Commins et al., 2012; Hannah et al., 2013). The main causes of these alterations are as follows:
In tropical areas, buds do not undergo dormancy, and with enough water and nutrients, grapevines grow continuously, causing excess vigor, poor lignification, heterogeneity in bud break and irregular yields between cycles (Tonietto and Pereira, 2011; Ashenfelter and Storchmann, 2014; Demir, 2014; Khalil-Ur-Rehman et al., 2017);
High temperatures throughout the plant cycle accelerate growth and induce early phenological events, as well as reducing the fertility of buds for the following cycle (Carbonneau, 2011; Demir, 2014; Leão et al., 2016);
High temperatures during the maturing of grapes lead them to produce more sugar, lower acidity and an incomplete metabolism of phenols (Tonietto and Pereira, 2011; Hickey et al., 2018; Costa et al., 2019; Fonseca et al., 2023);
Excessive vegetative growth favors the susceptibility to the attack of pathogens, and high temperature and moisture are favorable for the progress of diseases such as downy mildew (Camargo et al., 2012; Nascimento-Gavioli, et al., 2020), which demands an adequate selection of the time and system of plantation, based in the weather conditions of the location (de Bem et al., 2016).
These latitudinal effects on the development of the grapevine limit the productivity of tropical grapevines (Ashenfelter and Storchmann, 2014), and the technification of the production systems required in the production of high-quality grapes is costly (Tonietto and Pereira, 2011; Camargo et al., 2012). Nevertheless, interest in tropical vine-growing has recently increased, as plants grow continuously in the absence of a resting period, therefore, with adapted varieties, along with an appropriate management of the canopy architecture, trimming and irrigation, two or more cycles can be obtained every year (Camargo, 2005; Mosedale et al., 2016; Nassur et al., 2017).
Alterations in temperature and rainfall are also decisive factors in the distribution patterns of diseases in diverse crops, as well as having repercussions on the effectiveness of the resistance genes to these pathogens (Garrett et al., 2006; Tylianakis et al., 2008). In this regard, Leis et al. (2018) indicate that in Europe, viticulture could be threatened by an increase in the pressure of diseases, including downy mildew, caused by the oomycete Plasmopara viticola, and which is projected to increase the potential for infection by 5 to 20 % (Bregaglio et al., 2013). This disease is one of the most important economic problems for plant health in viticulture; therefore, it is crucial to have effective alternatives to face future dynamics of epidemics in diverse scenarios.
Grapevine planting in Costa Rica
In 1945, Dr. Joseph L. Fennell expressed interest in developing hybrids for wine and fresh grapes from crosses between wild grape species from tropical forests and imported varieties, that could adapt to warm and humid climates, and eventually, be grown on a large scale (Fennell, 1945; Cruz, 1948). However, it was only in the 1970s that the first commercial plantations of European varieties were established in Costa Rica, located in Playa Panamá, Guanacaste, although the susceptibility of these varieties to Pierce’s disease (Xylella fastidiosa), as well as the lack of technical information, affected the evolution of the project (Sheng-Pin, 1988).
In 1985, a cooperative program between the Ministry of Agriculture and Livestock (MAG), the Nacional Learning Institute (INA), the Agrarian Development Institute (IDA) and the Republic of China’s Technical Agriculture Mission agreed to evaluate over 75 imported grapevine varieties, in order to select the ones that best adapted to the tropical climate (Sheng-Pin, 1988). On the path towards the establishment of the Ruby Seedless cultivar as the most promising one in that moment, the program also facilitated research, dissemination, training and technical assistance, which led to the creation of informative material on the agricultural management of the grapevine in Costa Rica (Lizano-Sáenz, 1992).
After the foundation of the Grape Farmer Association of Costa Rica in 1997, the plant material was tested in other areas in Costa Rica for several years, until in 2006, Republic of China Technical Agriculture Mission left the country. After that moment, several farmers retired from the activity and even sold their farms (Cordero-Pérez, 2022). Currently, grapevine growing activities have been recorded in five provinces: Alajuela, Cartago, Guanacaste, Puntarenas and San José (Cruz, 1948; Sheng-Pin, 1988; Pymes, El Financiero, 2015; Barquero, 2016; Fernández, 2016). However, the viticultural activity is not significant, and the 2021 National Agricultural Survey 2021 (INEC, 2022) does not report data on the area or varieties of grapevines planted in the country.
Despite the lack of official data on the current state of the crop, there are records of at least 9 farmers, located in the cantons of La Garita (Alajuela), Carrillo (Guanacaste), Acosta, Curridabat, Pérez Zeledón, Puriscal and Santa María de Dota (San José) (Figure 1). Out of these plantations, only those located in La Garita and Santa María de Dota are larger than 2 ha. The latter one is located at an altitude of over 2,000 m.a.s.l., the highest and largest in the country, with over 10 ha planted with different varieties of temperate-weather grapes for the production and export of wine. The remaining vineyards are located in warm-weather areas, at an altitude equal to or lower than 1,000 m.a.s.l.; this condition allows farmers to obtain two or more harvests every year.
On a commercial level, the vineyards in Acosta, La Garita and Santa María de Dota produce and own registered wine brands. In turn, the plantation located in Curridabat serves as a laboratory for the improvement of varieties that can be adapted to the tropical climate. The other plantations represent a secondary activity for farmers, who sell the grapes for fresh consumption and may occasionally produce craft wine.
Although these precedents suggest that there are possibilities of exploiting the winegrowing activity in different areas of the country, the lack of information on this crop limits the search for new areas with a potential for the activity. In addition, the alternatives for the chemical management of diseases are scarce, since according to the database of the State Phytosanitary Service (SFE, 2023), at the moment, only two fungicides are registered for use on grapevines: myclobutanil (triazole) and mancozeb (dithiocarbamate). Out of the two, only the latter is registered for use
against downy mildew. This situation restricts the profitability of the vineyards and the projection of small-scale companies to insert themselves in the commercial chain.
In Costa Rica, all grape farmers claim that under relatively high moisture levels and temperature, diseases appear that affect their grapevines, among which downy mildew is the most common, although nobody has estimated the losses it has caused. Other reported pathologies that affect to a lower extent include Pierce’s disease (Xylella fastidiosa), rust (Phakopsora euvitis) and downy mildew (Erysiphe necator), although the latter is only found in plantations in areas with warm weather. Likewise, there have been reports of damages caused by pests such as the grape root borer (Vitacea polistiformis), maybug (Phyllophaga spp.) and the fruit fly (Ceratitis capitata).
Downy mildew in grapevine
The majority of the area planted with grapevines in the world are done so with European varieties (V. vinifera), since yields are greater and their quality is considered superior (Sun et al., 2011; OIV, 2017; Teissedre, 2018). Nevertheless, the V. vinifera varieties are highly susceptible to diseases, including downy mildew, which is why it is considered a constant threat in wine-growing regions (Gessler et al., 2011; Yu et al., 2012; Wilcox et al., 2015; Ash, 2017).
The causal agent of downy mildew, P. viticola, is an obligate biotrophic parasite (Göker et al., 2007). The taxonomic classification locates it in the Chromista kingdom, phylum Oomycota, class Peronosporea, order Peronosporales and family Peronosporaceae (Index Fungorum, 2023). Although it is not a true fungus, ecologically and epidemiologically, it has a similar behavior (Kassemeyer et al., 2015). This organism is pathogenic for at least 28 species of Vitis, out of which V. vinifera and V. labrusca are the main hosts (Rouxel et al., 2014; CABI, 2021).
Plasmopara viticola is native to North America, where the wild and cultivated varieties of American origin (V. labrusca) display different degrees of resistance to the infection as a product of a longer period of coevolution with the pathogen (Bitsadze et al., 2014; Boso et al., 2014). By importing rootstock with resistance to phylloxera (Daktulosphaira vitifoliae) from the United States, P. viticola was introduced into southwestern France in the late 1870s, and from there, it spread to the rest of the continent, causing significant losses in yields, which extended throughout the first half of the 20th century (Gessler et al., 2011; Fontaine et al., 2021; Koledenkova et al., 2022).
Downy mildew is highly destructive in warm areas with abundant rainfalls, since moisture is the main cause of epidemics (Kennelly et al., 2007; Caffi et al., 2013; Koledenkova et al., 2022). When weather conditions are favorable and agronomic management is inappropriate, this disease can cause losses of up to 100% in production (Ash, 2017; Buonassisi et al., 2017). In addition to parasitizing the plant during the entire vegetative cycle, when the infection is severe, defoliation takes place, and as a consequence, the grapes lose their commercial and nutritional value (Jermini et al., 2010; Taylor, 2021). Due to a low accumulation of reserve carbohydrates, defoliation also causes losses in the yields of the next cycles (Matasci et al., 2008; Jackson, 2022).
In temperate regions, at the beginning of the spring, when temperatures are higher than 10 ºC, relative humidity reaches higher than 95 %, and the frequency of rainfalls increases, and the oospores, which are sexual survival structures and remain on the soil and the fallen leaves, germinate if a water layer remains for more than 24 h (CABI, 2021). Through a germinative tube, a macrosporangium is formed, which contains biflagellate zoospores that, dispersed by rainwater and wind, penetrate the live plant tissue, colonize, infect and reproduce asexually, which gives rise to a secondary infection cycle (Yin et al., 2017). This cycle may be completed in 5 days (Figure 2), depending on the weather conditions and the susceptibility of the host (Agrios, 2005; Kortekamp, 2005).
In polycyclic diseases such as downy mildew, both the oospores and the zoospores are involved in the origin of new infections. The former act as a primary inoculum and the latter, the secondary inoculum (Carisse, 2016; Maddalena et al., 2021; Massi et al., 2022). In the past it was thought that only the secondary inoculum contributed to the progress of epidemics, although evidence indicates that the oospores maintain their ability to germinate and cause infections for up to three months (Vercesi et al., 2010; Gessler et al., 2011; Rossi et al., 2013).
The zoospores are released from the sporangium when moisture levels in the air is high, and remain active for a few hours. Because they have no cell wall, their survival is determined by the presence of free water (Massi et al., 2022). After they reach susceptible tissue, these cells swim in the layer of water on the leaves until they reach the stomata, where they encyst, germinate and penetrate through them, by means of the action of enzymes that degrade the cell walls of the plant. In the sub-stomatal cavity, they form a vesicle, out of which hyphae branch out and colonize the cells of the mesophyll to produce haustoria (Fröbel and Zyprian, 2019). This structure absorbs the nutrients from the cells. Kassemeyer et al. (2015) indicate that sporulation takes place under temperatures above 13 ºC, a relative humidity of 95 % and at least 4 h of darkness, which completes the latent period of the disease.
The incubation period is also influenced by temperature. On average, symptoms take between 7 and 10 days after the beginning of the infection to appear, but it may extend up to 21 days (Ash, 2017). Additionally, this event varies depending on the organ of the plant that is infected and the ontogenic resistance expressed (Steimetz et al., 2012; Rossi et al., 2013; Buonassisi et al., 2017). This period is shorter between 20-25 ºC and in new leaves, and longer in temperatures below 12 ºC and in old leaves (CABI, 2021).
Downy mildew infections take place in all the photosynthetic tissues of the plant (Bitsadze et al., 2014; Fröbel et al., 2019). The initial symptoms appear on the upper surface of new leaves as oily chlorotic spots, which become brown as the lesions age (Figure 3A). On the other hand, in older leaves, the veins demarcate these lesions, forming small angular spots that grow and coalesce until they cover the entire tissue (Rossi et al., 2013; Taylor, 2021).
In the tendrils, petioles and inflorescences, infections cause tissues to thicken and roll up, followed by necrosis (Kassemeyer et al., 2015). Likewise, Koledenkova et al. (2022) mention that in sprouts and young grape bunches, the volume of the intercellular mycelia causes a deformity and the sinking of tissues, which quickly necrotize. Young bunches are highly susceptible to infections, but as they develop, resistance to the disease increases, due to the lenticels in the epicarp blocking the penetration of the hyphae (Carisse, 2016). Nevertheless, in mature bunches, the pedicel remains susceptible and the grapes can become infected from there (Gindro et al., 2022).
Sporangiophores are white and are produced in the abaxial side of leaves, around lesions and in the rest of the infected tissues (Figures 3B and 3C). After sporulation, the proportion of necrotic tissue increases, and eventually, the abscission of the affected organs takes place, along with the total defoliation of the plant (Taylor, 2021; Jackson, 2022).
Downy mildew management
Downey mildew management traditionally (Figur3 4) involves preventive agricultural practices, such as pruning and the use of stakes to promote ventilation and the reduction of moisture in the canopy, thus preventing the production of secondary infections (Agrios, 2005; Gessler et al., 2011). In the late 19th century, Bordeaux, France, a delay in the appearance of symptoms was observed after spraying grapevines with a mixture produced from copper sulfate and calcium hydroxide. The mixture became popular in other grape-growing regions of the world,
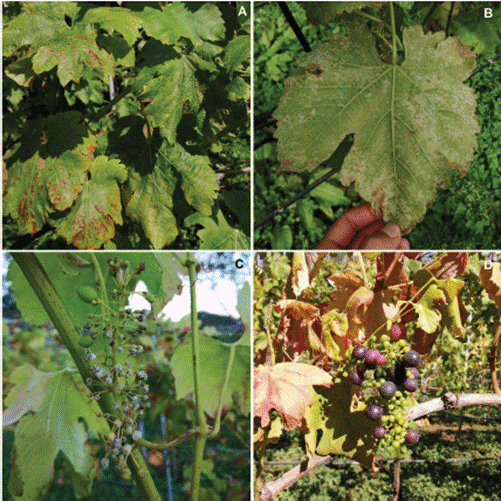
Figure 3 Symptoms and characteristic signs of downy mildew in the grapevine (Vitis vinifera), caused by the oomycete Plasmopara viticola. A. Chlorosis and foliar necrosis. B. Sporulation on the reverse of leaves with necrotic lesions. C. Sporulation on young fruits. D. Leaf necrosis and poor filling of fruits.
due to its strong adherence and persistence in plants, with the name of “Bordelais mixture.” Since then, copper salts have been used to prevent secondary infections in the spray programs, with variations in composition, dosage and application intervals depending on the pressure of the current inoculant (Lamichhane et al., 2018; Massi et al., 2021).
The scarcity of copper for agriculture during World War II led to the need to find new substances to fight downy mildew (Lamberth, 2019). In the 1970s, molecules
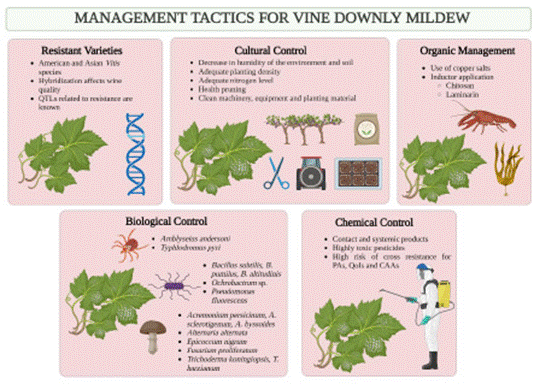
Figure 4 Tactics (advantages and disadvantages) documented for the management of downy mildew in the vine (Vitis vinifera), caused by the oomycete Plasmopara viticola.
were created to control P. viticola with the ability to act upon this organism for more days, resist washing by rains, since they could be absorbed and translocated by the plant, and cure infections during its development (Massi et al., 2021). Nowadays, these fungicides, called “systemic” fungicides, are applied globally on a wide range of crops. However, in most winegrowing regions, P. viticola populations have been found that are resistant to diverse fungicide groups, and their resistance appeared as soon as these products started to be used (Wicks et al., 2005; Baudoin et al., 2008; Furuya et al., 2010; Lucas et al., 2015; Hall et al., 2017; Zhang et al., 2017; Toffolatti et al., 2018b; Campbell et al., 2020; Ghule et al., 2020; Santos et al., 2020b; Campbell et al., 2021; Massi et al., 2021).
To date there are 12 groups of fungicides available to control phytopathogenic oomycetes (Table 1), yet for the three most widely used-phenylamides (PAs), Quinone Outside Inhibitors (QoIs) and carboxylic acid amides (CAAs)-more cases of resistance have been reported for P. viticola and other downy mildew (Gisi and Sierotzki, 2015). Poor practices associated with the repeated application of
Table 1 List of active ingredients available for oomycete control, divided according to the FRAC mode of action and chemical group, modified from Hollomon
FRAC Mode of Action | Group name | Chemical or biological group | Common name (active ingredient) |
---|---|---|---|
Nucleic acids metabolism | PAs (PhenylAmides) | Acylalanines | Metalaxyl, Mefenoxam, Benalaxyl, Kiralaxyl |
Oxazolidinones | Oxadixyl | ||
Unknown | Cyanoacetamide-oxime | Cyanoacetamide-oxime | Cymoxanil |
Cytoskeleton and motor proteins | Benzamides | Pyridinylmethyl-benzamides Toluamides | Fluopicolide Zoxamide |
Thiazole carboxamide | Ethylamino-thiazole-carboxamide | Ethaboxam | |
Cell wall biosynthesis | CAAs (Carboxylic Acid Amides) | Cinnamic acid amides | Dimethomorph, Flumorph |
Mandelic acid amides | Mandipropamid | ||
Valinamide carbamates | Iprovalicarb, Benthiavalicarb | ||
Respiration | QoIs (Quinone outside Inhibitors) | Oxazolidine-diones | Famoxadone |
Imidazolinones | Fenamidone | ||
Methoxy-acrylates | Azoxystrobin | ||
QiIs (Quinone inside Inhibitors) | Cyano-imidazole | Cyazofamid | |
Sulfamoyl-triazole | Amisulbrom | ||
2,6- dinitro-anilines | Fluazinam | ||
QoSIs (QoI, stigmetallin binding type) | Triazolo-pyrimidylamine | Ametoctradine | |
Multisite | Dithio-carbamates | Dithio-carbamates | Mancozeb |
Chloronitriles/Phthalonitriles | Chloronitriles | Chlorothalonil | |
Inorganic | Inorganic | Copper (salts) | |
Plant defence induction | Phosphonates | Ethyl phosphonates | Fosetyl-Al |
these fungicides accelerate this phenomenon and lead to the loss of effectiveness of commercial products. This situation not only hinders the progressive reduction of resistant populations, but also prevents the mitigation of the progress of the disease once it has been established (Hollomon, 2015).
Along with the economic cost implied by fighting against downy mildew, estimated by Taylor and Cook (2018) at USD $5 million per year, the resistance to fungicides causes the number of effective products for the management of this disease to decrease over time. Plasmopara viticola is described as an organism with a high risk of developing resistance, due to the high rate of asexual and sexual reproduction, as well as to the polycyclic behavior of epidemics (Toffolatti et al., 2011; Fungicide Resistance Action Committee [FRAC], 2019). The risk of resistance is higher for fungicides that act on a single biochemical site (Gisi and Sierotzki, 2008; Massi et al., 2021). However, multiple applications with these products are carried out every year, despite some studies concluding that curative control is not effective to contain the progress of the downy mildew epidemics (Hollomon, 2015; Massi et al., 2021; Poeydebat et al., 2022).
Astrategy recommended for the management of resistance consists of diversifying compounds with different mode of action in the applications. It is advised that the systemic action fungicides be combined with those that act on a multisite level, thus reducing the selection of resistant populations to the molecule with the highest risk (Brent and Hollomon, 2007; van den Bosch, 2014; Kassemeyer et al., 2015; Elderfield et al., 2018). Alongside this, the use of mixtures of fungicides encourage the potential synergism between molecules, which enhances a more prolonged residual effect (Gisi, 1996; van de Bosch, 2014).
The relative damage caused by this disease to viticulture activities exceeds that caused by other downy mildews in their respective hosts (Gisi and Sierotzki, 2008). This makes chemical control the most widely used management practice in the world to ensure optimal yields (Toffolatti et al., 2018a; Massi et al., 2021), positioning viticulture as one of the agricultural activities with the highest consumption of pesticides; in Europe alone, over 70 % of the fungicide market is dedicated to this crop (Muthmann and Nadin, 2007; Sargolzaei et al., 2020; Wingerter et al., 2021). This scenario underscores the discussion around the negative impact of pesticides in health and the environment, in such a way that modern practices must include more sustainable management practices (Romanazzi et al., 2016; Yu et al., 2022).
In order to reduce the chemical load on conventional systems, other approaches have arisen for the integrated management of this disease, in which greater importance has been given to the innate resistance of wild American Vitis species (V. labrusca, V. rotundifolia, V. rupestris, V. riparia amd V. cinerea), as well as Asian ones (V. amurensis, V. piasezkii and V. coignetiae), which display partial or total resistance to the disease, conferred by multiple resistance genes (R) (Merdinoglu et al., 2018; Koledenkova et al., 2022). Although these varieties are not planted for the wine market, the existence of this germplasm is a valuable resource for breeding programs that focus on generating materials with resistant to downy mildew and other biotic and abiotic stress factors (Moreira et al., 2011; Yu et al., 2012; Toffolatti et al., 2016; Buonassisi et al., 2017).
The development of varieties with genetic resistance is the most sustainable alternative to control grapevine diseases and reduce the use of pesticides (Mundt, 2014; Zini et al., 2019; Fröbel et al., 2019). However, conventional breeding techniques are costly and the observation of results may take up to 30 years (Eibach and Töpfer, 2015; Sargolzaei et al., 2020).
The need to introduce R genes from wild species into cultivated grapevines has led, since the early 20th century, to the development and registration of thousands of hybrids with a greater resistance to the disease (Pacifico et al., 2013). However, during hybridization, wild parents segregate characteristics that modify the chemical properties of the grapes, which translates into a low-quality wine. Due to this, most of these hybrids have been discarded from the market (Eibach and Töpfer, 2015; Pertot et al., 2017; Toffolatti et al., 2018a; Foria et al., 2022).
To overcome the difficulties related to the duration of conventional breeding and to the value of wines made from hybrids, the use of molecular markers has helped detect the main QTLs (quantitative trait loci) of wild plants associated with the different levels of resistance to downy mildew, which makes it easier to evaluate the genotypes harboring the R genes (Zyprian et al., 2016; Merdinolgu et al., 2018; Sargolzaei et al., 2020). By mapping the QTLs that intervene to a greater and lesser degree to resistance, significant associations can be found between molecular markers and the phenotypes resistant to this disease (Divilov et al., 2018; Possamai and Wiedemann-Merdinoglu, 2022). This knowledge is the basis for the marker- assisted selection, which has helped reduce the breeding process considerably, since the candidate varieties that not only combine diverse resistance factors, but also important agronomic characteristics such as quality and yield can be identified, as far back as the seedling stage (Fischer et al., 2004; Eibach and Töpfer, 2015; Merdinolgu et al., 2018; Fu et al., 2020).
Current agronomic practices for the integrated management of this disease consider the relation between the weather variables and the progress of epidemics, in order to identify between climate variables and other critical moments that require applications (Caffi et al., 2011; Gessler et al., 2011). By recording the temperature, relative moisture, rainfalls and leaf wetting, prediction models can be created for these epidemics. These predictions are integrated into alert systems to help make decisions during the moments of greatest susceptibility, with the aim of optimizing the effect of the applications to the fullest (Madden et al., 2000; Dalla Marta et al., 2005; Rossi et al., 2013; Brischetto et al., 2021).
Regarding cultural management strategies, it is advisable to control moisture, both at soil and canopy levels (Thind et al., 2004; Mian et al., 2021), use clean machinery and vegetative material, plant at an optimal density, and maintain a balanced level of nitrogen (Taylor, 2021). In agroecological and organic systems, as an alternative to copper, inducers are used to promote the activation of the defense system of the plant, prior to infection events. The preventive application of these substances helps reduce the use of fungicides, since it takes advantage of the early induction of resistance responses (Guerreiro et al., 2016; Jacquens et al., 2022).
Among the most widely studied inducers, it has been proven that chitosan, when applied preventively, reduces severity in grapevine leaves at greenhouse (Aziz et al., 2006; Llamazares De Miguel et al., 2022) and field levels (Vitalini et al., 2020; Taibi et al., 2022; Mian et al., 2023), through an increase in the accumulation of salicylic acid and phytoalexins in tissues, as well as an overexpression of genes for the synthesis of pathogenesis-related proteins (PR) (Aziz et al., 2006; Inchaya et al., 2013; Mian et al., 2023). On the other hand, Romanazzi et al. (2021) and Vitalini et al. (2020) determined that different individual chitosan formulations, or mixed with copper, help reduce the incidence and severity in leaves and grape bunches against high and low pressures of the disease in commercial grapevines.
Similar effects to those produced with chitosan have been reported with the use of laminarin, a glucan derived from the algae Laminaria digitata. The application of this substance promotes the expression of defense genes and PR proteins (Aziz et al., 2003; Gauthier et al., 2014). Likewise, its efficiency for the control of downy mildew in the greenhouse and field has been proven in individual applications or combined with copper (Paris et al., 2016; Romanazzi et al., 2016; Taibi et al., 2023).
The induction of these resistance mechanisms has also been found when using β- aminobutyric acid (BABA), whose effect on the disease has been observed in controlled environments (Hamiduzzaman et al., 2005; Slaughter et al., 2008; Dagostin et al., 2011) and on the field (Reuveni et al., 2001), and benzothiadiazole (BTH), a synthetic compound, analogous to salicylic acid, which favors the synthesis of phytoalexins (Dufour et al., 2012; Burdziej et al., 2021) and helps reduce the incidence and severity of the disease in greenhouse conditions (Dagostin et al., 2006; Perazzolli et al., 2008; Harm et al., 2011). However, only BTH exists as a commercial product (Bion® 50 WG, Syngenta).
The action of endophytic fungi as potential biocontrol agents has also gained greater importance. Individuals belonging to Acremonium sp., A persicinum, A. sclerotigenum, A. byssoides y Alternaria alternata have been identified, and their metabolites in in vitro conditions display anti-germinative activity on the sporangia of P. viticola (Assante et al., 2005; Musetti et al., 2006; Arnone et al., 2008; Lo Piccolo et al., 2015). On the other hand, through mechanisms such as hyperparasitism and enzymatic lysis, Epicoccum nigrum and Fusarium proliferatum, respectively, have displayed control over P. viticola, in vitro (Bakshi et al., 2001; Kortekamp, 1997; Shen et al., 2017).
Likewise, endophytic bacterial strains such as Bacillus subtilis, B. pumilus, B. altitudinis, Ochrobactrum sp. and Pseudomonas fluorescens have been effective in reducing the impact of downy mildew in the greenhouse and the field (Furuya et al., 2011; Zhang et al., 2017; Lakkis et al., 2019; Zang et al., 2020; Zeng et al., 2021). Other non-endophytic organisms on which there have been reports of control over P. viticola include Trichoderma koningiopsis and commercial formulations with T. harzianum T9, which also have the effect of resistance induction (Perazzolli et al., 2008; Kamble et al., 2021; Palmieri et al., 2021; Küpper et al., 2022). In another study, Bolzonello et al. (2023) found that, when preventively using synthetic analogs of secondary metabolites of Trichoderma spp. on leaf discs, P. viticola cells display membrane rupture and cytoplasmic granulation, which represented a similar level of protection to a copper-based fungicide.
Regarding bacteria, Lysobacter capsici, AZ78, Streptomyces atratus PY-1 and S. viridosporus HH1 were established as reducers of the severity of the disease (Puopolo et al., 2014; Liang et al., 2016; El-Sharwaky et al., 2018; Brescia et al., 2021; Markellou et al., 2022). On the other hand, Pozzebon and Duso (2008) recorded that the mites Amblyseius andersoni and Typhlodromus pyri feed off P. viticola mycelia and spores, which makes them candidates for biological control.
Problem and management in Costa Rica
Out of all the phytosanitary problems of the vineyards in Costa Rica, downy mildew is the most limiting, causing losses that, although not estimated, have been observed in different magnitudes. For their management, winegrowers carry out suckering, constant trimming and apply copper salts and mancozeb as a prevention measure, since chemical control is restricted to a single active ingredient. Likewise, when the presence of inoculum is high, the most affected leaves are cleansed and removed from the plantation.
In the case of farmers that have materials that descend from V. labrusca in their vineyards, downy mildew does not represent a significant problem. Among them, the hybrid Isabella (V. labrusca × V. vinifera) is the most common in warm climate vineyards, both for its tolerance to the disease and for being the one that has adapted best to tropical conditions. Since the activity in Costa Rica is incipient, no molecular breeding techniques have been implemented to develop materials that resist downy mildew, nor have there been reports on the use of effective biological controllers of this pathogen.
With the exception of the hybrid Isabella, in most of the varieties planted in the country, this disease generates losses in productivity. Among them, the variety Syrah is highly susceptible, although it has also stood out as one of the temperate climate cultivars with the highest potential for wine production in tropical areas (Camargo et al., 2011; Tonietto and Pereira, 2011; Commins et al., 2012; Wurz et al., 2017). This variety is planted only in the most technified vineyard in the country, located in Dota, and it is considered high-quality for the production of wine. For all these reasons, it is important to elucidate management strategies that reduce the impact of epidemics.
An obstacle for the production of Syrah in Dota is the delay in growth, in comparison with the phenological cycle observed in temperate regions (Serrano- Segura, 2020, personal communication). This condition causes the vegetative period to be more prolonged, and therefore, for the pathogen to reproduce for longer. Alongside this, the climatic conditions of the place allow for the fulfillment of the 3-10 rule for primary infections of grapevine downy mildew, according to Rossi et al. (2013): 10 cm of leaf tissue, at least 10 mm of rain in the last 48 h, and more than 10 ºC.
As part of the agronomic management for Syrah, after harvesting, the plants remain with little maintenance while the leaves translocate the remaining nutrients to the trunk, so they accumulate the necessary reserves for the budding of the following cycle, after which a high dose of ammonium nitrate is applied as a burner. Later, the productive branches of the previous period are trimmed to give rise to the productive offspring of the following cycle. Next, cold compensation is applied to break the dormancy of the lateral buds which, approximately one year later, will bud and begin a new crop cycle, as well as a pathogen cycle.
In the Dota vineyard, a wide variety of contact, translaminar and systemic fungicides have been evaluated to determine their efficiency against the disease. However, the efficiency of some active ingredients has decreased with time. Copper- based contact products have been observed to be ineffective in the rainy seasons, whereas some tested systemic fungicides have caused toxicity at commercial or lower doses. On the other hand, products formulated with B. subtilis have not given adequate results, either, according to visual estimations in the progress of the epidemic.
The lack of new permitted active ingredients in Costa Rica for the management of this disease is an obstacle, not only for the production of Syrah, but also for the other varieties planted in Dota and the rest of the country. Currently, out of the 25 available active ingredients in the world for the control of phytopathogenic oomycetes (Table 1), in Costa Rica, only mancozeb is permitted for use against the grapevine downy mildew. Although this fungicide is used in all vineyard-producing areas, except for the European Union (Debelder, 2020), its individual use in an spray program is not enough against epidemics in Dota, where, additionally, climate conditions favor the accelerated development of the disease. For these reasons, it is appropriate to determine the effect of new molecules and mixtures that may eventually be registered for use on grapevines.
During the period of 2019 and 2020, the best result in a vineyard, in terms of yield, against downy mildew in the Syrah variety was obtained by applying a cyazofamid-based fungicide. Nevertheless, this molecule is only registered for the control of Phytophthora infestans in potato and tomato, and Pseudoperonospora cubensis in cantaloupe and watermelon, two phytopathogenic oomycetes of great economic importance for agriculture.
In an investigation carried during the 2020-2021 production cycle (data not published), both cyazofamid and the mixture of cymoxanil + fosetyl-Al + mancozeb were determined to be effective in reducing the impact of the disease. These results show the importance of performing biological efficacy tests for molecules that have not been evaluated in this pathosystem, which provide an approach towards new registrations, with the aim of continuing to search for strategies that can help establish an integrated and sustainable management of the activity.
Conclusions
The changes in the global distribution of viticulture have awaken interest in considering tropical areas as potential regions in which to carry out this activity. However, the temperature and moisture conditions of the tropics favor the appearance and damage caused by downy mildew and other diseases. On the other hand, the resistance of P. viticola to diverse fungicides, along with the transition towards more agroecologically sustainable markets, are challenges for current conventional systems, which must place their efforts on integrated management strategies that prioritize the use of resistant varieties and prevention practices, in order to reduce and optimize the use of synthetic fungicides.
In Costa Rica, the lack of technical information on the agronomic management of grapevines and of phytosanitary resources against downy mildew, are obstacles in the progress of the existing grapevine production activity. Despite this, the diversity found in the areas of the country with vineyards shows that there is a possibility of developing this market, which is why it is important to explore forms of phytosanitary management. In order to determine effective strategies in the fight against this disease, it is necessary to evaluate the behavior of the reported biocontrol agents, as well as of new synthetic molecules in a tropical environment such as Costa Rica, to identify the inputs with best efficacy.
Literatura Citada
Agrios, GN (2005). Plant Pathology. 5th Ed. Academic Press, New York, USA. 922p. [ Links ]
Ash, G (2017). Downy mildew of grape. The American Phytopathological Society. https://www.apsnet.org/edcenter/disandpath/oomycete/pdlessons/Pages/DownyMildewGrape.aspx [ Links ]
Arnone, A , Assante, G , Bava, A , Dallavalle, S y Nasini, G (2009). Acremines H-N, novel prenylated polyketide metabolites produced by a strain of Acremonium byssoides. Tetrahedron, 65(4), 786-791. https://doi.org/10.1016/j.tet.2008.11.058 [ Links ]
Assante, G , Dallavalle, S , Malpezzi, L , Nasini, G , Burruano, S y Torta, L (2005). Acremines A-F, novel secondary metabolites produced by a strain of an endophytic Acremonium, isolated from sporangiophores of Plasmopara viticola in grapevine leaves. Tetrahedron, 61, 7686-7692. https://doi.org/10.1016/j.tet.2005.05.094 [ Links ]
Ashenfelter, O y Storchmann, K (2014). Wine and climate change. The American Phytopathological Society. https://ageconsearch.umn.edu/record/164854/ [ Links ]
Aziz, A , Poinssot, B , Daire, X , Adrian, M , Bézier, A , Lambert, B , Joubert, JM y Pugin, A (2003). Laminarin elicits defense responses in grapevine and induces protection against Botrytis cinerea and Plasmopara viticola. Molecular Plant-Microbe Interactions, 16(12), 1118-1128. https://doi.org/10.1094/MPMI.2003.16.12.1118 [ Links ]
Aziz, A , Trotel-Aziz, P , Dhuicq, L , Jeandet, P , Couderchet, M y Vernet, G (2006). Chitosan oligomers and copper sulfate induce grapevine defense reactions and resistance to gray mold and downy mildew. Phytopathology, 96(101), 1188-1194. https://doi.org/10.1094/PHYTO-96-1188 [ Links ]
Barquero, M. (2 de mayo de 2016). Productores buscan llevar uva a niveles comerciales. La Nación. https://www.nacion.com/economia/agro/productores-buscan-llevar-uva-a-niveles-comerciales/2EQKCZVJJFBFBPTN6ZTSWUJNH4/story/ [ Links ]
Bakshi, S , Sztejnberg, A y Yarden, O (2001). Isolation and characterization of a cold-tolerant strain of Fusarium proliferatum, a biocontrol agent of grape downy mildew. Phytopathology, 91, 1062-1068. https://doi.org/10.1094/PHYTO.2001.91.11.1062 [ Links ]
Baudoin, A , Olaya, G , Delmotte, F , Colcol, JF y Sierotzki, H (2008). QoI resistance of Plasmopara viticola and Erysiphe necator in the mid-Atlantic United States. Plant Health Progress, 9(1), 25. https://doi.org/10.1094/PHP-2008-0211-02-RS [ Links ]
Bitsadze, N , Chipashvili, R , Tskhvedadze, L , Aznarashvili, M , Maghradze, D y Failla, O (2014). Screening of the Georgian grape germplasm to susceptibility of downy mildew: preliminary results. Acta Horticulturae, 1032(191), 6. [ Links ]
Bolzonello, A , Morbiato, L , Tundo, S , Sella, L , Baccelli, I , Echeverrigaray, S , Musetti, R , De Zotti, M y Favaron, F (2023). Peptide analogs of a Trichoderma peptaibol effectively control downy mildew in the vineyard. Plant Disease, 107(9). https://doi.org/10.1094/PDIS-09-22-2064-RE [ Links ]
Boso, S , Alonso-Villaverde, V , Gago, P , Santiago, JL y Martínez, MC (2014). Susceptibility to downy mildew (Plasmopara viticola)of different Vitis varieties. Crop Protection, 63, 26-35. https://doi.org/10.1016/j.cropro.2014.04.018 [ Links ]
Bregaglio, S , Donatelli, M y Confalonieri, R (2013). Fungal infections of rice, wheat, and grape in Europe in 2030-2050. Agronomy for Sustainable Development, 33, 767-776. https://doi.org/10.1007/s13593-013-0149-6 [ Links ]
Brent, KJ y Hollomon, DW (2007). Fungicide resistance in crop pathogens: how can it be managed? FRAC Monograph No. 1. 2nd Ed. [ Links ]
Brescia, F , Vlassi, A , Bejarano, A , Seidl, B , Marchetti-Deschmann, M , Schuhmacher, R y Puopolo, G (2021). Characterisation of the antibiotic profile of Lysobacter capsici AZ78, an effective biological control agent of plant pathogenic microorganisms. Microorganisms, 9(6): 1320. https://doi.org/10.3390/microorganisms9061320 [ Links ]
Brischetto, C , Bove, F , Fedele, G y Rossi, V (2021). A weather-driven model for predicting infections of grapevines by sporangia of Plasmopara viticola. Frontiers in Plant Science, 12: 636607. https://doi.org/10.3389/fpls.2021.636607 [ Links ]
Buonassisi, D , Colombo, M , Migliaro, D , Dolzani, C , Peressotti, E , Mizzotti, C , Velasco, R , Masiero, S, Perazzolli, M y Vezzulli, S (2017). Breeding for grapevine downy mildew resistance: a review of “omics” approaches. Euphytica, 213(5): 1-21. https://doi.org/10.1007/s10681-017-1882-8 [ Links ]
Burdziej, A , Bellée, A , Bodin, E , Valls Fonayet, J , Magnin, N , Szakiel, A , Richard, T y Corio-Costet, MF (2021). Three types of elicitors induce grapevine resistance against downy mildew via common and specific immune responses. Journal of Agricultural and Food Chemistry, 69(6), 1781-1795. https://doi.org/10.1021/acs.jafc.0c06103 [ Links ]
Burger, P , Bouquet, A y Striem, MJ (2009). Grape breeding. En: Mohan, JS y Priyadarhan, PM (eds.). Breeding Plantation Tree Crops: Temperate Species. Springer Verlag, Berlin, Germany, 161-189. [ Links ]
CABI (2021). Plasmopara viticola (grapevine downy mildew). CABI Invasive Species Compendium. https://www.cabi.org/isc/datasheet/41918 [ Links ]
Caffi, T , Rossi, V y Carisse, O (2011). Evaluation of a dynamic model for primary infections caused by Plasmopara viticola on grapevine in Quebec. Plant Health Progress, 12(1): 22. https://doi.org/10.1094/PHP-2011-0126-01-RS [ Links ]
Caffi, T , Gilardi, G , Monchiero, M y Rossi, V (2013). Production and release of asexual sporangia in Plasmopara viticola. Phytopathology, 103(1): 64-73. https://doi.org/10.1094/PHYTO-04-12-0082-R [ Links ]
Camargo, UA (2005). Grape management techniques in tropical climates. En: XIV International GESCO Viticulture, Geisenheim, Alemania. [ Links ]
Camargo, UA , Protas, JFS y Mello, LMR (2008). Grape growing and processing in Brazil. Acta Horticulturae, 785: 51-58. https://doi.org/10.17660/ActaHortic.2008.785.2 [ Links ]
Camargo, UA , Pereira, GE y Guerra, CC (2011). Wine grape cultivars adaptation and selection for tropical regions. En: II International Symposium on Tropical Wines, Petrolina, Brazil. https://www.ishs.org/symposium/228 [ Links ]
Camargo, UA , Mandelli, F , Conceição, MAF y Tonietto, J (2012). Grapevine performance and production strategies in tropical climates. Asian Journal of Food and Agro-Industry, 5(4): 257-269. [ Links ]
Campbell, SE , Brannen, PM , Scherm, H y Brewer, MT (2020). Fungicide sensitivity survey of Plasmopara viticola populations in Georgia vineyards. Plant Health Progress, 21(4): 256-261. https://doi.org/10.1094/PHP-05-20-0039-RS [ Links ]
Campbell, SE , Brannen, PM , Scherm, H, Eason, N y MacAllister, C (2021). Efficacy of fungicide treatments for Plasmopara viticola control and occurrence of strobilurin field resistance in vineyards in Georgia, USA. Crop Protection, 139: 105371. https://doi.org/10.1016/j.cropro.2020.105371 [ Links ]
Carbonneau, A (2011). Tropical viticulture: Specificities and challenges for a quality viticulture. En: II International Symposium on Tropical Wines, Petrolina, Brazil. https://doi.org/10.17660/ActaHortic.2011.910.1 [ Links ]
Carisse, O (2016). Development of grape downy mildew (Plasmopara viticola) under northern viticulture conditions: influence of fall disease incidence. European Journal of Plant Pathology, 144: 773-783. https://doi.org/10.1007/s10658-015-0748-y [ Links ]
Commins, T , Asavasanti, S y Deloire, A (2012). What is tropical wine and what defines it? Thailand as a case study. Asian Journal of Food and Agro-Industry, 5(02): 79-95. [ Links ]
Cordero-Pérez, C (12 de marzo de 2022). Vicosa es la creadora del vino nacional Teber y ahora fermenta cuatro nuevos productos desde La Garita. https://www.elfinancierocr.com/tecnologia/vicosa-es-la-creadora-del-vino-nacional-teber-y/2IOR7WVWRJBRXGYSRZQFMM2UJE/story/ [ Links ]
Costa, RRD , Rodrigues, AAM , Vasconcelos, VAFD, Costa, JPD y Lima, MACD (2019). Trellis systems, rootstocks and season influence on the phenolic composition of ‘Chenin Blanc’ grape. Scientia Agricola, 77(3): e20180207. https://doi.org/10.1590/1678-992X-2018-0207 [ Links ]
Cruz, L (1948). La uva y sus posibilidades de cultivo a grande escala en Costa Rica. http://www.mag.go.cr/rev-histo/ra-20-01-007.pdf [ Links ]
Dagostin, S , Vecchione, A, Zulini, L, Ferrari, A y Pertot, I (2006). Efficacy evaluation of the resistance inducer benzothiadiazole against grapevine downy mildew. En: 5th International Workshop on Grapevine Downy and Powdery Mildew Proceedings, 29-30. [ Links ]
Dagostin, S , Schärer, HJ, Pertot, I y Tamm, L (2011). Are there alternatives to copper for controlling grapevine downy mildew in organic viticulture?. Crop Protection, 30(7): 776-788. https://doi.org/10.1016/j.cropro.2011.02.031 [ Links ]
Dalla Marta, A , Magarey, RD y Orlandini, S (2005). Modelling leaf wetness duration and downy mildew simulation on grapevine in Italy. Agricultural and Forest Meteorology, 132(1-2): 84-95. https://doi.org/10.1016/j.agrformet.2005.07.003 [ Links ]
de Bem, BP , Bogo, A, Everhart, SE, Casa, RT, Gonçalves, MJ, Filho, JLM, Rufato, L, da Silva, FN, Allebrandt, R y da Cunha, IC (2016). Effect of four training systems on the temporal dynamics of downy mildew in two grapevine cultivars in southern Brazil. Tropical Plant Pathology, 41: 370-379. https://doi.org/10.1007/s40858-016-0110-8 [ Links ]
Debelder, T (2020). Mancozeb Non-Renewal and MRL Review. Voluntary Report E42020-0099. Foreign Agricultural Service. United States Department of Agriculture. https://www.fas.usda.gov/data/european-union-mancozeb-non-renewal-and-mrl-review [ Links ]
Demir, KOK (2014). A review on grape growing in tropical regions. Turkish Journal of Agricultural and Natural Sciences, 6: 1236-1241. https://dergipark.org.tr/en/pub/turkjans/issue/13310/160891 [ Links ]
Divilov, K , Barba, P, Cadle-Davidson, L y Reisch, BI (2018). Single and multiple phenotype QTL analyses of downy mildew resistance in interspecific grapevines. Theoretical and Applied Genetics, 131: 1133-1143. https://doi.org/10.1007/s00122-018-3065-y [ Links ]
Dufour, MC , Lambert, C, Bouscaut, J, Mérillon, JM y Corio-Costet, MF (2012). Benzothiadiazole-primed defense responses and enhanced differential expression of defense genes in Vitis vinifera infected with biotrophic pathogens Erysiphe necator and Plasmopara viticola. Plant Pathology, 62(2): 370-382. https://doi.org/10.1111/j.1365-3059.2012.02628.x [ Links ]
Eibach, R y Töpfer, R (2015). Traditional grapevine breeding techniques. En: Reynolds, A (ed.). Grapevine breeding programs for the wine industry. Cambridge, UK: Woodhead Publishing, 3-22. [ Links ]
Elderfield, JA , López-Ruiz, FJ, van den Bosch, F y Cunniffe, NJ (2018). Using epidemiological principles to explain fungicide resistance management tactics: Why do mixtures outperform alternations?. Phytopathology, 108(7): 803-817. https://doi.org/10.1094/PHYTO-08-17-0277-R [ Links ]
El-Sharkawy, HHA, Abo-El-Wafa, TSA y Ibrahim, SAA (2018). Biological control agents improve the productivity and induce the resistance against downy mildew of grapevine. Journal of Plant Pathology, 100: 33-42. https://doi.org/10.1007/s42161-018-0007-0 [ Links ]
Fennell, J (1945). La uva tropical. Revista del Instituto de Defensa del Café de Costa Rica, Tomo XV, 128-129. https://www.sinabi.go.cr/biblioteca%20digital/revistas/Revista%20del%20Instituto%20de%20Defensa%20del%20Cafe.aspx [ Links ]
Fernández, E (14 de agosto de 2016). Vinos costarricenses luchan por sobrevivir en el mercado dominado por la importación. https://www.elfinancierocr.com/negocios/vinos-costarricenses-luchan-por-sobrevivir-en-el-mercado-dominado-por-la-importacion/E2F5VXHXYFDMNDEPKPSGXCFCG4/story/ [ Links ]
Fischer, BM, Salakhutdinov, I, Akkurt, M, Eibach, R, Edwards, KJ, Töpfer, R y Zyprian, EM (2004). Quantitative trait locus analysis of fungal disease resistance factors on a molecular map of grapevine. Theoretical and Applied Genetics, 108: 501-515. https://doi.org/10.1007/s00122-003-1445-3 [ Links ]
Fonseca, A, Fraga, H y Santos, JA (2023). Exposure of Portuguese viticulture to weather extremes under climate change. Climate Services, 30: 100357. https://doi.org/10.1016/j.cliser.2023.100357 [ Links ]
Fontaine, MC, Labbé, F, Dussert, Y, Delière, L, Richart-Cervera, S, Giraud, T y Delmotte, F (2021). Europe as a bridgehead in the worldwide invasion history of grapevine downy mildew, Plasmopara viticola. Current Biology, 31(10): 2155-2166. https://doi.org/10.1016/j.cub.2021.03.009 [ Links ]
Foria, S, Magris, G, Jurman, I, Schwope, R, De Candido, M, De Luca, E, Ivanišević, D, Morgante, M y Di Gaspero, G (2022). Extent of wild-to-crop interspecific introgression in grapevine (Vitis vinifera) as a consequence of resistance breeding and implications for the crop species definition. Horticulture Research, 9: uhab010. https://doi.org/10.1093/hr/uhab010 [ Links ]
Fungicide Resistance Action Committee (2019). FRAC Pathogen Risk List. https://www.frac.info/docs/default-source/publications/pathogen-risk/frac-pathogen-list-2019.pdf [ Links ]
Fröbel, S y Zyprian, E (2019). Colonization of different grapevine tissues by Plasmopara viticola—a histological study. Frontiers in Plant Science, 10: 951. https://doi.org/10.3389/fpls.2019.00951 [ Links ]
Fröbel, S, Dudenhöffer, J, Töpfer, R y Zyprian, E (2019). Transcriptome analysis of early downy mildew (Plasmopara viticola) defense in grapevines carrying the Asian resistance locus Rpv10. Euphytica, 215(2): 28. https://doi.org/10.1007/s10681-019-2355-z [ Links ]
Fu, P, Wu, W, Lai, G, Li, R, Peng, Y, Yang, B, Wang, B, Yin, L, Qu, J, Song, S y Lu, J (2020). Identifying Plasmopara viticola resistance loci in grapevine (Vitis amurensis) via genotyping-by-sequencing-based QTL mapping. Plant Physiology and Biochemistry, 154: 75-84. https://doi.org/10.1016/j.plaphy.2020.05.026 [ Links ]
Furuya, S, Mochizuki, M, Saito, S, Kobayashi, H, Takayanagi, T y Suzuki, S (2010). Monitoring of QoI fungicide resistance in Plasmopara viticola populations in Japan. Pest Management Science, 66(11): 1268-1272. https://doi.org/10.1002/ps.2012 [ Links ]
Furuya, S, Mochizuki, M, Aoki, Y, Kobayashi, H, Takayanagi, T, Shimizu, M y Suzuki, S (2011). Isolation and characterization of Bacillus subtilis KS1 for the biocontrol of grapevine fungal diseases. Biocontrol Science and Technology, 21(6): 705-720. https://doi.org/10.1080/09583157.2011.574208 [ Links ]
Garrett, KA, Dendy, SP, Frank, EE, Rouse, MN y Travers, SE (2006). Climate change effects on plant disease: genomes to ecosystems. Annual Review of Phytopathology, 44: 489-509. https://doi.org/10.1146/annurev.phyto.44.070505.143420 [ Links ]
Gauthier, A, Trouvelot, S, Kelloniemi, J, Frettinger, P, Wendehenne, D, Daire, X, Joubert, JM, Ferrarini, A, Delledonne, M, Flors, V y Poinssot, B (2014). The sulfated laminarin triggers a stress transcriptome before priming the SA-and ROS-dependent defenses during grapevine’s induced resistance against Plasmopara viticola. PLoS One, 9(2): e88145. https://doi.org/10.1371/journal.pone.0088145 [ Links ]
Gessler, C, Pertot, I y Perazzolli, M (2011). Plasmopara viticola: A review of knowledge on downy mildew of grapevine and effective disease management. Phytopathologia Mediterrana, 50(1): 3-44. https://www.jstor.org/stable/26458675 [ Links ]
Ghule, MR, Sawant, IS, Sawant, SD y Saha, S (2020). Resistance of Plasmopara viticola to multiple fungicides in vineyards of Maharashtra, India. Journal of Environmental Biology, 41(5): 1026-1033. http://doi.org/10.22438/jeb/41/5/MRN-1097 [ Links ]
Gindro, K, Schnee, S, Lecoultre, N, Michellod, E, Zufferey, V, Spring, JL, Viret, O y Dubuis, PH (2022). Development of downy mildew in grape bunches of susceptible and resistant cultivars: infection pathways and limited systemic spread. Australian Journal of Grape and Wine Research, 28(4): 572-580. https://doi.org/10.1111/ajgw.12560 [ Links ]
Giovinazzo, G y Grieco, F (2015). Functional properties of grape and wine polyphenols. Plant Foods for Human Nutrition, 70(4): 454-462. https://doi.org/10.1007/s11130-015-0518-1 [ Links ]
Gisi, U y Sierotzki, H (2008). Fungicide modes of action and resistance in downy mildews. European Journal of Plant Pathology, 122: 157-167. https://doi.org/10.1007/s10658-008-9290-5 [ Links ]
Gisi, U y Sierotzki, H (2015). Oomycete Fungicides: Phenylamides, Quinone Outside Inhibitors, and Carboxylic Acid Amides. En: Ishii, H y Hollomon, D (eds.). Fungicide Resistance in Plant Pathogens. Tokyo, Japón: Springer, 145-174. https://doi.org/10.1007/978-4-431-55642-8_10 [ Links ]
Gisi, U (1996). Synergistic interaction of fungicides in mixtures. Phytopathology, 86(11): 1273-1279. [ Links ]
Göker, M, Voglmayr, H, Riethmüller, A y Oberwinkler, F (2007). How do obligate parasites evolve? A multi-gene phylogenetic analysis of downy mildews. Fungal Genetics and Biology, 44(2): 105-122. https://doi.org/10.1016/j.fgb.2006.07.005 [ Links ]
Guerreiro, A, Figueiredo, J, Sousa Silva, M y Figueiredo, A (2016). Linking jasmonic acid to grapevine resistance against the biotrophic oomycete Plasmopara viticola. Frontiers in Plant Science, 7: 565. https://doi.org/10.3389/fpls.2016.00565 [ Links ]
Hall, BH, McKay, SF, Lopez, F, Harper, L, Savocchia, S, Borneman, A y Herderich, M (2017). Fungicide resistance in Australian viticulture. Modern Fungicides and Antifungal Compounds, 8: 181-186. [ Links ]
Hamiduzzaman, MM, Jakab, G, Barnavon, L, Neuhaus, JM y Mauch-Mani, B (2005). β-aminobutyric acid-induced resistance against downy mildew in grapevine acts through the potentiation of callose formation and jasmonic acid signaling. Molecular Plant-Microbe Interactions, 18(8): 819-829. https://doi.org/10.1094/MPMI-18-0819 [ Links ]
Hannah, L, Roehrdanz, PR, Ikegami, M, Shepard, AV, Shaw, MR, Tabor, G, Zhi, L, Marquet, PA y Hijmans, RJ (2013). Climate change, wine, and conservation. Proceedings of the National Academy of Sciences, 110(17): 6907-6912. https://doi.org/10.1073/pnas.1210127110 [ Links ]
Harm, A, Kassemeyer, HH, Seibicke, T y Regner, F (2011). Evaluation of chemical and natural resistance inducers against downy mildew (Plasmopara viticola) in grapevine. American Journal of Enology and Viticulture, 62(2): 184-192. https://doi.org/10.5344/ajev.2011.09054 [ Links ]
Hickey, CC, Kwasniewski, MT y Wolf, TK (2018). Leaf removal effects on Cabernet franc and Petit Verdot: II. Grape carotenoids, phenolics, and wine sensory analysis. American Journal of Enology and Viticulture, 69(3): 231-246. https://doi.org/10.5344/ajev.2018.17107 [ Links ]
Hollomon, DW (2015). Fungicide resistance: facing the challenge. Plant Protection Science, 51(4): 170-176. https://doi.org/10.17221/42/2015-PPS [ Links ]
Inchaya, P, Mathukorn, S, Sopone, W, Dusit, A y Natthiya, B (2013). Changes in salicylic acid in grapevine treated with chitosan and BTH against Sphaceloma ampelinum, the causal agent of grapevine anthracnose. African Journal of Microbiology Research, 7(7): 557-563. [ Links ]
Index Fungorum (2023). Index Fungorum Database. http://www.indexfungorum.org/names/NamesRecord.asp?RecordID=208592 [ Links ]
Instituto Nacional de Estadística y Censo (2021). Encuesta Nacional Agropecuaria 2021. Resultados generales de la actividad agrícola y forestal. https://inec.cr/estadisticas-fuentes/encuestas/encuesta-nacional-agropecuaria [ Links ]
Jackson, RS (2022). Wine Science. Principles and Applications. Londres, UK: Academic Press. 1030p. [ Links ]
Jacquens, L, Trouvelot, S, Lemaitre-Guillier, C, Krzyzaniak, Y, Clément, G, Citerne, S, Mouille, G, Moreau, E, Héloir, M-C y Adrian, M (2022). Biostimulation can prime elicitor induced resistance of grapevine leaves to downy mildew. Frontiers in Plant Science, 13: 998273. https://doi.org/10.3389/fpls.2022.998273 [ Links ]
Jermini, M, Blaise, P y Gessler, C (2010). Quantitative effect of leaf damage caused by downy mildew (Plasmopara viticola) on growth and yield quality of grapevine ‘Merlot’ (Vitis vinifera). Vitis, 49(2): 77-85. [ Links ]
Kamble, MV, Joshi, SM, Hadimani, S y Jogaiah, S (2021). Biopriming with rhizosphere Trichoderma harzianum elicit protection against grapevine downy mildew disease by triggering histopathological and biochemical defense responses. Rhizosphere, 19: 100398. https://doi.org/10.1016/j.rhisph.2021.100398 [ Links ]
Kassemeyer, HH, Gaduroy, DM, Hill, G y Wilcox, WF (2015). Part I. Diseases caused by biotic factors: diseases caused by fungi and oomicetes. En: Wilcox, WF, Gubler, WD y Uyemoto, JK (eds.). Compendium of grape diseases, disorders, and pests. APS Press, MN, USA. 17-146. [ Links ]
Khan, W, Rayirath, UP, Subramanian, S, Jithesh, MN, Rayorath, P, Hodges, DM, Critchley, AT, Craigie, JS, Norrie, J y Prithiviraj, B (2009). Seaweed extracts as biostimulants of plant growth and development. Journal of Plant Growth Regulation, 28: 386-399. https://doi.org/10.1007/s00344-009-9103-x [ Links ]
Kennelly, MM, Gadoury, DM, Wilcox, WF, Magarey, PA y Seem, RC (2007). Primary infection, lesion productivity, and survival of sporangia in the grapevine downy mildew pathogen Plasmopara viticola. Phytopathology, 97(4): 512-522. https://doi.org/10.1094/PHYTO-97-4-0512 [ Links ]
Khalil-Ur-Rehman, M, Wang, W, Xu, YS, Haider, MS, Li, CX y Tao, JM (2017). Comparative study on reagents involved in grape bud break and their effects on different metabolites and related gene expression during winter. Frontiers in Plant Science, 8: 1340. https://doi.org/10.3389/fpls.2017.01340 [ Links ]
Koledenkova, K, Esmaeel, Q, Jacquard, C, Nowak, J, Clément, C y Barka, EA (2022). Plasmopara viticola, the causal agent of downy mildew of grapevine: from its taxonomy to disease management. Frontiers in Microbiology. https://doi.org/10.3389/fmicb.2022.889472 [ Links ]
Kortekamp, A (1997). Epicoccum nigrum LINK: A biological control agent of Plasmopara viticola (BERK. et CURT.). Vitis, 36(4): 215-216. [ Links ]
Kortekamp, A (2005). Growth, occurrence and development of septa in Plasmopara viticola and other members of the Peronosporaceae using light-and epifluorescence-microscopy. Mycological Research, 109(5): 640-648. https://doi.org/10.1017/S0953756205002418 [ Links ]
Küpper, V, Steiner, U y Kortekamp, A (2022). Trichoderma species isolated from grapevine with tolerance towards common copper fungicides used in viticulture for plant protection. Pest Management Science, 78(8): 3266-3276. https://doi.org/10.1002/ps.6951 [ Links ]
Lakkis, S, Trotel-Aziz, P, Rabenoelina, F, Schwarzenberg, A, Nguema-Ona, E, Clément, C y Aziz, A (2019). Strengthening grapevine resistance by Pseudomonas fluorescens PTA-CT2 relies on distinct defense pathways in susceptible and partially resistant genotypes to downy mildew and gray mold diseases. Frontiers in Plant Science, 10: 1112. https://doi.org/10.3389/fpls.2019.01112 [ Links ]
Lallanilla, M. (2013). Will global warming crush the wine industry?. Live Science. https://www.livescience.com/28577-wine-global-warming.html [ Links ]
Lamberth, C (2019). Episodes from the continuous search for solutions against downy mildew diseases. Chimia, 73(7-8): 571-571. https://doi.org/10.2533/chimia.2019.571 [ Links ]
Lamichhane, JR, Osdaghi, E, Behlau, F, Köhl, J, Jones, JB y Aubertot, JN (2018). Thirteen decades of antimicrobial copper compounds applied in agriculture. A review. Agronomy for Sustainable Development, 38: 1-18. https://doi.org/10.1007/s13593-018-0503-9 [ Links ]
Leão, PCDS, Nunes, BTG y Lima, MACD (2016). Canopy management effects on ‘Syrah’ grapevines under tropical semi-arid conditions. Scientia Agricola, 73: 209-216. https://doi.org/10.1590/0103-9016-2014-0408 [ Links ]
Leis, D, Renner, W y Leitner, E (2018). Characterisation of wines produced from fungus resistant grape varieties. En: Flavour Science. Proceedings of the XV Weurman Flavour Research Symposium, Graz University of Technology, Austria. https://doi.org/10.3217/978-3-85125-593-5-109 [ Links ]
Liang, C, Zang, C, McDermott, MI, Zhao, K, Yu, S y Huang, Y (2016). Two imide substances from a soil-isolated Streptomyces atratus strain provide effective biocontrol activity against grapevine downy mildew. Biocontrol Science and Technology, 26(10): 1337-1351. https://doi.org/10.1080/09583157.2016.1199014 [ Links ]
Liang, Z, Cheng, L, Zhong, GY y Liu, RH (2014). Antioxidant and antiproliferative activities of twenty-four Vitis vinifera grapes. PLoS ONE, 9(8). https://doi.org/10.1371/journal.pone.0105146 [ Links ]
Lin, H, Leng, H, Guo, Y, Kondo, S, Zhao, Y, Shi, G y Guo, X (2019). QTLs and candidate genes for downy mildew resistance conferred by interspecific grape (V. vinifera L. × V. amurensis Rupr.) crossing. Scientia Horticulturae, 244: 200-207. https://doi.org/10.1016/j.scienta.2018.09.045 [ Links ]
Lizano-Sáenz, JR (1992). Carta de entendimiento. Programa Cooperativo entre el Ministerio de Agricultura y Ganadería, Instituto Nacional de Aprendizaje, Instituto de Desarrollo Agrario y la Misión Técnica Agrícola de la República de China, para la ejecución del proyecto de uva en Costa Rica. https://www.mag.go.cr/convenios/1992/1992c07-0434.pdf [ Links ]
Lucas, JA, Hawkins, NJ y Fraaije, BA (2015). The evolution of fungicide resistance. Advances in Applied Microbiology, 90: 29-92. https://doi.org/10.1016/bs.aambs.2014.09.001 [ Links ]
Mackenzie, DE y Christy, AG (2005). The role of soil chemistry in wine grape quality and sustainable soil management in vineyards. Water Science and Technology, 51(1): 27-37. https://doi.org/10.2166/wst.2005.0004 [ Links ]
Maddalena, G, Russo, G y Toffolatti, SL (2021). The study of the germination dynamics of Plasmopara viticola oospores highlights the presence of phenotypic synchrony with the host. Frontiers in Microbiology, 12: 698586. https://doi.org/10.3389/fmicb.2021.698586 [ Links ]
Madden, LV, Ellis, MA, Lalancette, N, Hughes, G y Wilson, LL (2000). Evaluation of a disease warning system for downy mildew of grapes. Plant Disease, 84(5): 549-554. https://doi.org/10.1094/PDIS.2000.84.5.549 [ Links ]
Markellou, E, Kapaxidi, E, Karamaouna, F, Samara, M, Kyriakopoulou, K, Anastasiadou, P, Vavoulidou, E, Meidanis, M, Machera, K, Mandoulaki, A, Margaritopoulou, T, Giovannini, O, Tomada, S y Pertot, I (2022). Evaluation of plant protection efficacy in field conditions and side effects of Lysobacter capsici AZ78, a biocontrol agent of Plasmopara viticola. Biocontrol Science and Technology, 32(8): 930-951. https://doi.org/10.1080/09583157.2022.2064431 [ Links ]
Massi, F, Marcianò, D, Russo, G, Stuknytė, M, Arioli, S, Mora, D y Toffolatti, SL (2022). Evaluation of the characteristics and infectivity of the secondary inoculum produced by Plasmopara viticola on grapevine leaves by means of flow cytometry and fluorescence-activated cell sorting. Applied and Environmental Microbiology, 88(21): e01010-22. https://doi.org/10.1128/aem.01010-22 [ Links ]
Massi, F, Torriani, SF, Borghi, L y Toffolatti, SL (2021). Fungicide resistance evolution and detection in plant pathogens: Plasmopara viticola as a case study. Microorganisms, 9(1): 119. https://doi.org/10.3390/microorganisms9010119 [ Links ]
Matasci, CL, Gobbin, D, Schärer, HJ, Tamm, L y Gessler, C (2008). Selection for fungicide resistance throughout a growing season in populations of Plasmopara viticola. European Journal of Plant Pathology, 120: 79-83. https://doi.org/10.1007/s10658-007-9190-0 [ Links ]
Merdinoglu, D, Schneider, C, Prado, E, Wiedemann-Merdinoglu, S y Mestre, P (2018). Breeding for durable resistance to downy and powdery mildew in grapevine. OENO One, 52(3): 203-209. https://doi.org/10.20870/oeno-one.2018.52.3.2116 [ Links ]
Mian, G, Buso, E y Tonon, M (2021). Decision support systems for downy mildew (Plasmopara viticola) control in grapevine: short comparison review. Asian Research Journal of Agriculture, 14(2): 12-20. https://doi.org/10.9734/arja/2021/v14i230120 [ Links ]
Mian, G, Musetti, R, Belfiore, N, Boscaro, D, Lovat, L y Tomasi, D (2023). Chitosan application reduces downy mildew severity on grapevine leaves by positively affecting gene expression pattern. Physiological and Molecular Plant Pathology, 125: 102025. https://doi.org/10.1016/j.pmpp.2023.102025 [ Links ]
Moreira, FM, Madini, A, Marino, R, Zulini, L, Stefanini, M, Velasco, R, Kozma, P y Grando, MS (2011). Genetic linkage maps of two interspecific grape crosses (Vitis spp.) used to localize quantitative trait loci for downy mildew resistance. Tree Genetics & Genomes, 7: 153-167. https://doi.org/10.1007/s11295-010-0322-x [ Links ]
Moriondo, M, Jones, GV, Bois, B, Dibari, C, Ferrise, R, Trombi, G y Bindi, M (2013). Projected shifts of wine regions in response to climate change. Climatic Change, 119(3): 825-839. https://doi.org/10.1007/s10584-013-0739-y [ Links ]
Mosedale, JR, Abernethy, KE, Smart, RE, Wilson, RJ y Maclean, IMD (2016). Climate change impacts and adaptive strategies: lessons from the grapevine. Global Change Biology, 22(11): 3814-3828. https://doi.org/10.1111/gcb.13406 [ Links ]
Mozell, MR y Thach, L (2014). The impact of climate change on the global wine industry: challenges and solutions. Wine Economics and Policy, 3: 81-89. https://doi.org/10.1016/j.wep.2014.08.001 [ Links ]
Mundt, CC (2014). Durable resistance: a key to sustainable management of pathogens and pests. Infection, Genetics and Evolution, 27: 446-455. https://doi.org/10.1016/j.meegid.2014.01.011 [ Links ]
Musetti, R, Vecchione, A, Stringher, L, Borselli, S, Zulini, L, Marzani, C, D’Ambrosio, L, di Toppi, S y Pertot, I (2006). Inhibition of sporulation and ultrastructural alterations of grapevine downy mildew by the endophytic fungus Alternaria alternata. Phytopathology, 96(7): 689-698. https://doi.org/10.1094/PHYTO-96-0689 [ Links ]
Muthmann, R y Nadin, P (2007). The use of plant protection products in the European Union. Eurostat Publications. https://ec.europa.eu/eurostat/documents/3217494/5611788/KS-76-06-669-EN.PDF.pdf [ Links ]
Nascimento-Gavioli, MCA, Rockenbach, MF, Welter, LJ y Guerra, MP (2020). Histopathological study of resistant (Vitis labrusca L.) and susceptible (Vitis vinifera L.) cultivars of grapevine to the infection by downy mildew. The Journal of Horticultural Science and Biotechnology, 95(4): 521-531. https://doi.org/10.1080/14620316.2019.1685411 [ Links ]
Nassur, RDCMR, Pereira, GE, Glória, MBA y de Oliveira Lima, LC (2017). Rootstock influencing the quality and biogenic amines content on Syrah tropical wines. Comunicata Scientiae, 8(2): 202-208. https://doi.org/10.14295/CS.v8i2.2562 [ Links ]
Organización Internacional de la Viña y el Vino (2017). Distribution of the world’s grapevine varieties. Focus OIV. https://www.oiv.int/public/medias/5888/en-distribution-of-the-worlds-grapevine-varieties.pdf [ Links ]
Organización Internacional de la Viña y el Vino (2019). Statistical report on world vitiviniculture. http://www.oiv.int/public/medias/6782/oiv-2019-statistical-report-on-world-vitiviniculture.pdf [ Links ]
Palmieri, MC, Perazzolli, M, Matafora, V, Moretto, M, Bachi, A y Pertot, I (2012). Proteomic analysis of grapevine resistance induced by Trichoderma harzianum T39 reveals specific defence pathways activated against downy mildew. Journal of Experimental Botany, 63: 6237-6251. https://doi.org/10.1093/jxb/ers279 [ Links ]
Paris, F, Krzyżaniak, Y, Gauvrit, C, Jamois, F, Domergue, F, Joubès, J, Ferrières, V, Adrian, M, Legentil, L, Daire, X y Trouvelot, S (2016). An ethoxylated surfactant enhances the penetration of the sulfated laminarin through leaf cuticle and stomata, leading to increased induced resistance against grapevine downy mildew. Physiologia Plantarum, 156(3): 338-350. https://doi.org/10.1111/ppl.12394 [ Links ]
Perazzolli, M, Dagostin, S, Ferrari, A, Elad, Y y Pertot, I (2008). Induction of systemic resistance against Plasmopara viticola in grapevine by Trichoderma harzianum T39 and benzothiadiazole. Biological Control, 47(2): 228-234. https://doi.org/10.1016/j.biocontrol.2008.08.008 [ Links ]
Pertot, I, Caffi, T, Rossi, V, Mugnai, L, Hoffmann, C, Grando, MS, Gary, C, Lafond, D, Duso, C, Thiery, D, Mazzoni, V y Anfora, G (2017). A critical review of plant protection tools for reducing pesticide use on grapevine and new perspectives for the implementation of IPM in viticulture. Crop Protection, 97: 70-84. https://doi.org/10.1016/j.cropro.2016.11.025 [ Links ]
Poeydebat, C, Courchinoux, E, Delière, L, Raynal, M y Delmotte, F (2022). Quantification and management of Plasmopara viticola primary inoculum in soil-Towards prophylactic control of grapevine downy mildew. BIO Web of Conferences, 50: 03011. https://doi.org/10.1051/bioconf/20225003011 [ Links ]
Possamai, T y Wiedemann-Merdinoglu, S (2022). Phenotyping for QTL identification: A case study of resistance to Plasmopara viticola and Erysiphe necator in grapevine. Frontiers in Plant Science, 13: 930954. https://doi.org/10.3389/fpls.2022.930954 [ Links ]
Pozzebon, A y Duso, C (2008). Grape downy mildew Plasmopara viticola, an alternative food for generalist predatory mites occurring in vineyards. Biological Control, 45(3): 441-449. https://doi.org/10.1016/j.biocontrol.2008.02.001 [ Links ]
Puopolo, G, Giovannini, O y Pertot, I (2014). Lysobacter capsici AZ78 can be combined with copper to effectively control Plasmopara viticola on grapevine. Microbiological Research, 169(7-8): 633-642. https://doi.org/10.1016/j.micres.2013.09.013 [ Links ]
Pymes (2015). Conozca al embajador de las uvas en Costa Rica. El Financiero. https://www.elfinancierocr.com/pymes/conozca-al-embajador-de-las-uvas-en-costa-rica/WNOJNZAJNNENVBSOM5NC5CZZ6M/story/ [ Links ]
Reuveni, M, Zahavi, T y Cohen, Y (2001). Controlling downy mildew (Plasmopara viticola) in field-grown grapevine with β-aminobutyric acid (BABA). Phytoparasitica, 29: 125-133. https://doi.org/10.1007/BF02983956 [ Links ]
Romanazzi, G, Mancini, V, Feliziani, E, Servili, A, Endeshaw, S y Neri, D (2016). Impact of alternative fungicides on grape downy mildew control and vine growth and development. Plant Disease, 100(4): 739-748. https://doi.org/10.1094/PDIS-05-15-0564-RE [ Links ]
Romanazzi, G, Mancini, V, Foglia, R, Marcolini, D, Kavari, M y Piancatelli, S (2021). Use of chitosan and other natural compounds alone or in different strategies with copper hydroxide for control of grapevine downy mildew. Plant Disease, 105(10): 3261-3268. https://doi.org/10.1094/PDIS-06-20-1268-RE [ Links ]
Rossi, V, Caffi, T y Gobbin, D (2013). Contribution of molecular studies to botanical epidemiology and disease modelling: Grapevine downy mildew as a case-study. European Journal of Plant Pathology. https://doi.org/10.1007/s10658-012-0114-2 [ Links ]
Rossi, V, Caffi, T y Salinari, F (2008). Development and evaluation of a dynamic risk model for downy mildew of grapevine. Agricultural and Forest Meteorology, 148(8): 1383-1394. https://doi.org/10.1016/j.agrformet.2008.04.014 [ Links ]
Rossi, V, Giosuè, S, Caffi, T, Rossi, V y Bugiani, R (2008). A mechanistic model simulating primary infections of downy mildew in grapevine. Ecological Modelling, 212(3): 480-491. https://doi.org/10.1016/j.ecolmodel.2007.10.041 [ Links ]
Saenz, L, Peña, I, Marín, D y Arias, J (2015). Diversificación de las plantaciones de uva en Costa Rica. Agronomía Costarricense, 40(1): 23-35. [ Links ]
Salinari, F, Giosuè, S, Tubiello, FN, Rosenzweig, C, Bindi, M y Rossi, V (2007). Downy mildew (Plasmopara viticola) epidemics on grapevine under climate change. Global Change Biology, 13(6): 1299-1307. https://doi.org/10.1111/j.1365-2486.2007.01348.x [ Links ]
Salinari, F, Giosuè, S, Tubiello, FN, Rosenzweig, C, Bindi, M y Rossi, V (2006). Modelling the impact of climate change on downy mildew in grapevine at a regional scale. Proceedings of the International Symposium on Climate Change, 1: 229-241. [ Links ]
Sargolzaei, M, Rustioni, L, Cola, G, Ricciardi, V, Bianco, PA, Maghradze, D, Failla, O, Quaglino, F y Toffolatti, SL (2021). Georgian grapevine cultivars: ancient biodiversity for future viticulture. Frontiers in Plant Science, 12: 94. https://doi.org/10.3389/fpls.2021.630122 [ Links ]
Sarkhosh-Khorasani, S, Sangsefidi, ZS y Hosseinzadeh, M (2021). The effect of grape products containing polyphenols on oxidative stress: a systematic review and meta-analysis of randomized clinical trials. Nutrition Journal, 20(1): 1-18. https://doi.org/10.1186/s12937-021-00686-5 [ Links ]
Servicio Fitosanitario de Estado (2023). Sistema de Insumos y Fiscalización. Ministerio de Agricultura y Ganadería. http://app.sfe.go.cr/SFEInsumos/aspx/Seguridad/Home.aspx [ Links ]
Shen, H, Li, Z, Yang, J, Zhang, M y Ran, L (2017). Identification of the mycoparasitic strain F3 on Plasmopara viticola and its control effect on grape downy mildew. Journal of Plant Protection, 44(4): 643-649. [ Links ]
Sheng-Pin, L (1988). Los viñedos en Costa Rica. Boltec, 21(3): 32-39. [ Links ]
Slaughter, AR, Hamiduzzaman, MM, Gindro, K, Neuhaus, JM y Mauch-Mani, B (2008). Beta-aminobutyric acid-induced resistance in grapevine against downy mildew: involvement of pterostilbene. En: Lebeda, A y Spencer-Phillips, PTN (eds.). The Downy Mildews—Genetics, Molecular Biology and Control. Springer, Dordrecht, 206. https://doi.org/10.1007/978-1-4020-8973-2_14 [ Links ]
Soares, S, Brandão, E, Mateus, N y de Freitas, V (2017). Sensorial properties of red wine polyphenols: Astringency and bitterness. Critical Reviews in Food Science and Nutrition, 57(5): 937-948. https://doi.org/10.1080/10408398.2014.946468 [ Links ]
Steimetz, E, Trouvelot, S, Gindro, K, Bordier, A, Poinssot, B, Adrian, M y Daire, X (2012). Influence of leaf age on induced resistance in grapevine against Plasmopara viticola. Physiological and Molecular Plant Pathology, 79: 89-96. https://doi.org/10.1016/j.pmpp.2012.05.004 [ Links ]
Sun, Q, Gates, MJ, Lavin, EH, Acree, TE y Sacks, GL (2011). Comparison of odor-active compounds in grapes and wines from Vitis vinifera and non-foxy American grape species. Journal of Agricultural and Food Chemistry, 59(19): 10657-10664. https://doi.org/10.1021/jf2026204 [ Links ]
Taibi, O, Bardelloni, V, Bove, F, Scaglia, F, Caffi, T y Rossi, V (2022). Activity of resistance inducers against Plasmopara viticola in vineyard. En: 9th International Workshop on Grapevine Downy and Powdery Mildews (GDPM 2022), BIO Web of Conferences, vol. 50. https://doi.org/10.1051/bioconf/20225003003 [ Links ]
Taibi, O, Salotti, I y Rossi, V (2023). Plant resistance inducers affect multiple epidemiological components of Plasmopara vitícola on grapevine leaves. Plants, 12(16): 2938. https://doi.org/10.3390/plants12162938 [ Links ]
Taylor, AS y Cook, DC (2018). An economic assessment of the impact on the Western Australian viticulture industry from the incursion of grapevine downy mildew. Journal of Plant Diseases and Protection, 125(4): 397-403. https://doi.org/10.1007/s41348-018-0152-x [ Links ]
Taylor, AS (2021). Downy mildew of grapevines. Department of Primary Industries and Regional Development: Agriculture and Food. https://www.agric.wa.gov.au/table-grapes/downy-mildew-grapevines?page=0%2C1 [ Links ]
Teissedre, PL (2018). Composition of grape and wine from resistant vines varieties. Oeno One, 52(3): 211-217. https://doi.org/10.20870/oeno-one.2018.52.3.2223 [ Links ]
Thind, TS, Arora, JK, Mohan, C y Raj, P (2004). Epidemiology of powdery mildew, downy mildew and anthracnose diseases of grapevine. En: Naqvi, SAMH (ed.). Diseases of Fruits and Vegetables Volume I. Springer, Dordrecht, 621-638. https://doi.org/10.1007/1-4020-2606-4_14 [ Links ]
This, P, Lacombe, T y Thomas, MR (2006). Historical origins and genetic diversity of wine grapes. Trends in Genetics, 22(9): 511-519. https://doi.org/10.1016/j.tig.2006.07.008 [ Links ]
Toffolatti, SL, Prandato, M, Serrati, L, Sierotzki, H, Gisi, U y Vercesi, A (2011). Evolution of Qol resistance in Plasmopara viticola oospores. European Journal of Plant Pathology, 129: 331-338. https://doi.org/10.1007/s10658-010-9677-y [ Links ]
Toffolatti, SL, Maddalena, G, Salomoni, D, Maghradze, D, Bianco, PA y Failla, O (2016). Evidence of resistance to the downy mildew agent Plasmopara viticola in the Georgian Vitis vinifera germplasm. Vitis, 55(3): 121-128. https://doi.org/10.5073/vitis.2016.55.121-128 [ Links ]
Toffolatti, SL, de Lorenzis, G, Costa, A, Maddalena, G, Passera, A, Bonza, MC, Pindo, M, Stefani, E, Cestaro, A, Casati, P, Failla, O, Bianco, PA, Maghradze, D y Quaglino, F (2018). Unique resistance traits against downy mildew from the center of origin of grapevine (Vitis vinifera). Scientific Reports, 8(1): 1-11. https://doi.org/10.1038/s41598-018-30413-w [ Links ]
Toffolatti, SL, Russo, G, Campia, P, Bianco, PA, Borsa, P, Coatti, M, Torriani, SFF y Sierotzki, H (2018). A time‐course investigation of resistance to the carboxylic acid amide mandipropamid in field populations of Plasmopara viticola treated with anti‐resistance strategies. Pest Management Science, 74(12): 2822-2834. https://doi.org/10.1002/ps.5072 [ Links ]
Tonietto, J y Pereira, GE (2011). The development of the viticulture for a high quality tropical wine production in the world. 17th GiESCO Meeting. https://ainfo.cnptia.embrapa.br/digital/bitstream/item/131044/1/45754.pdf [ Links ]
Trouvelot, S, Varnier, AL, Allègre, M, Mercier, L, Baillieul, F, Arnould, C, Gianinazzi-Pearson, V, Klarzynski, O, Joubert, JM, Pugin, A y Daire, X (2008). A β-1, 3 glucan sulfate induces resistance in grapevine against Plasmopara viticola through priming of defense responses, including HR-like cell death. Molecular Plant-Microbe Interactions, 21(2): 232-243. https://doi.org/10.1094/MPMI-21-2-0232 [ Links ]
Tylianakis, JM, Didham, RK, Bascompte, J y Wardle, DA (2008). Global change and species interactions in terrestrial ecosystems. Ecology Letters, 11(12): 1351-1363. https://doi.org/10.1111/j.1461-0248.2008.01250.x [ Links ]
van den Bosch, F, Paveley, N, van den Berg, F, Hobbelen, P y Oliver, R (2014). Mixtures as a fungicide resistance management tactic. Phytopathology, 104(12): 1264-1273. https://doi.org/10.1094/PHYTO-04-14-0121-RVW [ Links ]
Vercesi, A, Toffolatti, SL, Zocchi, G, Guglielmann, R y Ironi, L (2010). A new approach to modelling the dynamics of oospore germination in Plasmopara viticola. European Journal of Plant Pathology, 128: 113-126. https://doi.org/10.1007/s10658-010-9635-8 [ Links ]
Vitalini, S, Orlando, F y Iriti, M (2020). Field study on the efficacy of plant activators against Plasmopara viticola. Plant Fungal Research, 3(2): 2-7. http://dx.doi.org/10.29228/plantfungalres.71 [ Links ]
Wicks, TJ, Hall, BH y Somers, A (2005). First report of metalaxyl resistance of grapevine downy mildew in Australia. En: The 15th Biennial Australasian Plant Pathology Society Conference Handbook. Australasian Plant Pathology Society, Geelong, Australia. [ Links ]
Wilcox, WF, Gubler, WD y Uyemoto, JK (2015). Compendium of grape diseases, disorders, and pests. APS Press, Second Edition. MN, USA, 232p. [ Links ]
Wingerter, C, Eisenmann, B, Weber, P, Dry, I y Bogs, J (2021). Grapevine Rpv3-, Rpv10- and Rpv12-mediated defense responses against Plasmopara viticola and the impact of their deployment on fungicide use in viticulture. BMC Plant Biology, 21(1): 1-17. https://doi.org/10.1186/s12870-021-03228-7 [ Links ]
Wurz, DA, de Bem, BP, Allebrandt, R, Bonin, B, Dalmolin, LG, Canossa, AT, Rufato, L y Kretzschmar, AA (2017). New wine-growing regions of Brazil and their importance in the evolution of Brazilian wine. En: BIO Web of Conferences, vol. 9. EDP Sciences. https://doi.org/10.1051/bioconf/20170901025 [ Links ]
Yin, L, An, Y, Qu, J, Li, X, Zhang, Y, Dry, I, Wu, H y Lu, J (2017). Genome sequence of Plasmopara viticola and insight into the pathogenic mechanism. Scientific Reports, 7: 1-12. https://doi.org/10.1038/srep46553 [ Links ]
Yu, Y, Zhang, Y, Yin, L y Lu, J (2012). The mode of host resistance to Plasmopara viticola infection of grapevines. Phytopathology, 102(11): 1094-1101. https://doi.org/10.1094/PHYTO-02-12-0028-R [ Links ]
Yu, S, Li, B, Guan, T, Liu, L, Wang, H, Liu, C, Zang, C, Huang, Y y Liang, C (2022). A comparison of three types of “vineyard management” and their effects on the structure of Plasmopara viticola populations and epidemic dynamics of grape downy mildew. Plants, 11(16): 2175. https://doi.org/10.3390/plants11162175 [ Links ]
Zang, C, Lin, Q, Xie, J, Lin, Y, Zhao, K y Liang, C (2020). The biological control of the grapevine downy mildew disease using Ochrobactrum sp. Plant Protection Science, 56: 52-61. https://doi.org/10.17221/87/2019-PPS [ Links ]
Zeng, Q, Xie, J, Li, Y, Gao, T, Zhang, X y Wang, Q (2021). Comprehensive Genomic Analysis of the endophytic Bacillus altitudinis strain GLB197, a potential biocontrol agent of grape downy mildew. Frontiers in Genetics, 12: 729603. https://doi.org/10.3389/fgene.2021.729603 [ Links ]
Zhang, H, Kong, F, Wang, X, Liang, L, Schoen, CD, Feng, J y Wang, Z (2017). Tetra-primer ARMS PCR for rapid detection and characterization of Plasmopara viticola phenotypes resistant to carboxylic acid amide fungicides. Pest Management Science, 73: 1655-1660. https://doi.org/10.1002/ps.4506 [ Links ]
Zhang, X, Zhou, Y, Li, Y, Fu, X y Wang, Q (2017). Screening and characterization of endophytic Bacillus for biocontrol of grapevine downy mildew. Crop Protection, 96: 173-179. https://doi.org/10.1016/j.cropro.2017.02.018 [ Links ]
Zini, E, Dolzani, C, Stefanini, M, Gratl, V, Bettinelli, P, Nicolini, D, Betta, G, Dorigatti, C, Velasco, R, Letschka, T y Vezzulli, S (2019). R-loci arrangement versus downy and powdery mildew resistance level: a Vitis hybrid survey. International Journal of Molecular Sciences, 20(14): 1-29. https://doi.org/10.3390/ijms20143526 [ Links ]
Zyprian, E, Ochßner, I, Schwander, F, Šimon, S, Hausmann, L, Bonow-Rex, M, Moreno-Sanz, P, Grando, MS, Wiedemann-Merdinoglu, S, Merdinolgu, D, Eibach, R y Töpfer, R (2016). Quantitative trait loci affecting pathogen resistance and ripening of grapevines. Molecular Genetics and Genomics, 291: 1573-1594. https://doi.org/10.1007/s00438-016-1200-5 [ Links ]
Received: September 18, 2023; Accepted: December 10, 2023